1 Introduction
Allylation of carbonyl compounds, using allylic metal reagents, is an important class of carbon–carbon bond-forming reactions, as it gives rise to synthetically useful homoallylic alcohols [1]. The homoallylic alcohol products are often obtained with high stereoselectivity and can further be manipulated to access synthetically valuable intermediates, such as β-hydroxy carbonyl compounds. These compounds can be easily converted into various building blocks useful for the synthesis of natural-like products [2]. A number of methods have been developed for allylation reaction involving various metals, such as zinc [3], tin [4], indium [5], bismuth [6], manganese [7], magnesium [8], antimony [9], scandium [10], cobalt [11], cerium [12], and silver [7b]. Masuyama et al. [13] developed a new approach for synthesizing homoallyl alcohol in one-pot via an umpolung of π-palladium complexes, using SnCl2 as a reducing reagent. Allylation of carbonyl compounds from allylic acetate requires the use of palladium as a catalyst, in addition to another metal or a reducing salt [14]. Some other methods, such as ultrasonic irradiation [15], the use of ionic liquids [16] have also been developed to promote this particular reaction. Nokami et al. first reported the coupling reaction of allyl bromide with carbonyl compounds in aqueous medium mediated by tin and aluminum in the presence of an acid, such as HBr or acetic acid [17]. Gambaro et al. [18] reported a method for the synthesis of allylbromodichlorotin from allyl bromide and tin(II) chloride for allylation of acetone in tetrahydrofuran. However, this method was studied for acetone only, where the corresponding homoallyl alcohol was obtained in low yield (30%) after 24 h of reaction under reflux temperature. In the same year, Mukaiyama et al. [19] reported that allylation of ketones can be achieved using allyl iodide at room temperature in 1,2-dimethylimidazole (DMI) as the solvent. However, this method uses expensive solvent as well as the allylating agent. Moreover, DMI is highly toxic. Very recently, Cuerva et al. [20] developed a new Barbier-type [1c] allylation reaction of carbonyl compounds, using allylic carboxylates in the presence of a Ti/Ni-based multi-metallic catalyst. This procedure requires high amounts of toxic chlorotrimethylsilane (TMSCl), Mn dust and 2,4,6-collidine as the activator and four equivalents of the allylating agent.
Copper [21] and palladium [22] compounds have attracted the attention as catalysts in numerous C–C bond-forming reactions. To our knowledge, there are only a very few reports of SnCl2-mediated, one-pot, Barbier-type allylations using an allyl halide, where Pd(II) [23] or Pd(0) [24] compounds were used as catalysts. But, in both cases, either a high amount of the catalyst or long reaction time is necessary to achieve good yield of homoallyl alcohols. Guo et al. reported a method for the allylation of carbonyl compound using copper powder [25]. This method requires two molar equivalents of copper powder to accomplish the allylation. As a continuation of our investigation on various allylation reactions [26], we present, herein, the results of a systematic study of Barbier-type allylation of carbonyl compounds under the influence of SnCl2·2H2O in the presence of CuI and Pd(OAc)2 as the catalyst (Scheme 1). While no detailed investigations on the catalytic activity of both catalysts for Barbier-type allylation reaction have been reported so far, such study may be useful in developing an effective protocol for various homoallylation reactions.
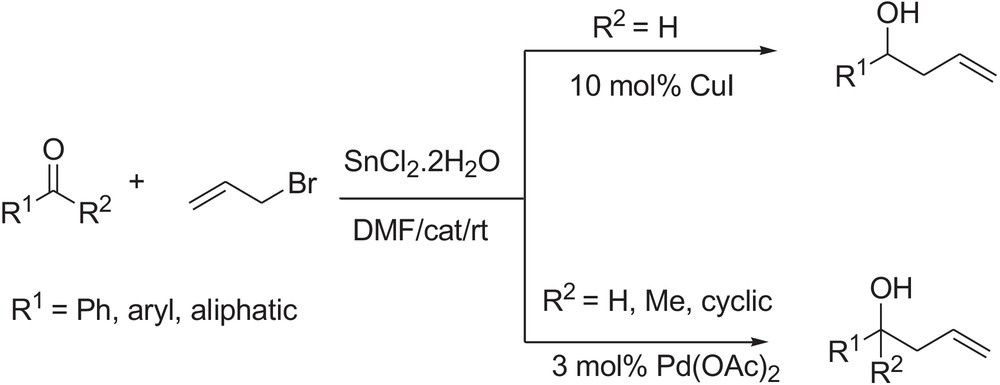
2 Results and discussion
The aim of this study is to evaluate the catalytic efficiency of CuI and Pd(OAc)2 for Barbier-type allylation of carbonyl compounds. In a typical reaction, SnCl2·2H2O (1 mmol) and CuI (5 mol%) were added to a mixture of allylbromide (1 mmol) and benzaldehyde (1 mmol) in N,N-dimethylformamide (DMF) (1 mL) at room temperature. The reaction was monitored by thin-layer chromatography (TLC). The results are presented in Table 1.
Synthesis of homoallylic alcohols from benzaldehydes using CuI as a catalyst.
Entry | Benzaldehyde (mmol) | Solvent | Allylbromide (mmol) | SnCl2·2H2O (mmol) | Catalyst (mmol) | Time (h) | Yielda (%) |
1 | 1 | DMF | 1 | 1 | – | 24 | 0 |
2 | 1 | DMF | 1 | – | CuI (0.05) | 24 | 0 |
3 | 1 | DMF | 1 | 1 | CuI (0.05) | 10 | 50 |
4 | 1 | DMF | 1 | 1 | CuI (0.10) | 10 | 60 |
5 | 1 | DMF | 1.1 | 1 | CuI (0.10) | 10 | 65 |
6 | 1 | DMF | 1.1 | 1.5 | CuI (0.10) | 10 | 72 |
7 | 1 | DMF | 1 | 2 | CuI (0.10) | 3 | 86 |
8 | 1 | DMF | 1.1 | 2 | CuI (0.10) | 3 | 90 |
9 | 1 | DMF | 1.1 | 2 | CuI (0.15) | 3 | 90 |
10 | 1 | CH3CN | 1.1 | 2 | CuI (0.10) | 24 | 22 |
11 | 1 | H2O | 1.1 | 2 | CuI (0.10) | 24 | 0 |
12 | 1 | CH2Cl2 | 1.1 | 2 | CuI (0.10) | 24 | 10 |
13 | 1 | Toluene | 1.1 | 2 | CuI (0.10) | 24 | 0 |
14 | 1 | DMSO | 1.1 | 2 | CuI (0.10) | 24 | 0 |
15 | 1 | DMF | 1.1 | 2 | Pd(OAc)2 (0.02) | 3 | 82 |
16 | 1 | DMF | 1.1 | 2 | Pd(OAc)2 (0.03) | 1 | 92 |
17 | 1 | DMF | 1.1 | 2 | Pd(OAc)2 (0.05) | 0.5 | 91 |
a Isolated yield after chromatographic purification.
Initial experiment, without CuI, failed to produce any product. Another experiment without SnCl2·2H2O also produced a negative result after 24 h of reaction at room temperature. Next, we examined the reaction using 10 mol% of CuI, which could improve the yield up to 60% after 10 h of reaction (Table 1, entry 4). The reaction with a larger amount of allyl bromide could not improve the yield significantly. However, the reaction rate as well as the yield of the product increased significantly, when the concentration of SnCl2·2H2O was doubled. In this case, the reaction produced the corresponding homoallylic alcohol in 86% yield just after 3 h of reaction. An improvement in the yield up to 90% was observed when the amount of allylating agent was increased from 1 to 1.1 equiv (Table 1, entry 8). Further increase of catalyst concentration upto 15 mol% could not improve the result. The reaction was also examined using different solvents, such as H2O, dimethyl sulfoxide (DMSO), toluene, DMF, CH2Cl2, and CH3CN. While the use of CH3CN and CH2Cl2 produced low yield, the reaction was found inactive in water, DMSO, and toluene. However, DMF was found to be the solvent of choice. Finally, the use of 10 mol% of CuI, 1.1 equiv of allyl bromide and 2 equiv of SnCl2·2H2O was found to be optimum to realize the best yield of the product. Next, we examined the catalytic efficiency of Pd(OAc)2 under the same reaction conditions. Initial experiment, using 2 mol% of Pd(OAc)2 as a catalyst produced 82% of the product in 3 h of reaction. Thereafter, we studied the reaction using 3 mol% and 5 mol% of the catalyst. Finally, the use of 3 mol% of Pd(OAc)2 was found to be the optimum in terms of yield and reaction time.
After finding the optimum conditions, the procedure was extended to a variety of aldehydes. The results are summarized in Table 2. In general, this procedure works well for a variety of aromatic as well as aliphatic aldehydes to produce the corresponding homoallylic alcohols in excellent yield. Aromatic substrates, bearing –Cl, –Br, –CH3, –OMe and aliphatic aldehydes reacted very successfully, irrespective of the substituent position in the aromatic ring. The presence of –NO2 and –F groups on the aromatic ring in both aldehydes and ketones not only lowers the yield, but also takes long time.
SnCl2·2H2O-mediated and CuI or Pd(OAc)2-catalyzed allylation of aldehydesa.
Entry | Carbonyl compound | Productb | Catalyst | Time (h) | Yield (%)c |
1 | CuI | 3 | 90 | ||
Pd(OAc)2 | 1 | 92 | |||
2 | CuI | 3 | 92 | ||
Pd(OAc)2 | 1.5 | 91 | |||
3 | CuI | 3 | 92 | ||
Pd(OAc)2 | 1.2 | 93 | |||
4 | CuI | 3 | 90 | ||
Pd(OAc)2 | 1.5 | 92 | |||
5 | CuI | 3 | 85 | ||
Pd(OAc)2 | 1.5 | 88 | |||
6 | CuI | 3.5 | 80 | ||
Pd(OAc)2 | 2 | 83 | |||
7 | CuI | 4 | 78 | ||
Pd(OAc)2 | 2 | 82 | |||
8 | CuI | 10 | 65 | ||
Pd(OAc)2 | 4 | 79 | |||
9 | CuI | 9 | 64 | ||
Pd(OAc)2 | 4 | 74 | |||
10 | CuI | 2 | 95 | ||
Pd(OAc)2 | 1.2 | 94 | |||
11 | CuI | 7 | 77 | ||
Pd(OAc)2 | 3.5 | 80 | |||
12 | CuI | 3 | 82 | ||
Pd(OAc)2 | 1.5 | 84 | |||
13 | CuI | 2.5 | 87 | ||
Pd(OAc)2 | 1.3 | 85 | |||
14 | CuI | 3 | 83 | ||
Pd(OAc)2 | 1.5 | 88 | |||
15 | CuI | 3.5 | 78 | ||
Pd(OAc)2 | 1.5 | 75 | |||
16 | CuI | 5 | 88 | ||
Pd(OAc)2 | 3 | 82 | |||
17 | CuI | 5 | 88 | ||
Pd(OAc)2 | 1.5 | 80 | |||
18 | CuI | 4.5 | 92 | ||
Pd(OAc)2 | 2 | 85 | |||
19 | CuI | 5 | 88 | ||
Pd(OAc)2 | 2.5 | 83 |
a Reaction condition: aldehydes, 1 mmol; allylbromide, 1.1 mmol; SnCl2·2H2O, 2 mmol; CuI, 10 mol%; Pd(OAc)2, 3 mol%.
b All products were characterized by IR, 1H-NMR and 13C-NMR.
c Isolated yield after chromatographic purification.
The success of the homoallylation of the aldehydes encouraged us to extend the reaction to ketones (Table 3). The initial reaction with acetophenone using CuI as the catalyst under the same reaction conditions failed to produce the corresponding homoallyl alcohol. However, the use of 3 mol% of Pd(OAc)2 could drive the reaction forward to produce homoallylic alcohols in 85% yield after 3 h of reaction. The reaction was further extended to a variety of ketones and the results are summarized in Table 3.
SnCl2·2H2O-mediated and Pd(OAc)2-catalyzed allylation of ketonesa.
Entry | Carbonyl Compound | Productb | Catalyst | Time (h) | Yield (%)c |
1 | CuI | 12 | 0 | ||
Pd(OAc)2 | 5 | 85 | |||
2 | Pd(OAc)2 | 6 | 77 | ||
3 | Pd(OAc)2 | 5 | 81 | ||
4 | Pd(OAc)2 | 8 | 68 | ||
5 | Pd(OAc)2 | 7 | 79 | ||
6 | Pd(OAc)2 | 8 | 72 | ||
7 | Pd(OAc)2 | 5 | 71 | ||
8 | Pd(OAc)2 | 7 | 69 | ||
9 | Pd(OAc)2 | 6 | 75 | ||
10 | Pd(OAc)2 | 6 | 77 |
a Reaction conditions: ketones, 1 mmol; allylbromide, 1.1 mmol; SnCl2·2H2O, 2 mmol; CuI, 10 mol%; Pd(OAc)2, 3 mol%.
b All products were characterized by IR, 1H-NMR and 13C-NMR.
c Isolated yield after chromatographic purification.
It can be seen from Table 3 that a variety of ketones undergo homoallylation in the presence of 3 mol% of Pd(OAc)2 to give the product in high yield. Literature search revealed that SnCl2-mediated allylation of ketones, aliphatic ketones, in particular, needs long reaction times to achieve good yields [4a,4b,5a,5c,11,13,16,23]. In the present investigation, the reaction completes in much shorter time with high yield. However, we observed that 4-fluoroacetophenone (Table 3, entry 4) and 4-methylcyclohexanone (Table 3, entry 8) produced slightly low yield of the product.
3 Conclusions
In summary, a comparative study on the Barbier-type allylation reactions of aldehyde- and ketone-mediated by SnCl2·2H2O, using CuI and Pd(OAc)2 as the catalyst have been reported. Although both the catalysts are very effective for aldehydes, CuI failed to catalyse the allylation reaction of ketones under this particular reaction condition. In the presence of Pd(OAc)2, allylation of ketones proceeds smoothly. The best results are obtained when the reaction was carried out using 1 equiv of carbonyl compound, 1.1 equiv of allyl bromide and 2 equiv of SnCl2·2H2O in DMF at room temperature.
4 Experimental
4.1 General
All the chemicals used are of analytical grade. IR spectra were recorded by using a Perkin Elmer Spectrum RX I FT-IR spectrometer. 1H and 13C-NMR spectra were obtained in CDCl3 using Bruker 300 MHz instrument.
4.2 Synthetic procedures
To a solution of carbonyl compound (1 mmol) and allylbromide (1.1 mmol) in DMF (1 ml), SnCl2·2H2O (2 mmol) and the catalyst, (CuI, 10 mol% or Pd(OAc)2, 3 mol%) were added successively at room temperature. After stirring the reaction medium for an appropriate time (TLC) at room temperature, water (10 mL) was added and the reaction mixture was extracted with diethylether (3 × 10 mL). The combined organic extract was dried over sodium sulphate, filtered and concentrated. The crude product was purified by flash column chromatography over silica gel (230–400 mesh) using ethylacetate-petroleum ether (5:95) as the eluent.
1-Phenyl-but-3-en-1-ol,4b (3a). IR (υ/cm−1): 3372, 3073, 2907, 1641, 1493, 1445, 1043; 1H-NMR (300 MHz, CDCl3): δ 7.43–7.23 (m, 5H), 5.91–5.72 (m, 1H), 5.24–5.11 (m, 2H), 4.80–4.70 (m, 1H), 2.60–2.45 (m, 2H), 2.00 (s, 1H); 13C-NMR (75 MHz, CDCl3): δ 143.8, 134.4, 128.4, 127.5, 125.8, 118.5, 73.3, 43.8.
1-(3-Chlorophenyl)but-3-en-1-ol,5e (3b). IR (υ/cm−1): 3377, 3075, 2927, 1709, 1641, 1574, 1429, 1196; 1H-NMR (300 MHz, CDCl3): δ 7.31–7.28 (m, 1H), 7.24–7.12 (m, 3H), 5.78–5.65 (m, 1H), 5.14–5.06 (m, 2H), 4.64 (dd, J1 = 4.83 Hz, J2 = 7.88 Hz, 1H), 2.49–2.33 (m, 2H), 1.91 (s, 1H); 13C-NMR (75 MHz, CDCl3): δ 145.9, 134.3, 133.8, 129.6, 127.6, 126.0, 123.9, 119.0, 72.5, 43.8.
1-(2-Chlorophenyl)but-3-en-1-ol,4b, 5e (3c). IR (υ/cm−1): 3419, 3074, 2927, 1709, 1641, 1574, 1429, 1196; 1H-NMR (300 MHz, CDCl3): δ 7.57 (d, J = 7.18 Hz, 1H), 7.48–7.15 (m, 3H), 6.02–5.76 (m, 1H), 5.35–5.07 (m, 3H), 2.76–2.54 (m, 1H), 2.51–2.31 (m, 1H), 2.26 (s, 1H); 13C-NMR (75 MHz, CDCl3): δ 141.1, 134.3, 131.6, 129.4, 128.4, 127.0, 118.8, 69.6, 42.0.
1-(4-Chlorophenyl)but3-en-1-ol,10, 5e (3d). IR (υ/cm−1): 3374, 3077, 2980, 2908, 1642, 1597, 1490, 1411, 1091; 1H-NMR (300 MHz, CDCl3): δ 7.47–7.14 (m, 4H), 5.87–5.61 (m, 1H), 5.25–4.99 (m, 2H), 4.63 (t, J = 6.42 Hz, 1H), 2.75 (s, 1H), 2.58–2.32 (m, 2H); 13C-NMR (75 MHz, CDCl3): δ 142.2, 133.9, 132.9, 128.3, 127.1, 118.4, 72.5, 43.6.
1-(4-Methoxyphenyl)but-3-en-1-ol,5e (3e). IR (υ/cm−1): 3398, 3072, 2932, 1886, 1611, 1512, 1456, 1247, 1036; 1H-NMR (300 MHz, CDCl3): δ 7.20 (d, J = 8.69 Hz, 2H), 6.82 (d, J = 8.69 Hz, 2H), 5.81–5.65 (m, 1H), 5.12–5.00 (m, 2H), 4.56 (t, J = 6.42 Hz, 1H), 3.72 (s, 3H), 3.41 (s, 1H), 2.53–2.34 (m, 2H); 13C-NMR (75 MHz, CDCl3): δ 158.6, 136.1, 134.6, 127.0, 117.4, 113.4, 72.9, 54.9, 43.4.
1-(2-Methoxyphenyl)but-3-en-1-ol,8b (3f). IR (υ/cm−1): 3412, 3072, 2938, 2837, 1640, 1596, 1462, 1240, 1045; 1H-NMR (300 MHz, CDCl3): δ 7.37 (d, J = 7.55 Hz, 1H), 7.31–7.21 (m, 1H), 6.98 (t, J = 7.18 Hz, 1H), 5.97–5.78 (m, 1H), 5.20–5.07 (m, 2H), 5.05–4.94 (m, 1H), 3.83 (s, 3H), 2.98 (s, 1H), 2.66–2.44 (m, 2H); 13C-NMR (75 MHz, CDCl3): δ 156.1, 135.1, 131.7, 128.1, 126.6, 120.5, 117.2, 110.2, 69.2, 55.0, 41.7.
1-(4-Bromophenyl)but-3-en-1-ol,5e (3g). IR (υ/cm−1): 3376, 3076, 2926, 2906, 1642, 1486, 1405, 1062, 1005; 1H-NMR (300 MHz, CDCl3): δ 7.42 (d, J = 8.31 Hz, 2H), 7.14 (d, J = 8.31 Hz, 2H), 5.81–5.61 (m, 1H), 5.21–5.01 (m, 2H), 4.58 (t, J = 6.04 Hz, 1H), 3.18 (s, 1H), 2.40 (t, J = 6.80 Hz, 2H); 13C-NMR (75 MHz, CDCl3): δ 142.7, 133.8, 131.2, 127.5, 121.0, 118.4, 72.5, 43.4.
1-(4-Nitrophenyl)but-3-en-1-ol,5e (3h). IR (υ/cm−1): 3551, 3389, 3055, 2931, 1631, 1523, 1429, 1169, 1048; 1H-NMR (300 MHz, CDCl3): δ 8.13 (d, J = 8.65 Hz, 2H), 7.46 (d, J = 8.39 Hz, 2H), 5.80–5.62 (m, 1H), 5.19–5.05 (m, 2H), 4.84–4.74 (m, 1H), 2.53–2.33 (m, 2H), 2.11 (s, 1H); 13C-NMR (75 MHz, CDCl3): δ 151.5, 147.6, 133.6, 126.9, 124.0, 120.1, 72.5, 44.3.
1-(2-Nitrophenyl)but-3-en-1-ol,11 (3i). IR (υ/cm−1): 3554, 3382, 3053, 2939, 1624, 1540, 1430, 1173, 1043; 1H-NMR (300 MHz, CDCl3): δ 7.95 (d, J = 8.31 Hz, 1H), 7.84 (d, J = 7.55 Hz, 1H), 7.66 (t, J = 7.18 Hz, 1H), 7.44 (t, J = 7.18 Hz, 1H), 6.04–5.80 (m, 1H), 5.42–5.13 (m, 3H), 2.58–2.27 (m, 2H), 1.62 (s, 1H); 13C-NMR (75 MHz, CDCl3): δ 150.1, 139.0, 133.8, 133.3, 128.0, 127.9, 124.3, 119.0, 68.2, 42.7.
1-(4-Methylphenyl)but-3-en-1-ol,4b, 23 (3j). IR (υ/cm−1): 3542, 3381, 3062, 2938, 1633, 1519, 1436, 1164, 1051; 1H-NMR (300 MHz, CDCl3): δ 7.26 (d, J = 7.93 Hz, 2H), 7.19 (d, J = 7.93 Hz, 2H), 5.92–5.71 (m, 1H), 5.27–5.07 (m, 2H), 4.67 (t, J = 6.42 Hz, 1H), 2.84 (s, 1H), 2.58–2.47 (m, 2H), 2.40 (s, 3H); 13C-NMR (75 MHz, CDCl3): δ 140.9, 136.9, 134.6, 128.9, 125.7, 117.8, 73.1, 43.5, 20.9.
1-(4-Fluorophenyl)but-3-en-1-ol,5e (3k). IR (υ/cm−1): 3359, 3078, 2944, 2858,1845, 1640, 1487, 1370; 1H-NMR (300 MHz, CDCl3): δ 7.40–7.17 (m, 2H), 7.13–6.92 (m, 2H), 5.89–5.60 (m, 1H), 5.26–4.99 (m, 2H), 4.74–4.52 (m, 1H), 3.22 (s, 1H), 2.58–2.31 (m, 2H); 13C-NMR (75 MHz, CDCl3): δ 160.2, 139.5, 134.0, 127.3, 118.0, 114.8, 72.6, 43.5.
1-(2,4-Dichlorophenyl)but-3-en-1-ol,5e, 8b (3l). IR (υ/cm−1): 3278, 3080, 2938, 1639,1470,1071, 1045; 1H-NMR (300 MHz, CDCl3): δ 7.42 (d, J = 8.69 Hz, 1H), 7.37–7.17 (m, 2H), 5.93–5.68 (m, 1H), 5.32–4.94 (m, 3H), 2.91 (s, 1H), 2.66–2.44 (m, 1H), 2.41–2.19 (m, 1H); 13C-NMR (75 MHz, CDCl3): δ 139.6, 133.6, 133.2, 131.9, 128.8, 127.8, 127.1, 118.7, 68.9, 41.7.
1-(4-Ethylphenyl)but-3-en-1-ol,5f (3m). IR (υ/cm−1): 3544, 3389, 3067, 2931, 1638, 1519, 1432, 1161, 1055; 1H-NMR (300 MHz, CDCl3): δ 7.30 (d, J = 7.93 Hz, 1H), 7.23 (d, J = 7.93 Hz, 1H) 5.95–5.73 (m, 1H), 5.28–5.09 (m, 2H), 4.68 (t, J = 6.42 Hz, 1H), 3.03 (s, 1H), 2.72 (q, J = 7.5 Hz, 2H), 2.53 (t, J = 6.8 Hz, 2H), 1.42- 1.27 (m, 3H); 13C-NMR (75 MHz, CDCl3): δ 143.1, 141.0, 134.5, 127.6, 125.7, 117.5, 73.1, 43.4, 28.3, 15.4.
1-(3,4-Dimethoxyphenyl)but-3-en-1-ol,7b (3n). IR (υ/cm−1): 3545, 3381, 3069, 2937, 1620, 1509, 1433, 1182, 1055; 1H-NMR (300 MHz, CDCl3): δ 6.92–6.69 (m, 3H), 5.84–5.63 (m, 1H), 5.17–4.97 (m, 2H), 4.67–4.51 (m, 1 H), 3.82 (s, 3H), 3.80 (s, 3H), 2.57 (s, 1H), 2.48–2.39 (m, 2H); 13C-NMR (75 MHz, CDCl3): δ 148.6, 148.0, 136.4, 134.4, 117.8, 110.6, 108.7, 73.0, 55.6, 43.5.
1-(2-Bromophenyl)but-3-en-1-ol,5e, 8b (3o). IR (υ/cm−1): 3297, 3070, 2933, 1640, 1498, 1465, 1433, 1065; 1H-NMR (300 MHz, CDCl3): δ 7.62–7.44 (m, 2H), 7.31 (t, J = 7.2 Hz, 1H), 7.22–7.04 (m, 1H), 6.00–5.70 (m, 1H), 5.31–4.94 (m, 3H), 2.99 (s, 1H), 2.67–2.51(m, 1H), 2.43–2.27 (m, 1H); 13C-NMR (75 MHz, CDCl3): δ 142.6, 134.1, 132.4, 128.6, 127.4, 127.2, 121.6, 118.2, 71.7, 41.8.
1-(Naphthalen-2-yl)but-3-en-1-ol,26f, 12 (3p). IR (υ/cm−1): 3547, 3382, 3069, 2932, 1639, 1511, 1431, 1161, 1056; 1H-NMR (300 MHz, CDCl3): δ 8.21–7.71 (m, 4H), 7.40–6.72 (m, 3H), 6.03–5.71 (m, 1H), 5.34–5.06 (m, 2H), 5.00–4.76 (m, 1H), 3.13 (s, 1H), 2.63 (t, J = 6.42 Hz, 2H); 13C-NMR (75 MHz, CDCl3): δ 141.1, 134.2, 132.9, 127.9, 127.8, 127.5, 125.9, 125.6, 124.4, 117.9, 73.2, 43.3.
Undec-1-en-4-ol,11 (3q). IR (υ/cm−1): 3363, 3074, 2927, 2857, 1828, 1641, 1458, 1377; 1H-NMR (300 MHz, CDCl3): δ 5.96–5.66 (m, 1H), 5.24–5.03 (m, 2H), 3.96–3.73 (m, 1H), 3.17(s, 1H, OH), 2.41–2.14 (m, 2H), 1.55–1.09 (m, 15 H); 13C-NMR (75 MHz, CDCl3): δ 134.9, 117.8, 45.9, 31.6, 31.5, 30.9, 29.8, 29.4, 29.1, 26.1, 22.5, 21.2, 13.9.
1-Phenylhex-5-en-3-ol,10 (3r). IR (υ/cm−1): 3367, 3064, 2931, 1632, 1517, 1432, 1162, 1051; 1H-NMR (300 MHz, CDCl3): δ 7.51–7.12 (m, 5H), 6.00–5.76 (m, 1H), 5.33–5.08 (m, 2H), 3.87–3.64 (m, 1H), 2.99–2.61 (m, 2H), 2.55–2.16 (m, 3H), 2.01–1.71 (m, 2H); 13C-NMR (75 MHz, CDCl3): δ 141.9, 134.5, 128.3, 128.2, 125.6, 117.9, 69.8, 41.8, 38.2, 31.8.
1-Phenyl-hexa-1,5-dien-3-ol,26f (3s). IR (υ/cm−1): 3542, 3381, 3072, 2930, 1638, 1520, 1431, 1156; 1H-NMR (300 MHz, CDCl3) δ: 7.62–7.14 (m, 5H), 6.62 (d, J = 15.86 Hz, 1H), 6.25 (dd, J1 = 6.42 Hz, J2 = 15.86 Hz, 1H), 6.00–5.75 (m, 1H), 5.33–5.06 (m, 2H), 2.59–2.28 (m, 2H), 1.90 (s, 1H); 13C-NMR (75 MHz, CDCl3): 136.6, 134.0, 131.5, 130.4, 128.6, 127.7, 126.5, 118.6, 71.7, 42.0.
1-phenyl-1-methylbut-3-en-1-ol,5a (5a). IR (υ/cm−1): 3440, 3069, 2977, 1641, 1444, 1371, 1068; 1H-NMR (300 MHz, CDCl3): δ 7.47–7.23 (m, 5H), 5.72–5.62 (m, 1H), 5.18–5.12 (m, 2H), 2.71 (dd, J1 = 13.6 Hz, J2 = 6.42 Hz, 1H), 2.55–2.48 (m, 1H), 1.57 (s, 3H, CH3); 13C-NMR (75 MHz, CDCl3): δ 147.5, 133.5, 128.0, 126.6, 125.0, 119.3, 73.5, 48.3, 29.7.
1-(o-Hydroxyphenyl)-1-methylbut-3-en-ol,5a (5b). IR (υ/cm−1): 3439, 3072, 2975, 1645, 1441, 1377, 1071; 1H-NMR (300 MHz, CDCl3): δ 9.26 (s, 1H), 7.19–6.81 (m, 4H), 5.83–5.75 (m, 1H), 5.22–5.17 (m, 2H), 2.84–2.77 (m, 1H,), 2.59–2.52 (m, 1H,), 1.63 (s, 3H, CH3); 13C-NMR (75 MHz, CDCl3): δ 155.7, 132.9, 129.3, 128.8, 125.9, 120.3, 119.5, 117.5, 76.6, 46.5, 28.3.
1-(p-Methylphenyl)-1-methylbut-3-en-1-ol,5a, 5c (5c). IR (υ/cm−1): 3441, 3064, 2977, 1641, 1444, 1373, 1062; 1H-NMR (300 MHz, CDCl3): δ 7.37 (d, J = 7.93 Hz, 2H), 7.19 (d, J = 7.55 Hz, 2H), 5.71–5.62 (m, 1H), 5.19–5.13 (m, 2H), 2.71 (dd, J1 = 13.6 Hz, J2 = 6.42 Hz, 1H), 2.52 (dd, J1 = 13.6 Hz, J2 = 8.31 Hz, 1H,), 2.38 (s, 3H, CH3), 1.56 (s, 3H, CH3); 13C-NMR (75 MHz, CDCl3): δ 144.7, 136.2, 133.9, 128.9, 124.8, 119.4, 73.6, 48.5, 29.9, 21.0.
1-(p-Fluorophenyl)-1-methylbut-3-en-ol,5a (5d). IR (υ/cm−1): 3445, 3062, 2971, 1639, 1440, 1372, 1062; 1H-NMR (300 MHz, CDCl3): δ 7.43–7.38 (m, 2H, ArH), 7.12–6.99 (m, 2H, ArH), 5.66–5.57 (m, 1H), 5.16–5.11 (m, 2H), 2.66 (dd, J1 = 13.6 Hz, J2 = 6.42 Hz, 1H), 2.52–2.45 (m, 1H), 1.54 (s, 3H, CH3); 13C-NMR (75 MHz, CDCl3): δ 163.2, 159.9, 143.3, 133.3, 126.4, 119.7, 114.6, 73.3, 48.4, 29.9.
1-Cyclohexylbut-3-en-1-ol,10, 5a, 5c (5e). IR (υ/cm−1): 3362, 3067, 2926, 2856,1828, 1640, 1452, 1376; 1H-NMR (300 MHz, CDCl3): δ 5.95–5.81 (m, 1H), 5.16–5.13 (m, 2H), 2.23–2.20 (m,2H), 1.70–1.38 (m, 10H); 13C-NMR (75 MHz, CDCl3): δ 138.8, 133.7, 122.7, 118.7, 70.9, 46.6, 37.3, 29.7, 25.5, 22.1.
1-Cycloheptylbut-3-en-1-ol,5a (5f). IR (υ/cm−1): 3550, 3068, 2936, 1621, 1505, 1185, 1056; 1H-NMR (300 MHz, CDCl3) δ 5.94–5.80 (m, 1H), 5.13–5.05 (m, 2H), 2.21–2.19 (m, 2H), 1.65–1.54 (m, 12H); 13C-NMR (75 MHz, CDCl3) δ 134.0, 118.5, 74.8, 47.7, 41.4, 30.3, 22.2.
1-Cyclopentylbut-3-en-1-ol,5a (5g). IR (υ/cm−1): 3360, 3072, 2919, 2845, 1826, 1640, 1457, 1376; 1H-NMR (300 MHz, CDCl3): δ 5.94–5.85 (m, 1H), 5.16–5.12 (m, 2H), 2.35–2.32 (m,2H), 1.80–1.61 (m, 8H); 13C-NMR (75 MHz, CDCl3): δ 134.6, 118.6, 81.3, 45.8, 38.4, 23.8.
1-(4-Methylcyclohexyl)but-3-en-1-ol,16, 5e (5h). IR (υ/cm−1): 3381, 3065, 2942, 1621, 1510, 1432, 1181, 1056; 1H-NMR (300 MHz, CDCl3): δ 5.14–5.07 (m, 2H), 2.37–2.34 (m, 2H), 1.38–1.25 (m, 12H); 13C-NMR (75 MHz, CDCl3): δ 133.7, 118.6, 70.2, 48.4, 40.8, 34.7, 32.2, 31.1, 30.2, 22.3, 20.1.
4-Methyl-hept-1-en-4-ol,5a, 16 (5i). IR (υ/cm−1): 3542, 3067, 2932, 1508, 1183, 1056; 1H-NMR (300 MHz, CDCl3): δ 5.87–5.78 (m, 1H), 5.10–5.04 (m, 2H), 2.18 (d, J = 7.18 Hz, 2H), 1.41–0.91 (m, 10H); 13C-NMR (75 MHz, CDCl3): δ 134.2, 118.4, 72.2, 46.3, 44.1, 26.6, 17.2, 17.1.
4-Methyl-oct-1-en-4-ol,5d (5j). IR (υ/cm−1): 3542, 3072, 2940, 1620, 1510, 1431; 1H-NMR (300 MHz, CDCl3): δ 5.84–5.73 (m, 1H), 5.03–4.97 (m, 2H), 2.33 (t, J = 7.18 Hz, 2H), 2.13 (d, J = 7.18 Hz, 2H), 2.04 (s, 1H, OH), 1.51–1.43 (m, 4H), 1.41–1.21 (m, 3H), 1.06–0.95 (m, 3H); 13C-NMR (75 MHz, CDCl3): δ 134.2, 118.1, 72.1, 46.2, 43.4, 41.4, 26.5, 25.9, 23.2, 22.2.
Acknowledgements
Financial support from DST (Grant No. SR/S1/OC-43/2011) is gratefully acknowledged.