1 Introduction
Recently, multi-component reactions (MCRs) have been paid much attention by synthetic organic chemists from all over the world because the building of architecturally complex molecules with diverse ranges of complexity can easily be achieved from available starting materials. In most of the cases, a single product was obtained from three or more different substrates by reacting in a well-defined manner through MCRs. These time-efficient reactions are environmentally benign and atom economic [1,2]. MCRs are cost-effective since the expensive purification processes as well as the protection-deprotection steps are non-existent [3]. The synthesis of heterocycles, using MCRs, is a domain of classical carbonyl condensation chemistry.
One of the important goals in organic chemistry is the development of new methodologies for the synthesis of functionalized biologically active compounds with structural diversity, such as β-amino carbonyl compounds, xanthenes and bis-indolyl methanes from simple and readily available precursor molecules. All of these compounds have various medicinal and pharmaceutical activities [4–8].
Among the various methods for the synthesis of β-amino carbonyl compounds, Mannich-type reaction constitutes a method of choice in many cases due to its versatility. Numerous versions of the Mannich reaction have been developed to overcome the drawbacks associated with the classical Mannich reaction. One of the efficient routes is to conduct a one-pot three-component reaction of an aldehyde, an amine and a ketone with the assistance of ultrasound irradiation [9], organic or mineral acids, like proline [10], p-dodecyl benzene sulphonic acid [11] and some Lewis acids (LA), like FeCl3 [12], Cu(OTf)2 [13], SbCl3 (10%) [14], Bi(OTf)3·4H2O [15], and siloxy serine organocatalysts [16]. Most of these methods, although useful, suffer from drawbacks, such as long reaction time, low yield, the use of toxic solvents, requirement of excess of reagents/catalysts, laborious workup-procedures, and harsh reaction conditions. Therefore, it is important to find more convenient methods for the synthesis of these types of compounds.
Xanthenes and their derivatives have received special attention due to their diverse array of biological activities such as anti-inflammatory, antibacterial and antiviral activities [17–19]. In addition to this, they can be employed as dyes [20], intracellular pH indicators [21], molecular probes in chemical biology [22], and fluorescent materials for the visualization of biomolecules [23]. There are several reports in the literature for the synthesis of 3,3,6,6-tetramethyl-1,8-dioxo-octahydro xanthene derivatives employing aromatic aldehydes and dimedone; these include p-TSA [24,25], sulfamic acid [26], molecular iodine [27,28], heteropoly acid [29,30], silica sulfuric acid [31,32], Amberlyst-15 [33], and wet cyanuric chloride [34]. However, these methods suffer from one or more disadvantages such as a long reaction time, low yield, the use of toxic solvents, requirement of excess of reagents/catalysts, laborious work-up procedures, and harsh reaction conditions. Thus, the development of an environmentally benign methodology for the synthesis of benzoxanthene derivatives is in great demand.
Indoles and their derivatives have long been utilized as antibiotics in the field of pharmaceuticals. Bis-indolyl alkanes and their derivatives are more attractive compounds as the bioactive metabolites of terrestrial and marine origin [35]. The acid-catalyzed reaction of electron-rich heterocyclic compounds with p-dimethylaminobenzaldehyde is known as the Ehrlich test [36] for p-electron excessive heterocycles, such as pyrroles and indoles. The analogous reaction of indoles with other aromatic or aliphatic aldehydes and ketones produces azafulvenium salts, which undergo the further addition with another indole molecule to afford bis-indolyl methanes. Brønsted [37,38] and Lewis acids [39] are both known to promote this reaction. Recently, it was reported that the synthesis of bis-indolyl methanes could be catalyzed by LiClO4 [40], Ln(OTf)3 [41], PPh3·HClO4 [42], In(OTf)3 [43], montmorillonite clay K-10 [44], etc., but the long reaction time (4–10 h) and complicated operation limited their further development. Despite the merits of these methodologies, harsh reaction conditions, toxicity or difficulty in product separation remain concerns. Therefore, the search for new readily available green catalysts for this reaction is still being actively pursued.
The above-mentioned disadvantages of the application of the catalysts in the promotion of the synthesis of β-amino carbonyl compounds, 1,8-dioxo-octahydro xanthenes and bis-indolyl methanes encouraged us to use rice-husk-supported FeCl3 nano-particles as a new, efficient and heterogeneous catalyst for the synthesis of these types of compounds. All the reactions are performed under mild reaction conditions in relatively short reaction times in good to high yields (Scheme 1).

Synthesis of β-amino carbonyl compounds, 1,8-dioxo-octahydro xanthenes and bis-indolyl methanes in the presence of FeCl3–rice husk.
2 Experimental
2.1 Material
The chemicals were purchased from Fluka, Merck, Aldrich and Southern Clay Products Chemical Companies. The yields refer to the isolated products. The products were characterized by their physical constants, comparison with authentic samples and IR and NMR spectroscopy. The purity determination of the substrate and the reaction monitoring were accomplished by TLC on silica gel polygram SILG/UV 254 plates.
2.2 Instrumentation
The IR spectra were run on a PerkinElmer bio-spectrometer and a Bruker Vector 22. The 1H NMR (400 MHz) and 13C NMR (100 MHz) spectra were run on a Bruker AvanceiII-400 spectrometer in CDCl3 using TMS as an internal reference (δ in ppm). Microanalyses were performed on a Perkin-Elmer 240-B microanalyzer. The melting points were recorded on a Büchi B-545 apparatus in open capillary tubes. The characterization of rice husk (RiH) was obtained using scanning electron microscopy (SEM-Philips XL30) with field emission gun and energy-dispersive spectroscopy (EDS). Prior to placing into the microscope, the RiH particles were coated with gold under vacuum (SCD 005 sputter coater, Bal-Tec, Swiss) and examined at an acceleration voltage of 17 kV.
2.3 Preparation of rice husk [45]
The rice samples (named as Hassani) were obtained from Rasht (Guilan Province) in the north of Iran. RiH was obtained from a local mill, washed several times with distilled water to remove any adhering materials and dried at room temperature for 48 h. The dried RiH was smashed and sieved (80–170 mesh size), washed with distilled water and dried at 110 °C for 4 h.
2.4 Preparation of the catalyst (FeCl3–rice husk) [45]
The catalyst was prepared by impregnation of RiH (50 g) with a solution of anhydrous FeCl3 (0.053 mol, 8.62 g) in acetone. The solvent was evaporated under reduced pressure and the catalyst was heated at 120 °C for 8 h to give FeCl3–RiH (58 g).
2.5 Synthesis of β-amino carbonyl compounds: a typical procedure
A mixture of benzaldehyde (1.0 mmol), aniline (1.0 mmol), acetophenone (1.1 mmol), and FeCl3–RiH (0.04 g) was stirred in anhydrous EtOH (3 mL) at room temperature for 8 h. The reaction was monitored by TLC. Upon completion, CH2Cl2 was added and the catalyst was separated by filtration. The solution was concentrated under reduced pressure. The solid residue was washed with hot EtOH and dried to afford the requested product in 95% yield.
2.6 Synthesis of 1,8-dioxo-octahydro xanthenes: a typical procedure
A mixture of rice-husk-supported FeCl3 nano-particles (0.2 g), benzaldehyde (1 mmol) and dimedone (2 mmol) was heated at 100 °C in the absence of the solvent. The progress of the reaction was monitored by TLC. After completion of the reaction (1 h), the reaction mixture was diluted with EtOH (10 mL) and stirred for 15 min. The catalyst was decanted and the crude product was recrystallized with EtOH. The product was found to be pure (91%) and no further purification was necessary.
2.7 Synthesis of bis-indolyl methanes: a typical procedure
A mixture of rice-husk-supported FeCl3 nano-particles (0.15 g), benzaldehyde (1 mmol) and indole and/or 2-methylindole (2 mmol) in EtOH (1 mL) was heated at 80 °C. The progress of the reaction was monitored by TLC. After completion of the reaction, the reaction mixture was diluted with EtOH (10 mL) and stirred for 10 min. The catalyst was decanted and the crude product was recrystallized from EtOH/H2O (3:1 mL). The product was found to be pure (92%) and no further purification was needed.
2.8 Spectral data for new compounds
2.8.1 3-(4-bromophenyl)-1-phenyl-3-(phenylamino)propan-1-one (Table 1, entry 4)
IR (KBr), 3400, 3020, 1662 cm−1; 1H NMR (400 MHz, TMS, CDCl3), 3.48 (m, 2 H), 4.60 (br, 1H), 5.00 (m, 1H), 6.57 (s, 2H), 6.72 (s, 1H), 7.13 (s, 2H), 7.37–7.60 (m, 8 H), 7.93 (s, 2H). 13C NMR (400 MHz, TMS, CDCl3): 46.08, 54.22, 113.87, 118.10, 121.08, 128.20, 128.23, 128.79, 129.18, 131.93, 133.62, 136.55, 142.09, 146.69, 197.90.
Preparation of β-amino carbonyl compounds promoted FeCl3–rice husk.
Entry | Product | Time (h) | Yield (%)a,b | m.p. (°C) (Found) | m.p. (°C) (Lit.) | Reference |
1 | 8 | 95 | 164–166 | 169–171 | [46] | |
2 | 12 | 96 | 100–101 | 119–120 | [46] | |
3 | 14 | 94 | 144–146 | 150–152 | [47] | |
4 | 13 | 95 | 127–129 | – | – | |
5 | 13 | 92 | 180–182 | – | – | |
6 | 8 | 94 | 160–162 | 170–171 | [46] | |
7 | 10 | 96 | 167–168 | 170–171 | [46] | |
8 | 14 | 92 | 185–187 | 184–186 | [46] | |
9 | 13 | 92 | 130–132 | – | – | |
10 | 8 | 91 | 125–127 | 131–132 | [48] | |
11 | 10 | 95 | 145–147 | – | – |
a Isolated yield.
b Products were characterized by their physical constants and comparison with authentic samples [46–48].
2.8.2 3-(4-bromophenylamino)-1,3-diphenylpropan-1-one (Table 1, entry 5)
IR (KBr) 3375, 3010, 1660 cm−1;1H NMR (400 MHz, TMS, CDCl3), 2.43 (s, 3H), 3.36–3.42 (dd, J1 = 8.00 Hz, J2 = 16.00 Hz, 1H), 3.47–3.52 (dd, J1 = 4.40 Hz, J2 = 16.00 Hz, 1H), 4.69 (br, 1H), 4.95 (m, 1H), 6.44–6.460 (d, 8.40 Hz, 2H), 7.17–7.19 (d, 8.40 Hz, 2H), 7.26 (s, 3H), 7.34–7.37 (t, 7.20 Hz, 2H), 7.43–7.44 (d, 7.20 Hz, 2H), 7.82–7.84 (d, 7.60 Hz, 2H), 7.84–7.86 (d, J = 7.60, 2H), 13C NMR (400 MHz, TMS, CDCl3), 21.70, 46.12, 54.93, 109.47, 115.45, 126.28, 127.49, 128.36, 128.92, 129.44, 131.79, 134.14, 142.55, 144.51, 146.06, 197.80.
2.8.3 3-(3-chlorophenylamino)-1,3-diphenylpropan-1-one (Table 1, entry 9)
IR (KBr) 3375, 3005, 1668 cm−1;1H NMR (400 MHz, TMS, CDCl3), 3.42–3.48 (dd, J1 = 7.60 Hz, J2 = 16.20 Hz, 1H), 3.51–3.56 (dd, J1 = 4.80 Hz, J2 = 16.01 Hz, 1H), 4.77 (br, 1H), 4.99–5.02 (dd, J1 = 4.80 Hz, J2 = 7.60 Hz, 1H), 6.58 (s, 1H) 6.64–6.66 (d, 7.60 Hz, 1H), 7.26–7.28 (d, 7.20 Hz, 1H), 7.35–7.39 (t, 7.60 Hz, 2H), 7.44–7.46 (d, 7.60 Hz, 1H), 7.48–7.50 (d, 7.60 Hz, 2H),7.58–7.62 (t, 7.20 Hz, 2H), 7.92–7.94 (d, J = 7.60, 2H),13C NMR (400 MHz, TMS, CDCl3), 46.13, 54.64, 111.96, 113.61, 117.70, 126.29, 127.58, 128.23, 128.77, 128.96, 130.11, 133.58, 134.82, 136.59, 142.36, 148.18, 198.12.
2.8.4 3-(4-bromophenylamino)-3-phenyl-1-p-tolylpropan-1-one (Table 1, entry 11)
IR (KBr) 3380, 3020, 1680 cm−1; 1H NMR (400 MHz, TMS, CDCl3), 3.41–3.47 (dd, J1 = 7.60 Hz, J2 = 16.20 Hz, 1H), 3.50–3.55 (dd, J1 = 4.80 Hz, J2 = 16.20 Hz, 1H), 4.67 (br, 1H), 4.96–5.00 (dd, J1 = 4.80 Hz, J2 = 7.60 Hz, 1H), 6.58 (s, 1H) 6.65–6.67 (d, 8.80 Hz, 2H), 7.17–7.19 (d, 8.80 Hz, 2H), 7.25–7.27 (d, 7.60 Hz, 3H), 7.34–7.37 (t, 7.60 Hz, 2H), 7.42–7.44 (d, 7.20 Hz, 2H),7.60 (t, 7.60 Hz, 1H), 7.92–7.94 (d, J = 7.20, 2H),13C NMR (400 MHz, TMS, CDCl3), 46.20, 54.82, 109.54, 115.45, 126.29, 127.54, 128.21, 128.76, 128.93, 131.81, 133.57, 136.59, 142.43, 146.00, 198.17.
3 Results and discussions
Very recently, we have reported the preparation of the rice-husk-supported FeCl3 nano-particles and its applicability in the promotion of the acylation of aldehydes and deprotection of the obtained 1,1-diacetates [45]. In continuation of this study, we have found that this reagent is also efficiently able to catalyze the synthesis of β-amino carbonyl compounds, 1,8-dioxo-octahydro xanthenes, and bis-indolyl methanes. All the reactions are performed under mild reaction conditions in good to high yields (Table 1).
First of all, we have tried to study the Mannich reaction using FeCl3–RiH as the catalyst. For obtaining the best amounts of the catalyst, an optimization study was performed using the reaction of benzaldehyde, acetophenone and aniline as a model reaction in the presence of varying amounts of the catalyst [FeCl3–RiH] under different conditions, including various solvents (DMF, CH3CN, DCM, H2O, and EtOH) at room temperature. We found that absolute EtOH was the best choice among the solvents. The reaction failed to yield any products in DCM or H2O and the yields in CH3CN and DMF were very poor. The results showed that the reaction using 0.04 g of absolute EtOH as a catalyst at room temperature proceeded with the highest yield.
Furthermore, the Mannich reaction was very sensitive to the reaction temperature. High temperatures could improve the reaction rate and shorten the reaction time, but favor side reactions and the oxygenolysis of aldehyde and amine. It was found that the room temperature was an appropriate condition for the Mannich reaction catalyzed by FeCl3–RiH (Scheme 2).

Mannich reaction catalyzed by FeCl3–rice husk.
After optimization of the reaction conditions and in order to show the generality of the method, we used the optimized conditions for the synthesis of different types of β-amino carbonyl compounds. Our investigations clarified that various aromatic aldehydes and amines bearing different substitutes, such as OMe, Me, Br and Cl, were all suitable for the reactions.
In order to show the merit of the catalyst, we have compared the results obtained from the reaction of acetophenone, benzaldehydes, and aniline in the presence of FeCl3–RiH with some of the other catalysts for the similar reaction (Table 3). LAs, such as [C6H11N(CH2C6H4)2SbOSO2CF3], FeCl3, SbCl3, phenyl phosphonic acid and phenyl phosphonic acid/SiO2 promoted the reaction in 0–65% yields after 15–20 h (Table 3, entries 1, 3–6). Under the same conditions, Cu–silicate and montmorillonite K-10 gave β-amino carbonyl compounds in 40% yield after 20 h and 35% yield after 20 h, respectively (Table 3, entries 2 and 7). However, among the catalysts studied for this reaction, FeCl3–RiH was found to be the most effective one for this transformation, since it resulted in the highest conversion into the desired product (Table 3, entry 8). It is very important to note that the same reaction in the presence of RiH produces the corresponding imine, even after prolonged reaction times (Table 3, entry 9).
Solvent-free synthesis of 1,8-dioxo-octahydro xanthenes in the presence of FeCl3–rice husk.
Entry | Product | Time (h) | Yield (%) a,b | m.p. (°C) (Found) | m.p. (°C) (Lit.) | Reference |
1 | 1 | 91 | 201–202 | 203–205 | [50] | |
2 | 1 | 95 | 232–234 | 230–232 | [50] | |
3 | 1.5 | 94 | 240–241 | 240–241 | [50] | |
4 | 1 | 92 | 220–221 | 223–224 | [50] | |
5 | 1.5 | 91 | 242–244 | 241–243 | [51] | |
6 | 1.5 | 92 | 217–220 | 221–223 | [51] | |
7 | 1.5 | 91 | 190–191 | 190–192 | [51] | |
8 | 1. | 94 | 163–165 | 167–168 | [51] | |
9 | 1.5 | 89 | 165–166 | 162–164 | [52] | |
10 | 1.25 | 91 | 226–229 | 226–228 | [51] |
a Isolated yield.
b Products were characterized by their physical constants and comparison with authentic samples [50–52].
After successful synthesis of a series of β-amino carbonyl compounds, in good yields, we turned our attention toward the synthesis of 3,3,6,6-tetramethyl-1,8-dioxo-octahydro xanthenes by the condensation of aldehydes and 5,5-dimethyl-1,3-cyclohexadione (dimedone) (Table 2) First of all and in an optimized procedure, with respect to the solvent, catalyst concentration, temperature, and time, the condensation of 4-chlorobenzaldehyde (1 mmol), and dimedone (2.1 mmol) was investigated in the presence of FeCl3–RiH as the catalyst. Our investigations clarified that the best result can be obtained in the absence of solvent at 100 °C using 0.2 g of FeCl3–RiH (Scheme 3).
Comparison of the effect of the catalysts on the preparation of 1,3-diphenyl-3-(phenylamino)propan-1-one (a).
Entry | Catalyst | Time (h) | Yield (%)a | Reference |
1 | [C6H11N(CH2C6H4)2SbOSO2CF3] (5 mol%) | 24 | 65 | [49] |
2 | Montmorillonite K10 (10%) | 20 | 35 | [11] |
3 | FeCl3 (10%) | 20 | 0 | [9] |
4 | SbCl3 (10%) | 15 | 60 | [11] |
5 | Phenyl phosphonic acid (10%) | 20 | 40 | [11] |
6 | Phenyl phosphonic acid/SiO2 (10%) | 20 | 45 | [11] |
7 | Cu–silicate (10%) | 20 | 40 | [11] |
8 | FeCl3–RiH | 8 | 95 | This work |
9 | RiH | 20 | 90 (imine) | This work |
a Isolated yields.
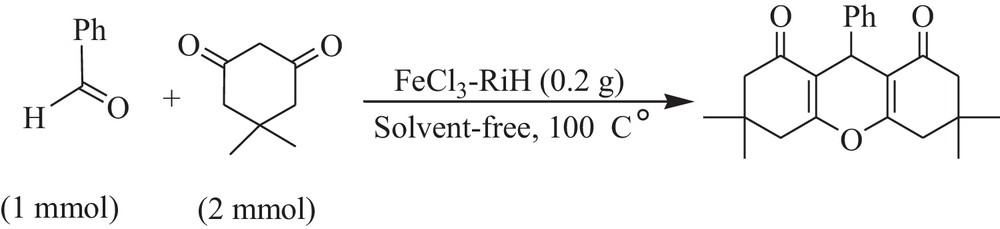
Synthesis of 1,8-dioxo-octahydro xanthenes catalyzed by FeCl3–rice husk.
After optimization of the reaction conditions and in order to show the applicability of the proposed method, different types of aldehydes were subjected to the same reaction and the results are presented in Table 3.
It is noteworthy that the electronic properties of the group on the aldehydes’ aromatic ring have a delicate effect on reaction times. As shown in Table 3, the aromatic aldehydes containing electron-withdrawing groups showed relatively similar reactivity to those containing electron-donating groups.
The advantages of FeCl3–RiH over the reported catalysts for the synthesis of 3,3,6,6-tetramethyl-1,8-dioxo-octahydroxanthene derivative of 4-chlorobenzaldehyde are shown in Table 4. It is noteworthy that some of the previously reported methods require longer reaction times.
Comparison of the results obtained from the synthesis of 1,8-dioxo-octahydro xanthenes from 4-chlorobenzaldehyde and dimedone in the presence of various catalysts.
Entry | Catalyst | Time (h) | Yield (%)a | Reference |
1 | FeCl3·6H2O in [bmim][BF4] at 80 °C | 7 | 93 | [53] |
2 | Cellulose sulfonic acid | 5 | 94 | [54] |
3 | TMSCl | 8 | 74.3 | [55] |
5 | FeCl3–RiH | 1 | 95 | This work |
a Isolated yields.
After this study, we were interested in studying the applicability of FeCl3–RiH in the promotion of the synthesis of bis-indolyl methanes via the condensation of aldehydes with indoles. In this study and in order to optimize the reaction conditions, the condensation of 4-chlorobenzaldehyde and indole was investigated in the presence of FeCl3–RiH. We have found that the best results can be obtained in EtOH at 80 °C using 0.15 g of FeCl3–RiH (Scheme 4).

Synthesis of bis-indolyl methanes catalyzed by FeCl3–rice husk.
Encouraged by this successful result, we studied various aldehydes under optimized conditions to better understand the scope and generality of this simple procedure. A series of aromatic aldehydes underwent an electrophilic substitution reaction with indole and 2-methyl indole to afford a wide range of substituted bis-indolyl methanes, in good to excellent yields. This method is equally effective for aldehydes bearing electron-withdrawing or -donating groups in the aromatic rings (Scheme 5, Table 5).

A plausible mechanism for the synthesis of β-amino carbonyl compounds, 1,8-dioxo-octahydro xanthenes, and bis-indolyl methanes in the presence of FeCl3–rice rusk.
Synthesis of bis-indolyl methanes in the presence of FeCl3–rice husk as the catalyst.
Entry | Product | Time (min) | Yield (%)a,b | m.p. (°C) (Found) | m.p. (°C) (Lit.) | Reference |
1 | 15 | 92 | 144–145 | 149–150 | [56] | |
2 | 10 | 96 | 75–77 | 76–77 | [56] | |
3 | 40 | 92 | 183–185 | 185–187 | [56] | |
4 | 30 | 95 | 222–224 | 217–220 | [56] | |
5 | 45 | 90 | 127–128 | 123–125 | [57] | |
6 | 10 | 94 | 89–91 | 93–94 | [56] | |
7 | 50 | 91 | 219–221 | 221–224 | [58] | |
8 | 40 | 93 | 75–76 | 70–71 | [56] | |
9 | 20 | 95 | 201–203 | 198–202 | [58] | |
10 | 20 | 90 | 101–103 | 98–99 | [56] | |
11 | 14 | 94 | 243–245 | 247–248 | [56] | |
12 | 11 | 97 | 247–248 | 186–188 | [58] | |
13 | 15 | 95 | 248–250 | 240–242 | [59] | |
14 | 30 | 90 | 209–211 | 237–239 | [60] |
a Isolated yield.
b Products were characterized by their physical constants and comparison with authentic samples [56–60].
In addition, this procedure is superior, compared to the literature data, with respect to the catalyst, solvent and eco-friendliness (Table 6).
Comparison of the results obtained from the synthesis of bis-indolyl methane from benzaldehyde and indole in the presence of various catalysts.
Entry | Catalyst | Time (min) | Yield (%)a | Reference |
1 | [bmim]BF4 | 270 | 90 | [61] |
2 | FeCl3·6H2O (5 mol %) in [omim]PF6 | 90 | 98 | [62] |
3 | I2 | 10 | 72 | [63] |
4 | Mg(HSO4)2 | 360 | 89 | [64] |
5 | Silica sulfuric acid | 120 | – | [64] |
6 | FeCl3–RiH | 15 | 92 | This work |
a Isolated yields.
A plausible mechanism for the synthesis of β-amino carbonyl compounds, 1,8-dioxo-octahydro xanthenes and bis-indolyl methanes in the presence of FeCl3–RiH is shown in Scheme 5. Presumably, FeCl3–RiH acts as a LA to catalyze the nucleophilic attack on the carbonyl group of the aldehyde.
4 Conclusions
In summary, we have developed an environmentally friendly protocol, allowing high yields in mild conditions, for the three-component synthesis of β-amino carbonyl compounds 1,8-dioxo-octahydro xanthenes and bis-indolyl methanes catalyzed by FeCl3–RiH. This method offers several advantages compared to those reported in literature:
- • mild and highly efficient catalyst activity;
- • cost efficiency of the catalyst;
- • avoidance of the troublesome preparation of the enol derivatives and of the pre-formed imines;
- • wide substrate scope;
- • ease of product isolation/purification, making it a useful and attractive strategy for the synthesis of the above-mentioned products.
Acknowledgments
We are thankful to the University of Guilan Research Council for the partial support of this work.