1 Introduction
Ionic liquids (ILs) have attracted great attention in a variety of different areas, reaching from material synthesis to separation science as well as alternative reaction media [1]. However, despite promising results, their widespread use in process chemistry is still hampered by the following practical drawbacks:
- • homogeneous reaction, which was difficult for product separation and catalyst recovery;
- • high viscosity, which results in only a minor part of ionic liquids take part in the catalyzed reaction;
- • the use of relatively large amounts of the ionic liquids, which is costly and may cause possible toxicological concerns [2].
To overcome these drawbacks, the concept of supported ionic liquid catalysis (SILC) has been established to combine the advantages of ionic liquids with those heterogeneous support materials. Many reports about the preparation of supported ionic liquid catalysts have been published and the ionic liquids were normally immobilized onto the support materials, such as silica gel [3–5], mesoporous silica [6–8], magnetic nanoparticles [9–11] and polymers [12–14]. But in the normal immobilization of ionic liquids, the amount of IL, which was loaded onto the surface of support materials, was very low due to surface immobilization occurring only in one surface layer. Among the support materials, magnetic nanoparticles are more interesting due to the large surface area-to-volume ratio and simple recyclability and reusability.
One of the processes used in the synthesis of pharmaceutical compounds is a base-catalyzed reaction with a homogeneous catalyst, such as KOH or NaOH. This reaction can produce products with high yield under mild conditions. However, for satisfactory use of the products as pharmaceutical compounds, the base catalyst must be removed from it, requiring a cumbersome purification process. Moreover, the process wastewater is environmentally toxic, corrosive and the catalyst could not be recovered. Thus, it is still a challenge to find novel heterogeneous Brønsted-type basic catalysts with high activity and selectivity, combined with simple separation from the reaction medium. However, many Brønsted-type basic catalysts were previously supported onto many support materials, but they suffer problems, such as low loading and low thermal stability [15,16].
Herein, we report novel heterogeneous Brønsted-type basic catalysts based on Fe3O4 magnetic nanoparticle (MNP) coated with the multilayers of poly(ethylvinylimidazolium) hydroxide, which proved to be highly efficient for the synthesis of 4H-benzo[b]pyrans in water.
The 4H-benzo[b]pyran family have attracted considerable attention as an important heterocyclic compounds because of their wide range of biological properties, such as anti-cancer, anti-coagulant, anti anaphylactic activities [17]. The conventional method for synthesis of 4H-benzo[b]pyrans is using organic solvents such as DMF or acetic acid under reflux temperature, which gives poor yields [18]. Several catalytic systems have been used for improvement of the products yields [19–31]; however, most of these methods suffer from some disadvantages, such as toxic reagents, long reaction times, no recyclability of catalysts and use of toxic solvents. Therefore, to overcome these drawbacks a great deal of efforts is directed to develop an efficient catalyst for the synthesis of these compounds under green conditions.
2 Experimental
2.1 Reagents and analysis
Ferric chloride hexahydrate (FeCl3·6H2O), ammonia (30%), ferrous chloride tetrahydrate (FeCl2·4H2O) and 3-(trimethoxysilyl)propylmethacrylate (MPS, 98%) were obtained from Merck. 1-vinylimidazole was obtained from Aldrich and was distilled before use. Bromoethane and 1,4-dibromobutane were obtained from Aldrich. 2,2′-Azobisisobutyronitrile (AIBN, Kanto, 97%) was recrystallized from ethanol.
Thin layer chromatography (TLC) was performed with silica gel 60 F254 plates and UV light was used for visualization. FT-IR spectra of samples were taken using an ABB Bomem MB-100 FT-IR spectrophotometer. The samples were powdered and mixed with KBr to make pellets. Proton nuclear magnetic resonance spectra (1H NMR) were recorded on a Brucker NMR 500 MHz instrument. Thermogravimetric analysis (TGA) was acquired under a nitrogen atmosphere with a TGA Q 50 thermogravimetric analyzer. Transmission electron microscopy (TEM) images were taken with a TOPCON-002B electron microscope. The magnetic property of catalyst was measured by a vibrating sample magnetometer (VSM) (Model 7400).
2.2 Synthesis of magnetic nanoparticles
The magnetic Fe3O4 nanoparticles were prepared by chemical co-precipitation method. An iron salt solution was obtained by mixing 13.6 g FeCl3·6H2O and 5 g FeCl2·4H2O in 500 mL deionized water under nitrogen at room temperature. By adding dropwise a NH3 solution, the solution pH was adjusted to 12. A black precipitate was formed after continuously stirring for 1 h. The precipitate was magnetically separated and washed four times with the deionized water and two times with ethanol and was dried under vacuum at 50 °C overnight.
2.3 Surface modification of magnetic nanoparticles
3 g MNP were dispersed in 40 mL of a 4/1 mixture of ethanol/water, then 2 mL of ammonium hydroxide 25% solution was added. Afterward, an excess amount (10 mmol per 1 g of Fe3O4) of the MPS was added dropwise over a period of 10 min, and the reaction mixture was stirred at 50 °C for 48 h. The modified MNPs were washed three times with methanol and magnetically separated to remove any excess of reagent and salts.
2.4 Synthesis of ionic liquid monomer and cross-linker
5 mmol of 1-vinylimidazole, 5 mmol of ethyl bromide were added to 30 mL of methanol in a 100 mL round bottom flask at 0 °C. The mixture was stirred at room temperature overnight, followed by 60 °C for 15 h. After cooling down, the reaction mixture was added dropwise into 250 mL of diethyl ether and a white precipitate was formed. The precipitate was filtered off and washed with diethyl ether again and dried at room temperature until constant weight. (1H NMR, CDCl3, δ ppm): 1.66 (t, 3H), 4.53 (q, 2H), 5.42 (d, 1H), 6.00 (d, 1H), 7.48 (dd, 1H), 7.70 (s, 1H), 7.87 (s, 1H), 10.97 (s, 1H).
1,4-butanediyl-3,3′-bis-l-vinylimidazolium dibromide (BVD), as cross-linking agent, was prepared in the same manner except that the molar ratio of 1-vinylimidazole to 1,4-dibromobutane was 2:1 in the reaction. (1H NMR, DMSO, δ ppm): 1.87 (s, 2H), 4.29 (s, 2H), 5.43 (d, 1H), 6.00 (d, 1H), 7.33 (dd, 1H), 7.99 (s, 1H), 8.24 (s, 1H), 9.69 (s, 1H).
2.5 Synthesis of poly(ionic liquid) coated magnetic nanoparticles
The MNP@P[imEt][OH] was prepared by distillation-precipitation-polymerization of IL monomer and [BVD] as the cross-linker and AIBN as the initiator, in methanol. Typically, 200 mg of MNP@MPS nanoparticles were dispersed by ultrasonic in 100 mL methanol in a 250 mL single-necked flask for 10 min. Then, a mixture of 2.5 mmol of 1-ethyl-3-vinylimidazolum bromide, 0.5 mmol of [BVD], and 2.5 mg of AIBN were added to the flask to initiate the polymerization. The mixture was completely deoxygenated by bubbling purified argon for 30 min. The flask submerged in a heating oil bath was attached with a fractionating column, Liebig condenser, and a receiver. The reaction mixture was heated from ambient temperature to the boiling state within 1 h and the reaction was ended after about 60 mL of methanol was distilled from the reaction mixture within 5 h. The obtained MNP@P[imEt][Br] were collected by magnetic separation and washed two times with water and three times with methanol to eliminate excess reactants and few generated polymer microspheres. After separation of MNP@P[imEt][Br], the catalyst was stirred in excess KOH (1 M) solution for 2 days at room temperature. Then the catalyst was magnetically separated and washed three times with water and two times with methanol and dried under vacuum at 50 °C for 2 h. To ensure that all bromide ions are exchanged with hydroxide ions in catalyst, the AgNO3 test was used and it was found that only 7% of bromide ion was not exchanged. The loading of the hydroxide anion in the catalyst was calculated by titration with HCl (0.01 M) and it was found to be 2.1 mmol/g.
2.6 General procedure for synthesis of 4H-benzo[b]pyrans
In a 10 mL round bottom flask, aldehyde (1 mmol), malononitrile (1.2 mmol), water (5 mL), MNP@P[imEt][OH] (5 mol%) was added and the mixture was stirred for 15 min at room temperature. Then, dimedone (1 mmol) was added to the flask and the mixture was refluxed for an appropriate time. After completion of the reaction, monitored by TLC, the reaction mixture was cooled down to room temperature and diluted with hot ethylacetate, then the catalyst was magnetically separated and washed two times with methanol and dried under vacuum. The organic layer was concentrated under vacuum and the resulting solid products were recrystallized in ethanol.
3 Results and discussion
A co-precipitation method was used for the synthesis of MNPs. Surface modification of MNPs with MPS before polymer coating causes grafting of polymer chains onto the surface of MNPs by covalent bonding. On the other hand, modification of MNPs by methacrylate groups cause polymerization to become easier on the surface and all magnetic nanoparticles will be grafted by a copolymer shell [32]. Fig. 1 shows FT-IR spectra of MNP before (Fig. 1a) and after (Fig. 1b) surface modification with MPS. The spectra of MNP@MPS nanoparticles and pure MPS (Fig. 1c) indicate a significant vibrational absorption of the Si–CH2 bond in the fingerprint region between 1326 and 1299 cm−1. Another characteristic signal can be attributed to the asymmetric Si–O–Si vibration between 1172 and 943 cm−1. In addition, the spectrum of the methacryloxy group-bearing particles shows the vibrational absorption of the carbonyl double bond at 1718 cm−1. From these data, it could result that MNPs were successfully modified with MPS. From the weight loss in the TGA curves of MNP and MNP@MPS on Fig. 2a, b respectively, it is calculated that the loading of MPS onto the MNPs surface was 0.54 mmol/g.

FT-IR spectrum of bare MNP(a), MNP@MPS (b), pure MPS (c), Ethylvinylimidazolium bromide (d), MNP@P[imEt][OH] (e).
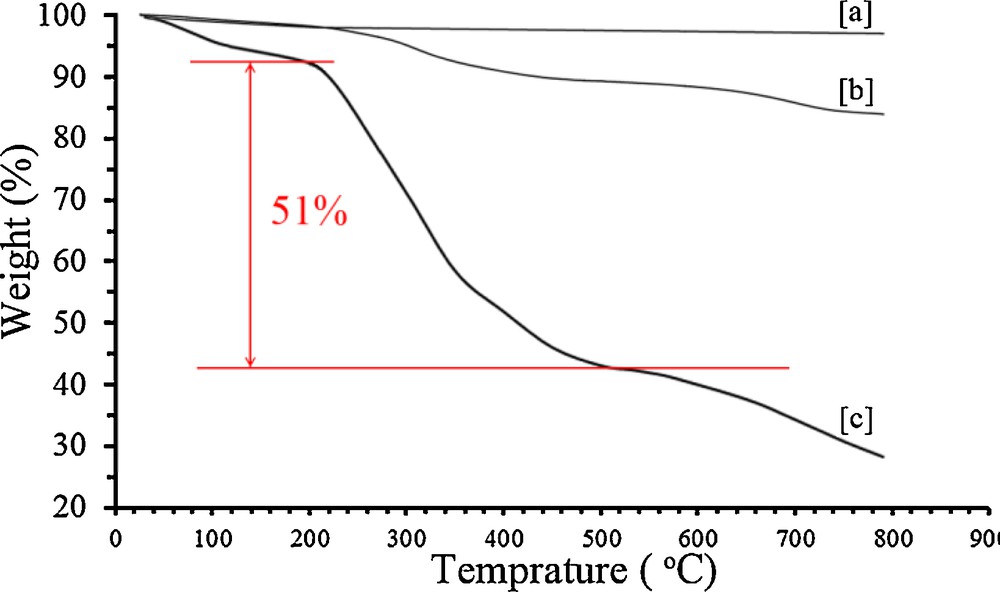
TG analysis of bare MNP (a), MNP@MPS (b) and MNP@P[imEt][OH] (c).
The procedure for synthesis of ionic liquid monomer 1-vinyl-3-ethylimidazolium bromide ([VEim][Br]) and cross-linker (BVD) is shown in Scheme 1. The ionic liquid monomer was synthesized by the quaternization of 1-vinylimidazole with ethyl bromide. For preparation of [BVD], quaternization was performed by 1,4-dibromobutane and two equivalent of 1-vinylimidazole.

Synthetic route for ionic liquid monomer and cross-linker.
The procedure for the synthesis of magnetic nanoparticle coated multilayered poly (1-vinyl-3-ethyl imidazolium hydroxide), noted as MNP@P[imEt][OH] is depicted in Scheme 2. Coating of multilayers of PIL onto the MNPs has some advantages over conventional grafting of material onto the surface of MNPs. In the normal grafting, only one layer of surface could be grafted by the homogenous catalyst. However, by multilayer polymer coating, the available-surface limitation for grafting would be removed. This approach causes loading of grafted homogenous material to increase and the catalyst will be used in a low weight percent compared to the substrates. This would be useful in large-scale applications. On the other hand, polymer chains will prevent aggregation of nanocatalysts in high temperature applications. MNP@P[imEt][OH] were synthesized by the distillation–precipitation–polymerization method in the presence of MNPs as heterogeneous bed. The polymerization was initiated by AIBN in the large amount of methanol at 70 °C. As copolymers are insoluble in the methanol (because of cross-linking), the generated copolymers will continuously precipitate from the solution and attach to the surface of the magnetic nanoparticles to form a multilayered shell. For exchange of bromide with hydroxide ion, MNP@P[imEt][Br] was treated by a KOH solution for 48 h at room temperature (Fig. 1).
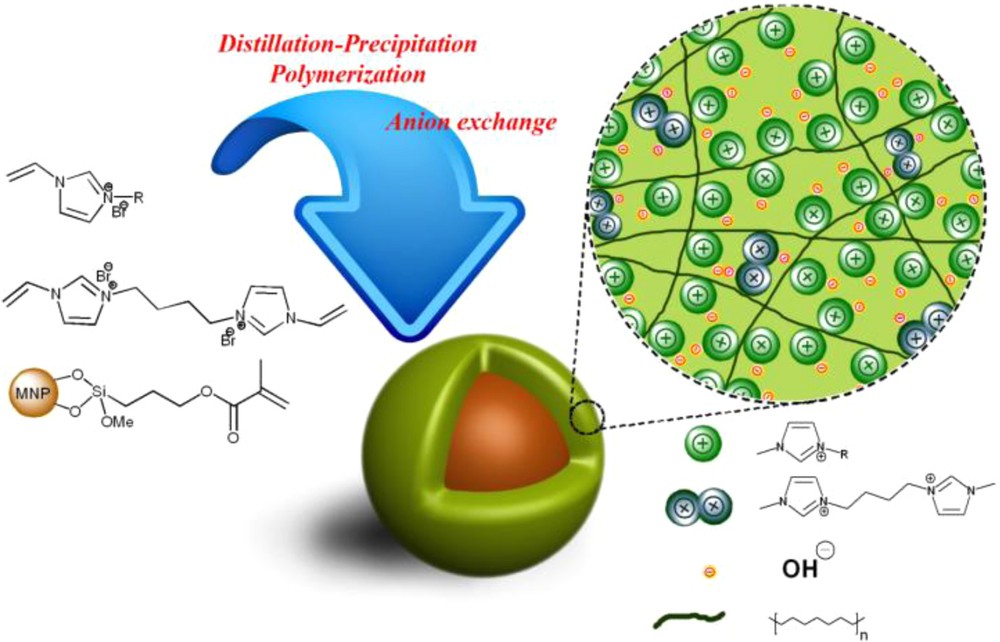
General procedure for synthesis of MNP@P[imEt][OH].
Fig. 1d shows the FT-IR spectrum of 1-ethyl-3-vinylimidazolium bromide with a characteristic absorption peak at 1574 cm−1, which is attributed to a stretching vibration of the imidazole ring. In the FT-IR spectrum of MNP@P[imEt][OH] (Fig. 1e), characterization peaks of the MNP and IL monomer can be seen.
The morphology of the MNP@ P[imEt][OH] was also investigated by TEM (Fig. 3). TEM analysis displays a dark MNPs core coated by grey polymer shells. Size distribution diagrams of catalyst before and after reaction are shown on Fig. S3. As expected, after recycling of the catalyst, the size of nanoparticles is increased because of aggregation.
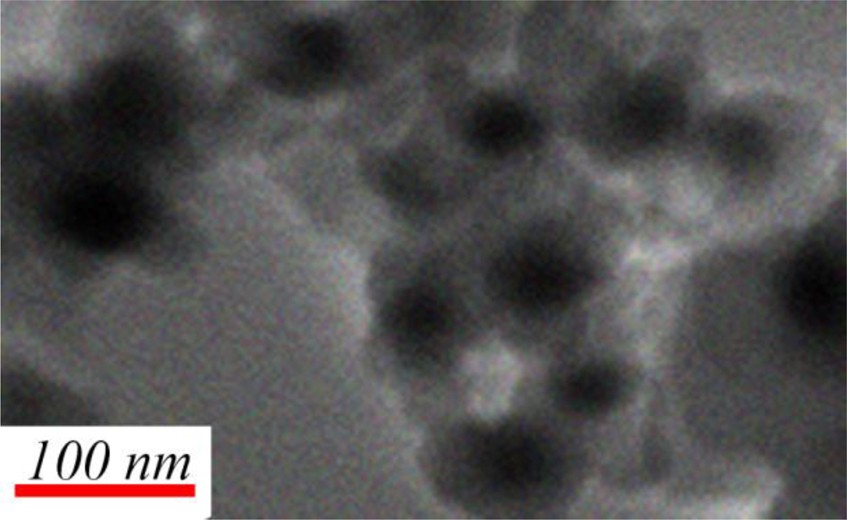
TEM image of MNP@P[imEt][OH].
The XRD pattern of the MNP@P[imEt][OH] shows characteristic peaks and relative intensity, which match well with the standard Fe3O4 sample (JCPDS file No. 19-0629, Fig. 4). The broad peak from 20° to 30° is consistent with an amorphous silica phase in the shell of the Fe3O4@MPS in catalyst (Fig. 4).

XRD pattern of MNP@P[imEt][OH].
The thermal stability of the MNP@P[imEt][OH] was determined by the TGA (Fig. 2c). The TG curve indicates an initial weight loss up to 100 °C, which is due to the adsorbed water on the support. Complete loss of the grafted PIL was seen in the temperature range from 200 to 600 °C, and the amount of organic component was about 51% of the total solid catalyst (Fig. 2).
The magnetic properties of the MNP@P[imEt][OH] and the bare MNP were investigated by VSM at room temperature (Fig. 5). As seen, the saturation magnetization of the sample decreases due to the polymer coating.
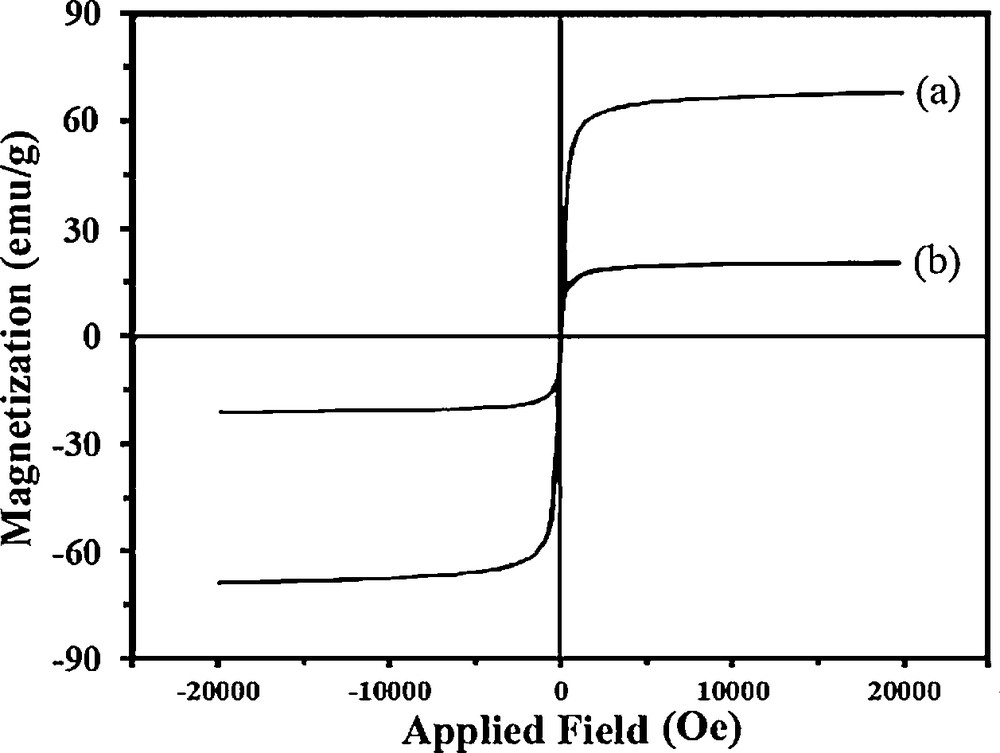
Vibration sample magnetometer curves of bare magnetic nanoparticles (a) and MNP@P[imEt][OH] (b).
The catalytic activity of MNP@P[imEt][OH] was investigated in the three-component reaction of dimedone, aldehydes, and malononitrile (Scheme 3).
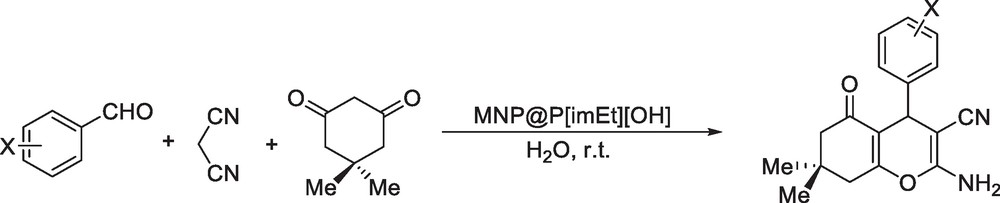
Synthesis of 4H-benzo[b]pyrans catalyzed by MNP@P[imEt][OH] as catalyst.
Table 1 lists the performances of various conditions for optimization of control experiments. As seen in Table 1, in the absence of catalyst, the yield of benzopyrene product was only 13% after 3 h at room temperature. For the sake of comparison between homogenous catalyst and heterogonous ones, the catalytic activity of 1-ethyl-3-vinylimidazolium hydroxide was tested and 95% product was obtained in 20 min at room temperature. The catalytic activity of unexchanged anion of MNP@P[imEt][Br] was also investigated and only 38% yield was obtained after 2 h. The optimum amount of catalyst was found to be 5 mol% at room temperature. The catalyst was used under the same conditions in various solvents and the results are shown in Table 1. Although using ethanol–water mixture as reaction solvent gave good yield, in the view of green chemistry, we chose water.
Optimization of reaction condition for synthesis of 4H-benzo[b]pyransa
Entry | Catalyst | Amount of catalyst (mol%) | Solvent | Time | Yieldb (%) |
1 | None | – | H2O | 3 h | 13 |
2 | EVim/OH | 5 | H2O | 20 min | 95 |
3 | MNP@P[imEt][Br] | 10 | H2O | 2 h | 38 |
4 | MNP@P[imEt][OH] | 10 | H2O | 20 min | 95 |
5 | MNP@P[imEt][OH] | 5 | H2O | 20 min | 92 |
6 | MNP@P[imEt][OH] | 2 | H2O | 30 min | 73 |
7 | MNP@P[imEt][OH] | 5 | H2O–Ethanol | 20 min | 96 |
8 | MNP@P[imEt][OH] | 5 | CHCl3 | 20 min | 73 |
9 | MNP@P[imEt][OH] | 5 | CH3CN | 20 min | 90 |
10 | MNP@P[imEt][OH] | 5 | EtOH | 20 min | 87 |
a Reaction condition Benzaldehyde (1 mmol), malononitrile (1.2 mmol) and dimedone (1 mmol), solvent volume (5 mL) at room temperature.
b Isolated yield.
Subsequently, we investigated the generality of the MNP@P[imEt][OH] in the synthesis of 4H-benzo[b]pyrans by the reaction of various aldehydes with malononitrile and dimedone. As seen in Table 2, various aldehydes including hindered aldehydes and highly deactivated aldehydes were also reacted to give excellent yields. In a scale-up experiment, we examined the reaction between benzaldehyde, malononitrile and dimedon as a model reaction under same conditions in 10 mmol scale. The yield of scale-up reaction was 88% after recrystallization. This demonstrated that catalyst performance in a scale-up process is as good as in a 1 mmol scale reaction.
Synthesis of 4H-benzo[b]pyrans by MNP@P[imEt][OH].
Entry | R–CHO | Time (min) | Yielda (%) |
1 | Ph | 20 | 92 |
2 | 4-(Cl)Ph | 20 | 97 |
3 | 2,4-di(Cl)Ph | 20 | 90 |
4 | 4-(Me)Ph | 25 | 92 |
5 | 4-(HO)Ph | 30 | 88 |
6 | 2-(HO)Ph | 40 | 84 |
7 | 4-(MeO)Ph | 25 | 90 |
8 | 2-(MeO)Ph | 40 | 81 |
9 | 4-(NO2)Ph | 20 | 97 |
10 | 3-(NO2)Ph | 15 | 95 |
11 | 2-(NO2)Ph | 25 | 90 |
12 | 4-(NMe2)Ph | 20 | 93 |
13 | 4-(CN)Ph | 20 | 94 |
14 | Vanillin | 35 | 89 |
15 | Furfural | 15 | 92 |
16 | 4-(CHO)Ph | 45 | 87 |
17 | PhCHCH– | 25 | 79 |
a Reaction condition Aldehyde (1 mmol), malononitrile (1.2 mmol), dimedone (1 mmol), MNP@P[imEt][OH] (5 mol%), H2O (5 mL), room temperature, isolated yield after recrystallization.
Recycling experiments were performed by choosing the reaction between benzaldehyde, malononitrile and dimedon as a model reaction. In the recycling experiments (Fig. S1), the yield of products is still higher than 90% after five cycles. FT-IR of the recovered catalyst shows no change in the structure of the catalyst in comparison with the fresh one (Fig. S2). Also, analysis of the crude product by inductively coupled plasma (ICP) shows no significant metals, which demonstrates low leaching amount of catalyst. This data show that the catalyst is stable under the reaction conditions.
4 Conclusion
In conclusion, we developed a novel green heterogeneous basic IL catalyst for the first time and used it in the synthesis of 4H-benzo[b]pyrans in excellent yields. Because of polymer coating, loading and recyclability of catalyst was improved. Moreover, the catalyst could be readily separated in a green way by using a magnetic force and reused five times without any significant loss of catalytic activity.