1 Introduction
Ionic liquids offer the potential for ground-breaking changes to synthesis routes and unit operations in research as well as in the chemical industry. Although the ionic liquid was initially introduced as an alternative green reaction medium, today it has marched far beyond showing its significant role in controlling the reaction as catalyst. The large number of cations and anions allow a wide range of physical and chemical characteristics to be achieved, including volatile and involatile systems, and thus the terms “designer” and “task-specific” ILs have been developed [1–4]. The prospects for ionic liquid use are vast. A number of ionic liquids with unique properties have been developed and applied to catalyze many types of reactions. Recently, basic ionic liquids have aroused unprecedented interest because they showed more advantages, such as catalytic efficiency and recycling of the ionic liquid, than the combination of an inorganic base and an ionic liquid for some base-catalyzed processes [5].
Tetrahydrobenzo[b]pyrans have recently attracted attention as an important class of heterocyclic scaffolds in the field of drugs and pharmaceuticals. These compounds are widely used as anti-coagulant, diuretic, spasmolytic, anticancer and anti-anaphylactin agents [6]. In addition, they can be used as cognitive enhancers, for the treatment of neurodegenerative disease, including Alzheimer's disease, amyotrophic lateral sclerosis, Huntington's disease, Parkinson's disease, AIDS associated dementia and Down's syndrome as well as for the treatment of schizophrenia and myoclonus [7]. Some 2-amino-tetrahydrobenzo[b]pyrans can be employed as photoactive materials [8]. Synthesis of tetrahydrobenzo[b]pyrans was traditionally promoted by harsh bases, strong acids or high temperature in the presence of VOCs [9–17]. A little effort has been made in view of green synthesis of tetrahydrobenzo[b]pyrans [18–20]. Surprisingly, there have been no reports regarding the use of the amino functionalized ionic liquids in an effort to influence the outcome of multicomponent reactions. In this report we will describe the synthesis of a functionalized ionic liquid, 4-amino-1-(2,3-dihydroxy propyl) pyridinium hydroxide [ADPPY] [OH] and its application as a mild catalyst for the synthesis of tetrahydrobenzo[b]pyrans.
The basic idea here is to replace the scavenger reagents by a functionalized ionic liquid. In comparison with the other scavengers, this synthetic approach has several outstanding advantages, including a high active site of IL, time saving, easy monitoring and unlimited scale-up potential in water. It is also worth noting that the used ionic liquids could be regenerated and recycled at least five times without significant loss of activity.
2 Results and discussion
2.1 Effect of different ILs
Our efforts were focused on the synthesis of the tetrahydrobenzo[b]pyrans using amino functionalized ionic liquids in comparison with other ILs. The results are summarized in Table 1.
Optimization of reaction conditions for the synthesis of tetrahydrobenzo[b]pyran in different ILs as a catalyst.
Entry | Ionic Liquids | Solventb | Mol% | Time (min.) | Yield (%)a |
1 | [bmim]BF4 | H2O | 40 | 480 | 0 |
2 | [bmim]OH | H2O | 40 | 50 | 75 |
3 | [bmim]PF6 | H2O + C2H5OH (70:30) | 40 | 120 | 46 |
4 | [bpyr]BF4 | H2O | 40 | 240 | 20 |
5 | [bpyr]PF6 | H2O + C2H5OH (70:30) | 40 | 180 | 35 |
6 | [omim]BF4 | H2O | 40 | 180 | 55 |
7 | [omim]PF6 | H2O + C2H5OH (70:30) | 40 | 180 | 61 |
8 | [ADPPY]OH | H2O + C2H5OH (90:10) | 10 | 15 | 90 |
9 | [ADPPY]OH | H2O | 05 | 25 | 78 |
10 | [ADPPY]OH | H2O | 10 | 10 | 95 |
11 | [ADPPY]OH | H2O | 15 | 15 | 92 |
12 | [ADPPY]OH | H2O | 30 | 15 | 91 |
13 | [ADPPY]OH | H2O | 45 | 15 | 91 |
a All reactions were carried out of benzaldehyde: malononitrile: dimedone 1:1:1 (molar ratio) at room temperature in ionic liquids; yield refer to pure isolated products.
b Entries in bracket indicate the ratio of H2O to C2H5OH on volume basis.
As shown in Table 1, the molar ratio of IL/substrate and the reaction times both have significant effects on the reaction. It could be seen that with the increase of the molar ratio of IL/substrate from 5 to 10%, the reaction yield was significantly improved and then it reduces (Table 1). Upon examination, it was found that 10 mol% of IL was sufficient to promote the reaction. In the presence of less than this amount, the yield dropped dramatically, even if longer reaction times were used (Table 1, entry 9). When the amount of IL was increased over 10 mol% equivalent, neither the yield nor the reaction time was improved (Table 1, entries 11–13). Experiments show that the catalytic performance of [ADPPY] [OH], [BMIM] [OH] could be much better than other ILs under the same reaction conditions. It is evident that the yields of the desired product in the ionic liquids containing the hydroxyl anion are higher than those in the ionic liquids containing tetrafluoroborate as well as hexaflurophosphate.
Furthermore, it can be seen that the conversion for reaction with [ADPPY] [OH] as catalyst was better than [BMIM] [OH]. These results suggest that the performance of the IL is dependent upon the character of the side chain of the cation and the N-heterocyclic ring. [ADPPY] [OH] exhibited the most excellent catalytic performance with high conversion under the same reaction conditions (Table 2, entry 10).
[ADPPY] [OH] catalyzed synthesis of tetrahydrobenzo[b]pyran derivatives.
Entry | Ar | Producta | Time (min.) | R1,R2 | Yield (%)b |
1 | 4-Cl-C6H4 | 4a | 15 | CH3,CH3 | 94 |
2 | 4-OMe-C6H4 | 4b | 20 | CH3,CH3 | 90 |
3 | C6H5 | 4c | 15 | CH3,CH3 | 95 |
4 | 2,5-2Cl-C6H3 | 4d | 25 | CH3,CH3 | 89 |
5 | 3-Pyridine | 4e | 15 | CH3,CH3 | 84 |
6 | 3-Pyridine | 4f | 20 | H,H | 92 |
7 | Thiophene | 4g | 20 | CH3,CH3 | 89 |
8 | 3,4-2CH3-C6H3 | 4h | 25 | CH3,CH3 | 91 |
9 | 3,4-2CH3-C6H3 | 4i | 20 | H,H | 94 |
10 | 4-isopropyl benzaldehyde | 4j | 25 | CH3,CH3 | 90 |
11 | C6H11 | 4k | 25 | CH3,CH3 | 89 |
12 | 4-CN-C6H4 | 4L | 20 | CH3,CH3 | 90 |
2.2 Reusability of [ADPPY] [OH] as catalyst
As one of the most effective functionalized IL, [ADPPY] [OH] was selected to investigate the possibility of reusability (Fig. 1). As the product is insoluble in water, it was collected by simple filtration, while the ionic liquid is completely soluble in water. So, after completion of reaction, it became very easy to isolate the product from the ionic liquid. The IL could be recovered easily by rotary evaporator and directly reused for subsequent runs. As it is shown in Fig. 1, no obvious change was observed on the recovered catalytic and excellent yield was obtained. After five times reusability, the IL remains stable and has less impact on the catalytic activity.
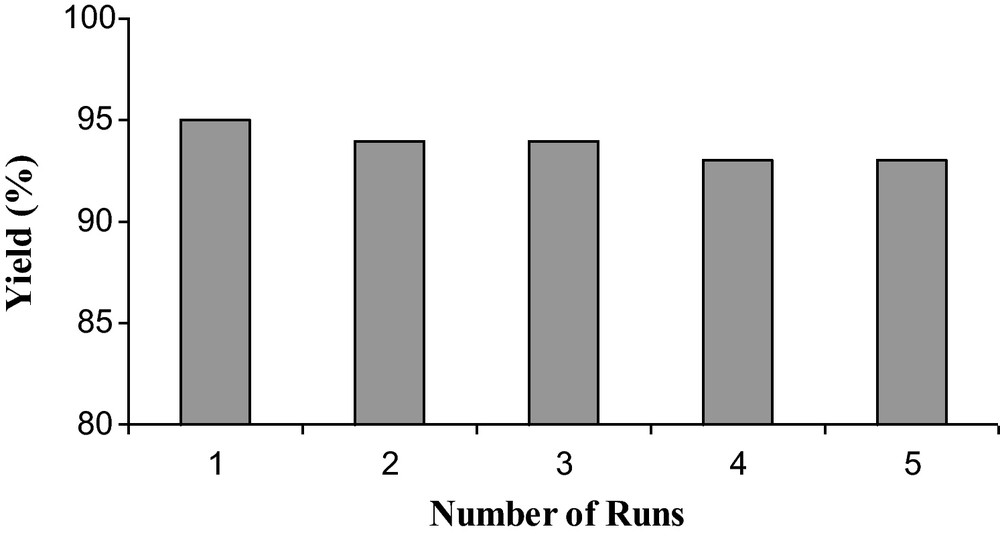
Reusability of [ADPPY] [OH].
3 Conclusion
In summary, we have introduced a new amino functionalized ionic liquid ([ADPPY] [OH]) that can be used as an efficient catalyst for tetrahydrobenzo[b]pyrans. The heterocycles synthesized in this study were obtained in high regioselectivity, good yields and short reaction times.
4 Experimental
4.1 Synthesis of the ionic liquids (ILs)
A novel amino functionalized ionic liquid 4-amino-1-(2,3-dihydroxy propyl) pyridinium, hydroxide [ADPPY] [OH] (Fig. 2) was synthesized from commercially available 4-amino pyridine and 3-Chloro-1,2-propanediol, followed by anion exchange with Amberlite IRA 400 Cl resin (Scheme 1) [21]. The product (87% yield) was characterized by 1H and 13C NMR spectroscopy.
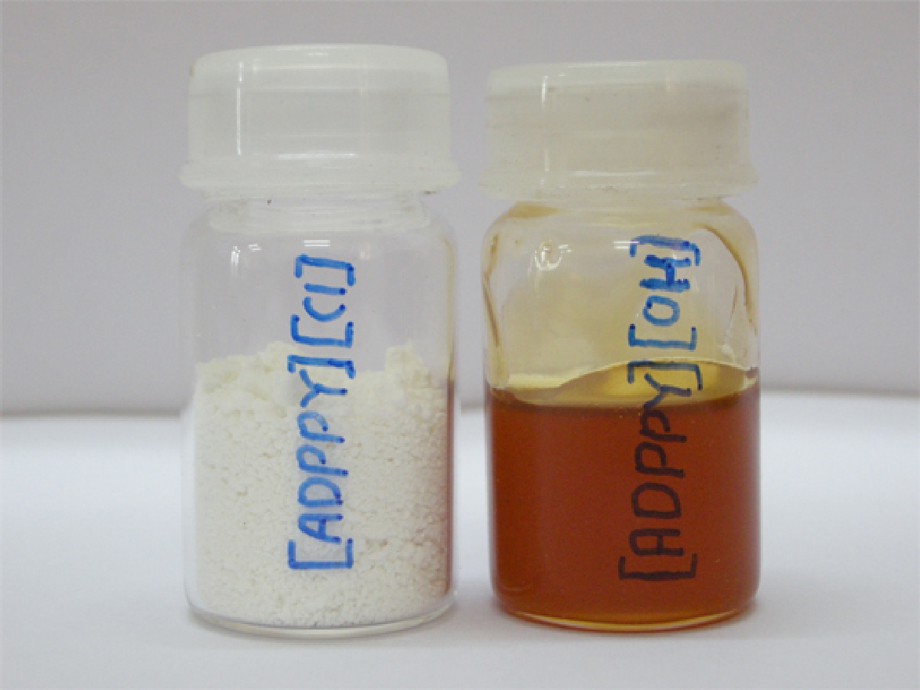
Images of TSILs.

Synthesis of TSIL [ADPPY] [OH].
4.2 Synthesis of tetrahydrobenzo[b]pyran
To demonstrate the utility of [ADPPY][OH] in multicomponent reaction, we began with a mixture of aldehydes (1 mmol), malononitrile (1 mmol) and 1,3-di ketone (1 mmol) as a model. Unfortunately, as the ionic liquid is very viscous, water (3 mL) was used as sequester. The reaction was carried out at room temperature with constant stirring for just 10 minutes to get a single product in excellent yield (95%). The formation of the product was further confirmed by IR, 1H NMR and 13C NMR spectroscopy. It is worthy to mention that no by-product was detected. A variety of aldehydes and 1,3-diketone also underwent to afford the corresponding tetrahydrobenzo[b]pyrans in moderate to high yields. Aromatic aldehydes with either electron donating or electron withdrawing groups underwent the reaction smoothly. Having obtained favourable results with aromatic aldehydes, we then examined heterocyclic aldehydes such as pyridine-3-carboxylaldehyde and 2-furaldehyde. The results are summarized in Table 2. A conceivable mechanism for the multicomponent synthesis of tetrahydrobenzo[b]pyran using [ADPPY] [OH] is proposed (Schemes 2 and 3).
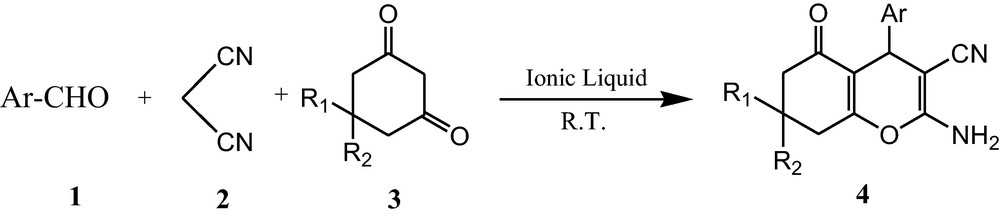
Synthesis of tetrahydrobenzo[b]pyran.
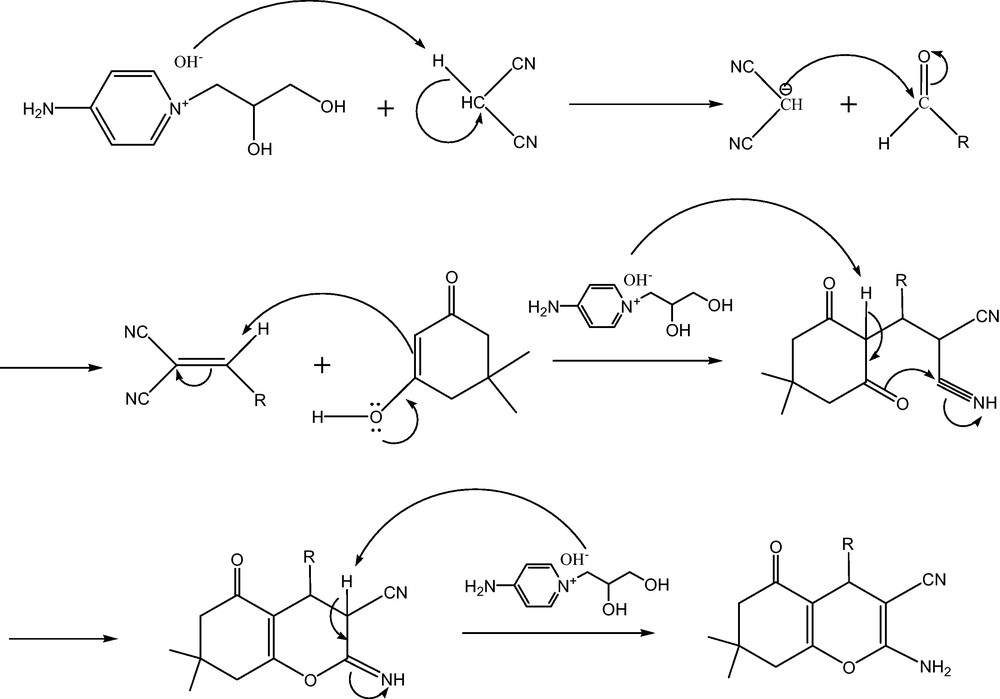
A conceivable mechanism for formation of tetrahydrobenzo[b]pyran.
4.3 Spectral data
4.3.1 Spectral data for TSIL (4-amino-1-(2,3-dihydroxy propyl) pyridinium, hydroxide)
1H NMR (300 MHz, DMSO-d6): δ 3.179 (m, 2H), 3.725 (s, 1H), 3.953–4.024 (m, 1H), 4.229–4.284 (m, 1H), 5.067 (t, 1H, OH), 5.425 (d, 1H, OH), 6.854 (d, J = 7.2 Hz, 2H), 8.090 (d, J = 7.2 Hz, 2H), 8.316 (bs, 2H, NH2). 13C NMR (75 MHz, DMSO): δ 60.24, 63.07, 70.78, 109.24, 144.03, 159.15.
4.3.2 Spectral data for unknown tetrahydrobenzo[b]pyran derivatives
Table-2, Entry 4e: mp 184–186 ̊C; IR (KBr): 3377, 3313, 2959, 2184, 1683, 1653, 1373, 1216, 1035, 712 cm−1; 1H NMR (300 MHz, CDCl3): δ 1.019 (s, 3H, CH3), 1.107 (s, 3H, CH3), 2.209 (s, 2H, CH2), 2.460 (s, 2H, CH2), 4.433 (s, 1H, Benzylic CH), 4.992 (s, 2H, NH2), 7.268 (s, J = 12 Hz, 1H, Ar-CH), 7.656 (s, J = 7.8 Hz, 1H, Ar-CH), 8.455 (d, 2H, Ar-CH); 13C NMR (75 MHz, CDCl3): δ 27.71, 28.74, 32.21, 33.65, 40.63, 50.54, 61.57, 113.08, 118.38, 123.61, 136.06, 139.12, 147.75, 148.71, 158.08, 162.17, 195.79. M/z = 296 (M + 1); Purity by LC-MS = 98.16%.
Table-2, Entry 4f: mp 204–205 ̊C; IR (KBr): 3360, 3329, 3036, 2192, 1665, 1524, 1366, 1214, 1028, 714 cm−1; 1H NMR (300 MHz, CDCl3): δ 1.989 (s, 2H, CH2), 2.253 (s, 2H, CH2), 2.516 (s, 2H, CH2), 4.306 (s, 1H, Benzylic CH), 6.298 (s, 2H, NH2), 7.238 (s, J = 12.6 MHz, 1H, Ar-CH), 7.577 (s, J = 14.1, 1H, Ar-CH), 8.360 (d, 2H, Ar-CH); 13C NMR (75 MHz, CDCl3): δ 20.06, 27.05, 33.74, 36.64, 58.49, 113.81, 119.38, 123.85, 136.30, 140.23, 147.23, 148.25, 158.91, 164.48, 195.91. M/z = 268 (M + 1); Purity by LC-MS = 74.78%.
Table-2, Entry 4 h: mp 201–202 ̊C; IR (KBr): 3451, 3329, 2964, 2193, 1670, 1600, 1365, 1206, 1039, 773 cm−1; 1H NMR (300 MHz, DMSO-d6 in CDCl3): δ 1.060 (s, 3H, CH3), 1.113 (s, 3H, CH3), 2.222 (s, 2H, CH2), 2.222 (s, 3H, Ar-CH3), 2.222(s, 3H, Ar-CH3), 2.459 (s, 2H, CH2), 4.325 (s, 1H, Benzylic CH), 4.572 (s, 2H, NH2), 6.949 (d, J = 16 MHz, 2H, Ar-CH), 7.057 (s, 1H, Ar-CH); 13C NMR (75 MHz, DMSO in CDCl3): δ 19.4, 19.9, 27.72, 28.29, 32.21, 35.09, 40.68, 50.69, 63.76, 114.10, 118.83, 124.76, 128.77, 129.87, 135.35, 136.61, 140.71, 157.35, 161.46, 196.00. M/z = 323 (M + 1); Purity by LC-MS = 91.00%.
Table-2, Entry 4i: mp 198–200 ̊C; IR (KBr): 3396, 3329, 2918, 2192, 1680, 1657, 1364, 1207, 1021, 795 cm−1; 1H NMR (300 MHz, DMSO-d6 in CDCl3): δ 6.93 (s, 1H, Ar-CH), 6.84 (d, J = 13.8 MHz, 2H, Ar-CH), 6.57 (s, 2H, NH2), 4.11 (s, 1H, Benzylic CH), 2.62 (s, 2H, CH2), 2.35 (s, 2H, CH2), 1.95 (s, 2H, CH2), 2.12 (s, 3H, Ar-CH3), 2.15 (s, 3H, Ar-CH3); 13C NMR (75 MHz, DMSO in CDCl3): δ 13.39, 19.96, 20.23, 27.05, 35.37, 36.84, 60.00, 114.81, 120.11, 124.94, 128.70, 129.65, 134.66, 136.12, 142.31, 158.72, 163.98, 195.90. M/z = 317 (M + Na); Purity by LC-MS = 100%.
Acknowledgment
The authors P.P.S. and S.S.K. thanks to DAE–BRNS, New Delhi (India) for the financial assistance and a research fellowship respectively.