1 Introduction
Since the prototype of a dye-sensitized solar cell (DSSC) was reported in 1991 by O’Regan and Grätzel [1], considerable efforts have been devoted to it [1–2]. Based on liquid electrolytes, DSSC with energy conversion efficiency of 11% has been achieved [3–4]. However, the potential problems caused by liquid electrolytes, such as the leakage and volatilization of organic solvents, are considered as some of the critical factors limiting the long-term performance and practical use of DSSC. Thus, researchers have been paying attention to find the substitutes of liquid electrolytes with some kinds of higher stable electrolytes such as polymer gel electrolytes, organic/inorganic p-type semiconductors, polymer electrolytes and so on [5–7]. Among them, polymer gel electrolytes are considered as one of the most prospective substitutes for liquid electrolytes. They usually exist in quasi-solid-state, which is on the boundary between liquid-state and solid-state, so they have both merits of solid-state electrolytes such as higher long-term stability, lower leakage or volatilization, and have advantages of liquid electrolytes as highly ionic conductivity, excellent interfacial contacting property [8–9]. These characteristics are beneficial for fabricating highly photovoltaic performance and long-term stable quasi-solid-state DSSC (QS-DSSC). In continuous efforts, QS-DSSC with energy conversion efficiency over 7% has been achieved [10].
The polymer gel electrolyte usually consists of a polymer gelling agent, a solvent (or mixed solvents) and an ionic conductor [11]. The concentration of ionic conductors dissolved in the solvent influences the ionic conductivity of the polymer gel electrolyte and the networks of polymer gelling agent formed in the polymer gel electrolyte affects the long-term stability of the polymer gel electrolyte. The high amount of absorbed solvent with high concentration of ionic conductors in the polymer gel electrolyte are needed to obtain high ionic conductivity and good photovoltaic performance of QS-DSSC, while the problem is that the solvent with high concentration of ionic conductors is hardly solidified by the polymer gelling agent; on the other hand, it will cause the shrinkage or phase separation of polymer gelling agent from the polymer gel electrolyte, which limits the ionic conductivity of the polymer gel electrolyte. If ionic conductors can be synthesized inside the polymer gel electrolyte in situ rather than be absorbed into the polymer gel electrolyte with solvent, the above-mentioned contradiction may be solved. Here, we try to fabricate a polymer gel electrolyte with in situ synthesized Acac-Py-I2 ionic conductors in order to solve the above-mentioned problems usually existing in polymer gel electrolytes [8,12–13]. It is found that the method of in situ synthesized ionic conductors in the polymer gel electrolyte can maintain high liquid electrolyte absorbency and highly ionic conductivity, QS-DSSC with the polymer gel electrolyte also shows improved photovoltaic performance.
2 Experimental
2.1 Materials
PEG with average molecular weight 20,000, acrylic acid monomers, ammonium persulfate, sodium pyrosulfite, titanium iso-propoxide, nitric acid, glacial acetic acid, terpineol, ethyl cellulose, ethanol, γ-butyrolactone (GBL), N-methylpyrrolidone (NMP), pyridine (PY), acetylacetone (Acac) and iodine (I2) were all A. R. grade and purchased from Sinopharm Chemical Reagent Co., Ltd, China. All reagents were used without further treatment.
Conducting glass plates (FTO glass, Fluorine doped tin oxide over-layer, sheet resistance 8 Ω·−2, purchased from Hartford Glass Co., USA) were used as substrates for precipitating TiO2 porous films. Sensitizing dye cis-[(dcbH2)2Ru(SCN)2] and optical diffuser paste Ti-nanoxide 300 were purchased from Solaronix SA.
2.2 Preparation of thermosetting polymer gel electrolyte with in situ synthesized ionic conductors
The PAA-PEG matrix was synthesized as given in the references [8,13–14]. The polymer gel electrolytes prepared with in situ synthesized Acac-Py-I2 ionic conductors (called PGEA) and traditional method (called PGEB) were done as the following processes. PGEA: firstly, a suitable amount of PAA-PEG matrix was put into the solvent mixture of PY and Acac; owing to the Lewis acid-basic interaction, the matrix could easily swell in the mixture and absorb all of the mixture. Secondly, the swollen matrix was put into GBL (70 vt %) and NMP (30 vt %) mixture containing suitable amount of I2. The mole proportion among PY, Acac and I2 was kept at 2:1:1.2 according to the reaction Eq. (1), a little extra amount of I2 in the mixture is to form suitable amount of I3−. When I2 penetrated into the swollen matrix, the reaction occurred and formed I−/I3− ionic conductors in the polymer gel electrolyte. PGEB: firstly, GBL (70 vt %), NMP (30 vt %) and suitable amount of PY, Acac and I2 (mole proportion 2:1:1.2) were mixed to form liquid electrolyte; then the PAA-PEG matrix was swollen in the liquid electrolyte to form polymer gel electrolyte. For comparison, the amount of Acac-Py-I2 ionic conductors in PGEA and PGEB was set in the same value.
2.3 Assembling of quasi-solid-state dye-sensitized solar cell
The preparation of TiO2 colloidal paste and double-layer film was according to the method reported by Wang et al. [15]. The TiO2 colloidal paste was used to fabricate the transparent film about 10 μm. The optical diffuser film about 4 μm was printed above the transparent film with Ti-Nanoxide 300 paste. After sintering at 500 °C and cooling down to 80 °C, the double-layer nanostructured TiO2 electrodes were dye-coated with 2.5 × 10−4 M absolute ethanol solution of cis-[(dcbH2)2Ru(SCN)2] for 24 h.
A QS-DSSC was fabricated by sandwiching a slice of the polymer gel electrolyte between a dye-sensitized TiO2 electrode and an electrodeposited platinum counter electrode. The two electrodes were clipped together and a piece of cyanoacrylate adhesive (15–30 μm) was used as sealant. Bisphenol a epoxy resin (using ethylene diamine as epoxy hardener) was used for further sealing process.
2.4 Measurements
Liquid electrolyte absorbency (Qle) of the polymer gel electrolyte was defined as:
(2) |
The photovoltaic tests of QS-DSSC were carried out by measuring I–V curves under simulated AM 1.5 solar illumination at 100 mW·cm−2 from a xenon arc lamp (CHF- XM500, Trusttech Co., Ltd, China) in ambient atmosphere and recorded with CHI 660 C electrochemical workstation. The active area of QS-DSSC was 0.5 cm2 (1 × 0.5 cm2).
3 Results and discussion
Liquid electrolyte absorbency of the thermosetting polymer gel electrolyte is a key parameter influencing ionic conductivity. As shown in Fig. 1, PGEA shows higher liquid electrolyte absorbency than that of PGEB. The reasons can be illustrated as follows: for PGEA, by going with the formation of Acac-Py-I2 ionic conductors in the polymer gel electrolyte, the ionic concentration gradient between the polymer gel electrolyte and the GBL-NMP mixed solvents is formed, which is beneficial for the further penetration of GBL-NMP mixed solvents into PGEA due to the existing osmotic pressure, so PGEA shows high liquid electrolyte absorbency. While for PGEB, the formation of Acac-Py-I2 ionic conductors in the liquid electrolyte shows negative influence on the penetration of liquid electrolyte into PAA-PEG matrix because of the increased viscosity of the liquid electrolyte and the shrinkage effect of the high ionic concentration on PAA-PEG matrix, so PGEB shows lower liquid electrolyte absorbency.
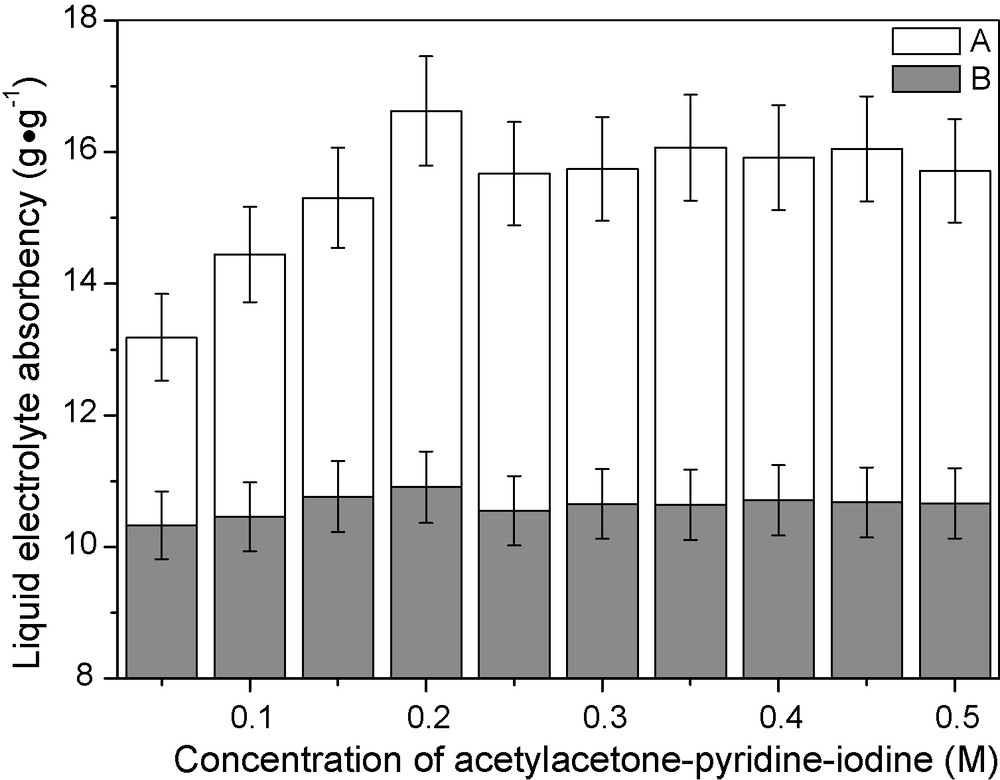
Liquid electrolyte absorbency of polymer gel electrolytes as functions of concentration of Acac-Py-I2 in γ-butyrolactone-N-methylpyrrolidone mixed solvents. A. PGEA. B. PGEB.
Fig. 2 shows the ionic conductivity of liquid electrolytes, PGEA and PGEB, with the changed concentration of Acac-Py-I2 ionic conductors. The ionic conductivity of the liquid electrolyte increases with the increased Acac-Py-I2 concentration, and there exist saturated values of ionic conductivity of PGEA and PGEB, which is owing to the saturated liquid electrolyte absorbency of the polymer gel electrolytes as shown in Fig. 1. From Fig. 2, it also can be seen that the ionic conductivity of PGEA is higher than that of PGEB with the same Acac-Py-I2 concentration because of the higher liquid electrolyte absorbency of PGEA.
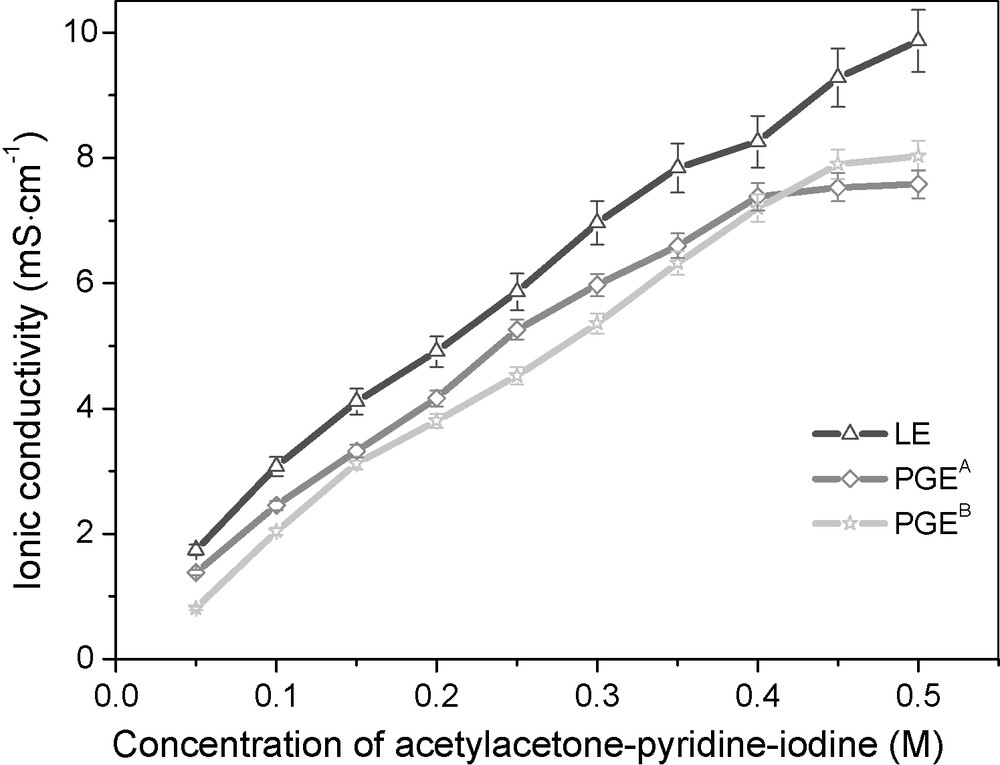
Ionic conductivity of liquid electrolyte (LE), PGEA and PGEB as functions of Acac-Py-I2 concentration in electroytes.
Fig. 3 shows the ionic conductivity change tendencies of PGEA and PGEB as functions of temperature. The ionic conductivity change tendencies of the two polymer gel electrolytes are similar. The ionic conductivity of PGEB increases quicker than that of PGEA with the increased temperature, which is owing to the different activation energy of Acac-Py-I2 ionic conductors in the two polymer gel electrolytes. Owing to the high liquid electrolyte absorbency of the polymer gel electrolytes, the transportation of ionic conductors in the polymer gel electrolytes mainly depends on their hopping movement through solvent channels, so the Arrhenius type relationship is used to fit the data presented in Fig. 3 [16].
(3) |
(4) |
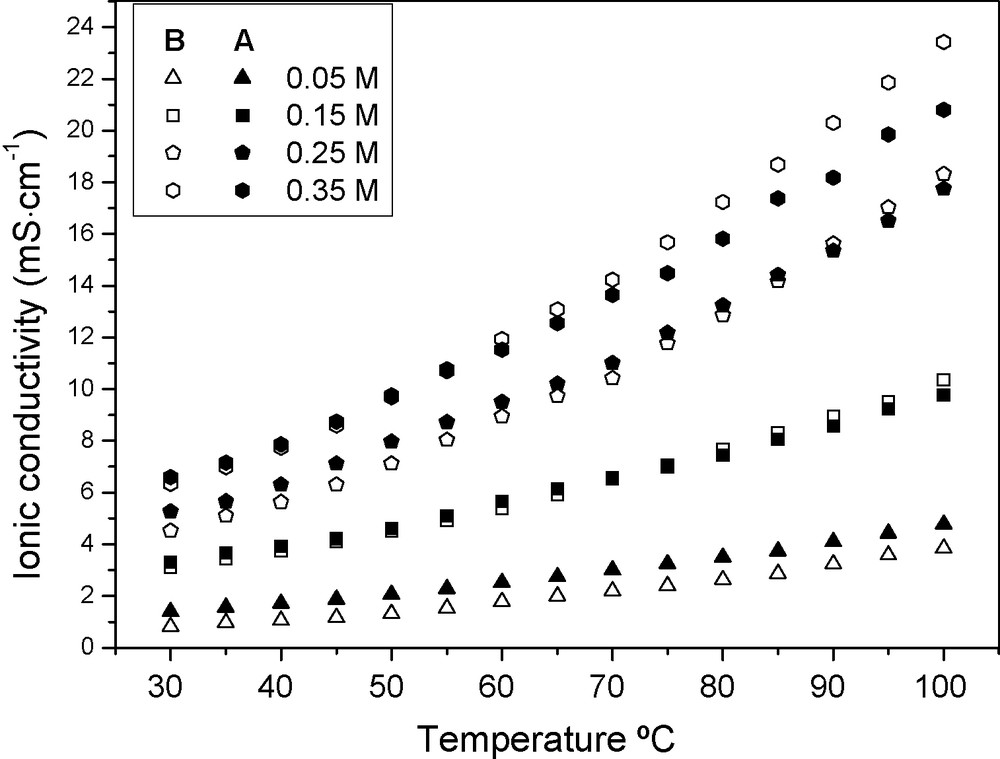
Ionic conductivity of PGEA and PGEB as functions of temperature.
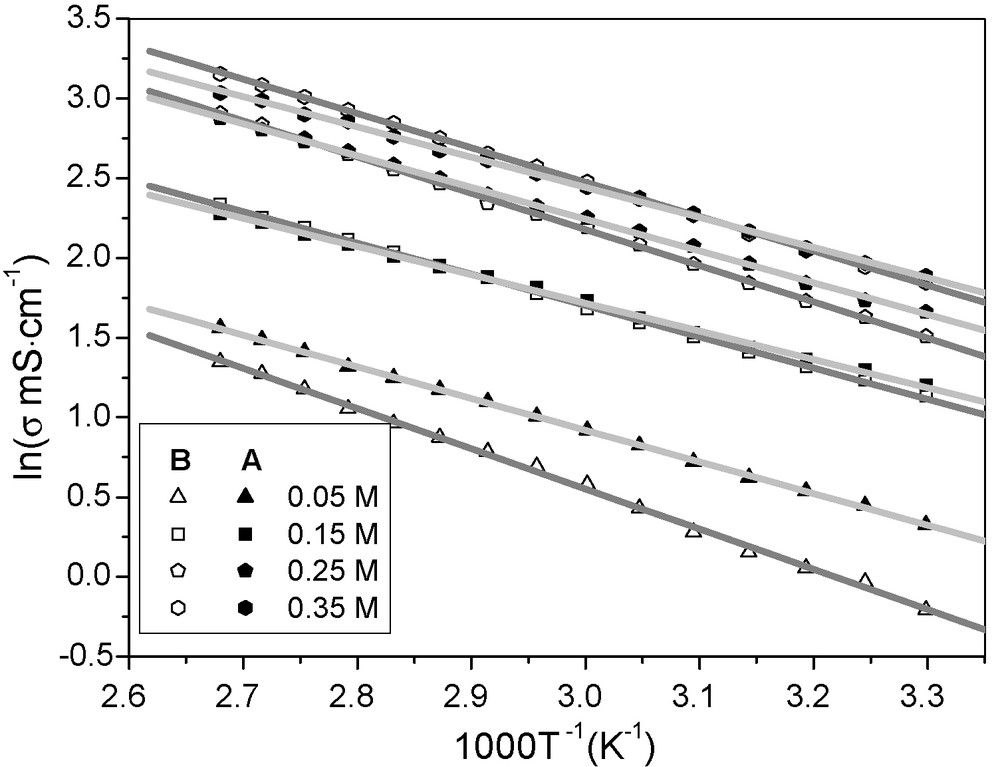
Temperature dependence of ionic conductivity of PGEA and PGEB.
The activation energy of Acac-Py-I2 ionic conductors in the polymer gel electrolytes are listed in Table 1. It is seen that Ea (B) is higher than Ea (A) when the polymer gel electrolytes contain the same amount of Acac-Py-I2 ionic conductors. One reason is that PGEA has higher liquid electrolyte absorbency, namely, it contains a larger amount of liquid components than that of PGEB, which makes for easier diffusion of Acac-Py-I2 ionic conductors in the polymer gel electrolyte, so the activation energy is lower. The other reason may be due to the more uniform distribution of in situ synthesized Acac-Py-I2 ionic conductors in PGEA, which is also beneficial for ionic diffusion.
Activation energy of Acac-Py-I2 ionic conductors in polymer gel electrolytes presented in Fig. 4.
Concentration (M) | 0.05 | 0.15 | 0.25 | 0.35 |
Ea(A) (kJ mol−1 K−1) | 16.523 ± 0.089 | 14.737 ± 0.161 | 16.562 ± 0.149 | 15.743 ± 0.128 |
Ea(B) (kJ mol−1 K−1) | 20.962 ± 0.263 | 16.300 ± 0.130 | 18.910 ± 0.140 | 17.921 ± 0.097 |
Fig. 5 shows the influence of Acac-Py-I2 concentration in the polymer gel electrolytes on the photovoltaic performance of QS-DSSC. It is seen that the maximum short-circuit current density and open-circuit voltage of QS-DSSC with PGEA can be obtained when the Acac-Py-I2 concentration is 0.25 M, while for QS-DSSC with PGEB, the case is different, the maximum open-circuit voltage is obtained when the Acac-Py-I2 concentration is 0.3 M, and the maximum value of short-circuit current density is obtained when the Acac-Py-I2 concentration is 0.35 M. The optimized Acac-Py-I2 concentration in PGEB is selected as 0.3 M by taking into account of the large decreased open-circuit voltage with 0.35 M ionic conductors.

Influence of Acac-Py-I2 concentration on the photovoltaic performance of quasi-solid-state dye-sensitized solar cells with PGEA and PGEB.
Fig. 6 shows the photovoltaic performance of QS-DSSCs with the optimized Acac-Py-I2 concentration in PGEA and PGEB. It is seen that the two cells have similar open-circuit voltage, while the QS-DSSC with PGEA shows higher short-circuit current density than the QS-DSSC with PGEB. The reason is mainly due to the different liquid electrolyte absorbency of the two polymer gel electrolytes. From Figs. 2 and 1, the two kinds of polymer gel electrolytes with the selected Acac-Py-I2 concentration show the same ionic conductivity, while their liquid electrolyte absorbency is different. It is known that polymer gel electrolyte contains a higher amount of liquid component and can form better interfacial contact with electrodes, which is important for light-to-electricity conversion in QS-DSSC. The energy conversion efficiencies of QS-DSSCs containing PGEA and PGEB show 3.815 and 3.138%, so it is verified that polymer gel electrolyte with in situ synthesized Acac-Py-I2 ionic conductors shows some advantages in preparing QS-DSSCs than that of polymer gel electrolyte formed by traditional method.
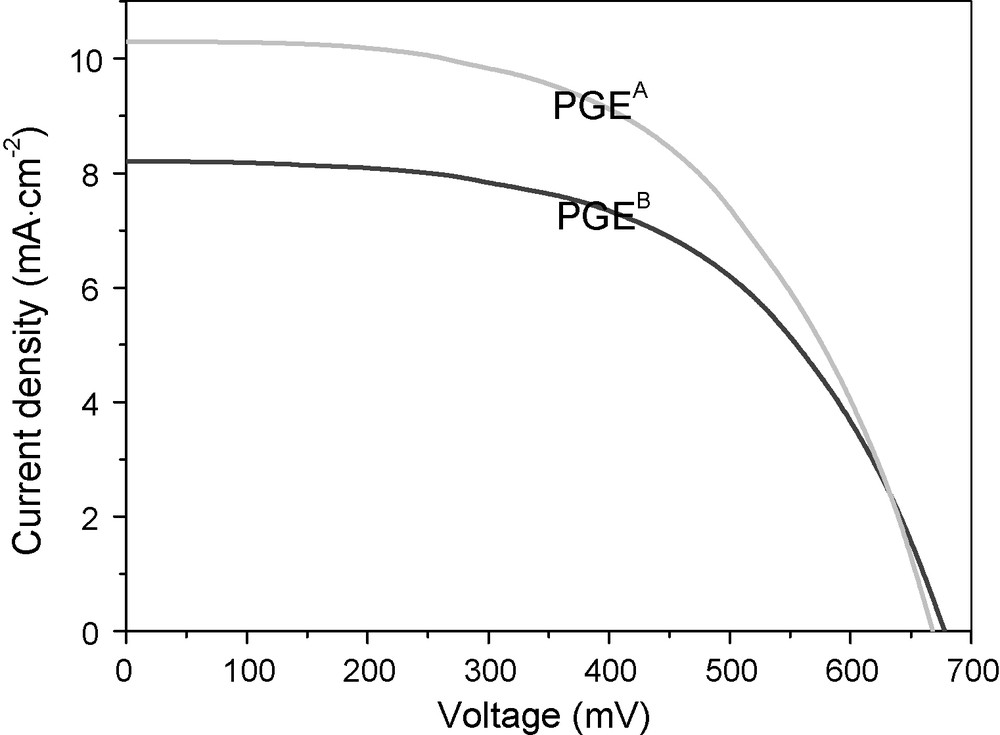
Photovoltage-current density curves of quasi-solid-state dye-sensitized solar cells with PGEA and PGEB (the Acac-Py-I2 concentration is 0.25 M in PGEA, and 0.3 M in PGEB).
4 Conclusions
A polymer gel electrolyte with in situ synthesized Acac-Py-I2 ionic conductors is prepared for fabricating QS-DSSC. It is found that the in situ synthesized Acac-Py-I2 ionic conductors show weaker influence on the liquid electrolyte absorbency of polymer gel electrolyte than that of traditional method of soaking polymer matrix in liquid electrolyte to form gel state. Owing to the higher liquid electrolyte absorbency of PGEA, it shows higher ionic conductivity than that of PGEB. QS-DSSC with PGEA shows a 3.815% energy conversion efficiency, which is about 21.6% higher than that of QS-DSSC with PGEB. So it is verified that polymer gel electrolyte with in situ synthesized Acac-Py-I2 ionic conductors shows some advantages in preparing QS-DSSC comparing with the traditional polymer gel electrolyte formed by swollen polymer matrix in liquid electrolyte.
Acknowledgements
The authors acknowledge the joint support of the National High Technology Research and Development Program of China (863 Program) (No. 2009AA03Z217), the National Natural Science Foundation of China (No. 90922028, 50842027), the Major Research Plan of National Natural Science Foundation of China (No. 90922028) and the Natural Science Foundation of Hua Qiao University (No. 09BS401).