1 Introduction
An increasing number of studies have reported on the environmentally friendly production of fine chemicals using recyclable heterogeneous catalysts via multi-component reactions (MCRs). They are considered to be superior synthetic strategies [1–10], with highly efficient atom economy compared to other organic reactions. Their advantages in terms of one-pot multi-component reaction, reaction time, product yield, minimization of waste production, energy consumption and reproducibility have been repeatedly exploited in various efficient syntheses of heterocyclic compounds.
Due to numerous important biological activities as versatile building blocks for the synthesis of natural products and as drug molecules [11–15], the pyrazolo[1,2-b]phthalazine-dione and their derivatives are important targets in synthetic organic chemistry. In particular, pyrazolo[1,2-b]phthalazine-diones are biologically active and occur in structures of a number of anti-inflammatory, anti-allergic, analgesic antihypoxic, anticonvulsant, cardiotonic, vasorelaxant, antipyretic anti-hyperglycemic, anti-bacterial, and anti-viral compounds [16–18]. This core also has been utilized in diverse pharmaceutical applications, such as in anti-cancer [19] and anti-diabetes [20] agents.
So far, only a few methods have been reported for the preparation of 1H-pyrazolo[1,2-b]phthalazine-5,10-dione carbonitriles and carboxylates [21]. However, the above-mentioned catalysts have one or more disadvantages, such as long reaction time, high reaction temperature, use of volatile and hazardous organic solvents, occurrence of side products, and they were reported in homogenous catalysts. Even though Pandurangan et al. used heterogeneous catalysts, they reported they worked in solvents, with long reaction times [21a]. The use of homogenous catalysts has received little attention as alternatives to alleviating some of the limitations. Solid acids as heterogeneous catalysts have received tremendous attention in different areas of organic synthesis [22]. Heterogeneous solid acids are advantageous over conventional homogeneous acid catalysts as they can be easily recovered from the reaction mixture by simple filtration and can be reused after with or without activation, thereby making the process economically more viable. Among them, silica gel-supported tungstic acid (STA) is a well-known and widely used solid acid catalyst in synthetic organic chemistry. It has received considerable attention due to its non-toxicity, cost effectiveness, air and water compatibility, ease of handling, good reactivity, recyclability, experimental simplicity, remarkable ability as a green catalyst to suppress side reactions in acid-sensitive substrates and as supports for a wide variety of reactions. Despite its great importance, only a few publications are reported on its catalytic application in organic synthesis [23]. It is evident from recent literature that the solvent-free endorsed STA reactions are well known as environmentally benign methods that also usually provide improved selectively, enhanced reaction rates, cleaner products, and manipulative simplicity [23a,b].
In continuation of our efforts to develop new green methodologies and as well as our interest in applications of heterogeneous-catalyzed organic reactions [24], we reported herein STA as a new heterogeneous catalytic system for MCR. The STA catalytic activity was investigated for the one-pot synthesis of 1H-pyrazolo[1,2-b]phthalazine-5,10-dione carbonitriles and carboxylates through a three-component condensation reaction of phthalhydrazide, aldehydes and malononitrile/ethyl cyanoacetate in solvent-free conditions at 70 °C (Scheme 1).
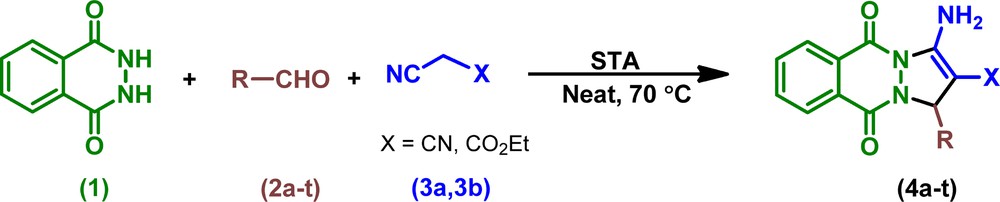
Neat synthesis of 1H-pyrazolo[1,2-b]phthalazine-5,10-dione carbonitriles and carboxylates catalyzed by STA.
2 Results and discussion
For our initial investigation, the reaction of phthalhydrazide (1), 2,3-dihydrobenzo[b][1,4]dioxine-6-carbaldehyde (2a), and malononitril (3a) under neat conditions was chosen as a model reaction. At 70 °C and in the absence of catalyst, we could not isolate any desired MCR product, even after 6 h of stirring. After 10 h of stirring, a trace amount of the corresponding product was obtained (Table 1, entry 1). Next, the same set of reactions was performed in the presence of 5 mol% of PS/PTSA. Within 4 h, the corresponding title product 4a was isolated in 40% product yield (Table 1, entry 2). The product 4a was confirmed by usual spectroscopic techniques. Encouraged by this result, we attempted to optimize the yield of the reaction by screening the same set of reactions with various heterogeneous catalysts, such as BF3–SiO2, CAN–SiO2, TiO2–SiO2 and STA, and the results are summarized in Table 1. Among all the screened catalysts, STA was found superior with respect to reaction time and product yield (Table 1, entry 6). Moreover, we found that the yields were obviously affected by the amount of loaded STA. When 1 mol%, 2 mol%, 5 mol% and 10 mol% of STA were used, the yields were 58, 70, 93, and 94%, respectively (Table 1, entries 6–9). Therefore, 5 mol% of STA was sufficient and no more significant improvement in the reaction rate and product yield was observed while increasing the amount of the catalyst from 5 to 10 mol% (Table 1, entry 9).
Influence of the catalyst for the synthesis of 3-amino-1-(2,3-dihydrobenzo[b][1,4]dioxin-6-yl)-5,10-dioxo-5,10-dihydro-1H-pyrazolo[1,2-b]phthalazine-2-carbonitrile 4aa.
Entry | Catalyst (mol%) | Time (min) | Yield (%)b |
1 | Neat | 600 | Trace |
2 | PT/PTSA (5 mol%) | 240 | 40 |
3 | BF3–SiO2 (5 mol%) | 170 | 60 |
4 | CAN–SiO2 (5 mol%) | 110 | 75 |
5 | TiO2–SiO2 (5 mol%) | 130 | 66 |
6c | STA (5 mol%) | 20 | 93, 92, 90, 89 |
7 | STA (1 mol%) | 100 | 58 |
8 | STA (2 mol%) | 55 | 70 |
9 | STA (10 mol%) | 20 | 94 |
a Reaction of 2,3-dihydrobenzo[b][1,4]dioxine-6-carbaldehyde (2a, 1 mmol), phthalhydrazide (1, 1 mmol) and malononitrile (3a, 1 mmol) under neat at 70 °C.
b Isolated yield.
c The catalyst was reused four times.
Then, we investigated the influence of various organic solvents at different reaction temperatures on the model reaction with 5 mol% of STA with and without catalyst. Among various solvents, such as toluene, THF, CH2Cl2, CH3CN, and DMF, the reaction rate is very slow and resulted in lower product yields (Table 2, entries 1–6). Conducting the same reaction in ethanol improved both the reaction rate and the product yield (Table 2, entry 7). However, the better product yield was observed in solvent-free conditions (Table 2, entry 8) and could be explained by a uniform distribution of the eutectic mixture of reactants, which are in closer proximity to react. It was concluded that irrespective of the nature of solvent, even at its boiling temperature, the optimum conversion rate of the reactants was always observed, whereas under solvent-free conditions, the maximum conversion rate of the reactants was only achieved at 70 °C.
Optimal reaction conditions and temperatures for different solvents in the synthesis of 3-amino-1-(2,3-dihydrobenzo[b][1,4]dioxin-6-yl)-5,10-dioxo-5,10-dihydro-1H-pyrazolo[1,2-b]phthalazine-2-carbonitrile 4aa.
Entry | Solvent | Temp (°C) | Yield (%)b | |
Catalyst-free conditions | STA (5 mol%) | |||
1 | Toluene | 110 | 33.4 | 52.6 |
2 | Chlorobenzene | 120 | 32.2 | 48.7 |
3 | CH2Cl2 | 75 | 34.1 | 48.4 |
4 | CCl4 | 75 | 30.4 | 45.1 |
5 | THF | 65 | 43.6 | 50.8 |
6 | Acetonitrile | 65 | 48.8 | 61.4 |
7 | Ethanol | 70 | 57.4 | 80.4 |
8 | No solvent | 70 | 20.5 | 93.0 |
a Reaction conditions: 3-dihydrobenzo[b][1,4]dioxine-6-carbaldehyde (2a, 1 mmol), phthalhydrazide (1, 1 mmol), and malononitrile (3a, 1 mmol). Reaction times: 20 min.
b Isolated yield.
Solvent and temperature variations have also been tested on the model reaction using 5 mol% of STA in different solvents at their boiling temperatures for 20 min of reaction (Table 2, entries 1–7). Irrespective of the nature of the solvent, even at its boiling temperature, we always observed an optimum conversion rate of the products (Fig. 1), but under solvent-free conditions, the maximum conversion yield was achieved (Table 2, entry 8). The lower yields in solvent medium may be due to salvation of reaction medium.
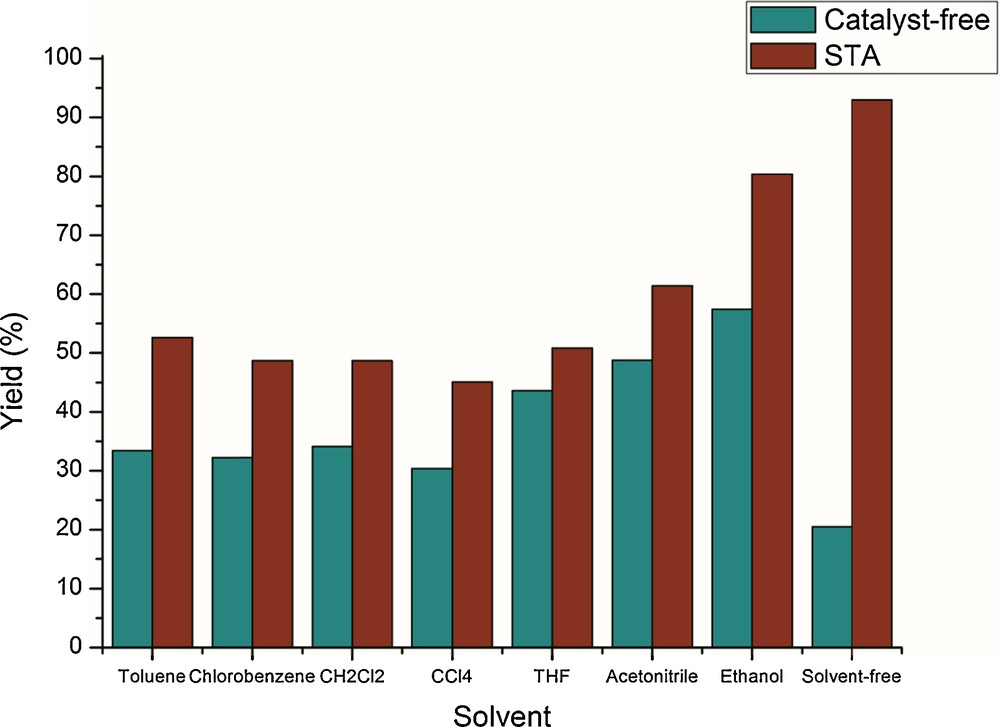
(Color online.) Product yields at various solvents on model reaction.
In order to investigate the catalytic activity and the possibility of catalyst recyclability and reusability, the STA was recovered from the reaction mixture by simple filtration in hot EtOH. The separated catalyst was dried in a vacuum oven at 100 °C and was reused as such for subsequent experiments under similar reaction conditions. The results showed that the catalyst could be effectively reused for at least four consecutive cycles without much appreciable loss in its catalytic activity (Table 1, entry 6). The recyclability data demonstrate a high stability of the catalyst under the reaction conditions.
Having optimized the reaction conditions for the synthesis of 1H-pyrazolo[1,2-b]phthalazine-5,10-dione carbonitriles and carboxylates using STA as the catalyst under solvent-free conditions, we subsequently applied the optimized reaction conditions to various aldehydes to explore the generality of the reaction system. As shown in Table 3, multi-component reactions produced excellent product yields for a wide range of aromatic aldehydes bearing both electron-donating and electron-withdrawing substituents. Non-aromatic and heteroaromatic aldehydes, however, were less reactive, producing only moderate yields (Table 3). In a similar manner to what was observed with malononitrile, ethyl cyanoacetate also participated in this multi-component reaction to afford the corresponding 1H-pyrazolo[1,2-b]phthalazine-5,10-dione carbonitriles and carboxylates in good yields. In all cases, the pure product was isolated by simple filtration, without chromatography or a cumbersome work-up procedure. After the reaction, the catalyst can be easily separated from the product and reused without any significant decrease in its catalytic activity. All new structures were characterized by 1H NMR, 13C NMR, and HRMS. Data and melting point of compounds 4f–j and 4p–t coincide with those of the reported ones.
STA-catalyzed multi-component synthesis of 1H-pyrazolo[1,2-b]phthalazine-5,10-dione carbonitriles and carboxylates 4a–t.
Compound | R | X | Yield (%)a | Time (min) | Mp (°C) | |
Found | Reported | |||||
4a | CN | 93 | 20 | 261–263 | ||
4b | CN | 90 | 25 | 259–261 | ||
4c | 2-Cl–6-F–C6H3 | CN | 91 | 30 | 266–268 | |
4d | 3,5-F–C6H3 | CN | 92 | 26 | 272–274 | |
4e | 2-F–4-Br–C6H3 | CN | 91 | 28 | 252–254 | |
4f | 3-F–C6H4 | CN | 93 | 20 | 263–265 | 264–266 [21f] |
4g | 3-F–4-Me-C6H3 | CN | 90 | 26 | 260–262 | 261–263 [21f] |
4h | C2H5 | CN | 88 | 30 | 240–242 | 238–240 [21f] |
4i | C3H7 | CN | 89 | 28 | 223–225 | 220–222 [21f] |
4j | 2-thiophene (C4H3S) | CN | 85 | 32 | 245–247 | 244–246 [21f] |
4k | CO2Et | 91 | 24 | 220–222 | ||
4l | CO2Et | 88 | 30 | 211–213 | ||
4m | 2-Cl–6-F–C6H3 | CO2Et | 88 | 31 | 217–219 | |
4n | 3,5-F–C6H3 | CO2Et | 90 | 26 | 246–248 | |
4o | 2-F–4-Br–C6H3 | CO2Et | 89 | 32 | 215–217 | |
4p | 3-F–C6H4 | CO2Et | 92 | 22 | 228–230 | 228–230 [21f] |
4q | 3-F–4-Me–C6H3 | CO2Et | 90 | 30 | 220–222 | 222–224 [21f] |
4r | C2H5 | CO2Et | 87 | 35 | 167–169 | 165–167 [21f] |
4s | C3H7 | CO2Et | 89 | 32 | 151–153 | 150–152 [21f] |
4t | 2-thiophene (C4H3S) | CO2Et | 90 | 35 | 228–230 | 228–230 [21f] |
a Isolated yield.
The role of STA as a catalyst in the synthesis of the title compounds (4a–t) should be postulated as shown in Scheme 2. The multi-component reaction should be proceeding in a stepwise manner. First, the reaction occurs via a Knoevenagel condensation between the enolic form of malononitrile (3) and aldehyde (2) in the presence of acidic STA as a catalyst to form intermediate 5. Here, the solid catalyst can facilitate active hosting sites for reactant molecules and accelerate the reaction rate. Second, intermediate 5 immediately transforms into one more intermediate, 6, by Michael addition of 2,3-dihydrophthalazine-1,4-dione (1) at the conjugated CC bond of 5. Finally, by intramolecular concerted cyclisation of the adduct, 6 gave the title compounds (4a–t) in good yields.

(Color online.) Schematic presentation of the postulated mechanistic activity of our STA catalyst.
3 Conclusion
In conclusion, we have explored the use of the heterogeneous STA catalyst as an effective and reusable catalyst for the synthesis of 1H-pyrazolo[1,2-b]phthalazine-5,10-dione carbonitriles and carboxylates. The use of an environmentally friendly and inexpensive catalyst and solvent-free conditions, along with the reusability of the catalyst, provides a good example of a competitive alternative synthetic methodology for these compounds.
4 Experimental
4.1 General
Chemicals were purchased from Aldrich and Alfa Aesar Chemical Companies. NMR spectra were recorded in ppm in DMSO-d6 on a Jeol JNM ECP 400 NMR instrument using TMS as an internal standard. Mass spectra were recorded on a Jeol JMS-700 mass spectrometer. All melting points were determined using open capillaries on an Electrothermal-9100 (Japan) instrument.
4.2 General procedure for the synthesis of 3-amino-1-(2,3-dihydrobenzo[b][1,4]dioxin-6-yl)-5,10-dioxo-5,10-dihydro-1H-pyrazolo[1,2-b]phthalazine-2-carbonitrile (4a)
A mixture of phthalhydrazide (1, 1 mmol, 162 mg), 2,3-dihydrobenzo[b][1,4]dioxine-6-carbaldehyde (2a, 1 mmol, 164 mg), malononitrile (3a, 1 mmol, 66 mg) and STA (5 mol%, 21 mg) was stirred at 70 °C under solvent-free conditions for 20 min (Table 3, entry 1). The progress of the reaction was monitored by TLC. After completion of the reaction, the mixture was dissolved in hot ethanol and the catalyst was filtered. The residue was crystallized from ethanol to afford product 4a (93%). The catalyst was washed with diethylether, dried at 100 °C for 2 h, and reused in another reaction.
4.2.1 3-Amino-1-(2,3-dihydrobenzo[b][1,4]dioxin-6-yl)-5,10-dioxo-5,10-dihydro-1H-pyrazolo-[1,2-b]phthalazine-2-carbonitrile (4a)
Yield 93%; yellow powder; mp 261–263 °C. 1H NMR (400 MHz, DMSO-d6): δ 8.25–8.23 (m, 1H), 8.11 (s, 2H), 8.10–8.06 (m, 1H), 7.96–7.92 (m, 2H), 6.95 (d, J = 8.2 Hz, 1H), 6.82 (d, J = 9.2 Hz, 2H), 6.25 (s, 1H), 4.20 (s, 4H). 13C NMR (100 MHz, DMSO-d6): δ 156.5, 153.9, 150.5, 146.8, 135.5, 134.5, 133.7, 129.0, 128.4, 127.2, 126.6, 120.5, 117.5, 115.5, 112.8, 64.2, 59.5, 58.3. HRMS (ESI, m/z): calcd for C20H14N4O4 (M+H+) 374.1015; found: 374.1011.
4.2.2 3-Amino-1-(benzo[d][1,3]dioxol-5-yl)-5,10-dioxo-5,10-dihydro-1H-pyrazolo[1,2-b]phthalazine-2-carbonitrile (4b)
Yield 90%; yellow powder; mp 259–261 °C. 1H NMR (400 MHz, DMSO-d6): δ 8.24–8.19 (m, 1H), 8.12 (s, 2H), 8.09–8.05 (m, 1H), 7.97–7.93 (m, 2H), 6.75–6.70 (m, 3H), 6.65 (s, 1H), 6.10 (s, 2H). 13C NMR (100 MHz, DMSO-d6): δ 156.5, 153.5, 152.5, 151.4, 146.6, 144.1, 136.5, 134.6, 133.4, 129.2, 128.5, 127.0, 126.1, 118.5, 115.9, 112.5, 104.5, 59.8, 58.6. HRMS (ESI, m/z): calcd for C19H12N4O4 (M+H+) 360.059; found: 360.059.
4.2.3 3-Amino-1-(2-chloro-6-fluorophenyl)-5,10-dioxo-5,10-dihydro-1H-pyrazolo[1,2-b]phthalazine-2-carbonitrile (4c)
Yield 91%; yellow powder; mp 266–268 °C. 1H NMR (400 MHz, DMSO-d6): δ 8.25–8.24 (m, 1H), 8.12 (s, 2H), 8.10–8.06 (m, 1H), 7.98–7.95 (m, 2H), 7.45 (t, J = 8.8 Hz, 1H), 7.30 (dd, J = 2.2, 12.8, Hz, 1H), 7.05 (dd, J = 2.2, 11.0, Hz, 1H), 6.09 (s, 1H). 13C NMR (100 MHz, DMSO-d6): δ 160.4, 156.5, 153.2, 150.4, 134.7, 133.8, 129.7, 129.6, 128.4, 127.5, 126.7, 124.6, 119.7, 117.6, 111.3, 59.9, 57.5. HRMS (ESI, m/z): calcd for C18H10ClFN4O2 368.0476; found: 368.0470.
4.2.4 3-Amino-1-(3,5-difluorophenyl)-5,10-dioxo-5,10-dihydro-1H-pyrazolo[1,2-b]phthalazine-2-carbonitrile (4d)
Yield 92%; yellow powder; mp 272–274 °C. 1H NMR (400 MHz, DMSO-d6): δ 8.28–8.26 (m, 1H), 8.08–8.06 (m, 1H), 8.05 (s, 2H), 7.88–7.86 (m, 2H), 6.95 (d, J = 7.2 Hz, 2H), 6.74–6.69 (m, 1H), 6.39 (s, 1H). 13C NMR (100 MHz, DMSO-d6): δ 164.2, 159.6, 155.6, 154.4, 151.4, 140.3, 134.7, 133.8, 128.9, 128.1, 127.7, 117.4, 110.4, 104.6, 62.9, 60.8. HRMS (ESI, m/z): calcd for C18H10F2N4O2 352.077; found: 352.070.
4.2.5 3-Amino-1-(4-bromo-2-fluorophenyl)-5,10-dioxo-5,10-dihydro-1H-pyrazolo[1,2-b]phthalazine-2-carbonitrile (4e)
Yield 91%; yellow powder; mp 252–234 °C. 1H NMR (400 MHz, DMSO-d6): δ 8.27–8.24 (m, 1H), 8.12 (s, 2H), 8.11–8.07 (m, 1H), 7.96–7.93 (m, 2H), 7.34 (t, J = 8.8 Hz, 2H), 7.01 (dd, J = 2.2, 12.8, Hz, 1H), 6.30 (s, 1H). 13C NMR (100 MHz, DMSO-d6): δ 160.5, 156.6, 153.4, 150.8, 134.7, 133.8, 130.5, 129.7, 128.6, 128.4, 127.3, 126.7, 121.4, 119.4, 115.7, 59.9, 57.5. HRMS (ESI, m/z): calcd for C18H10BrFN4O2411.997; found: 411.997.
4.2.6 Ethyl 3-amino-1-(2,3-dihydrobenzo[b][1,4]dioxin-6-yl)-5,10-dioxo-5,10-dihydro-1H-pyrazolo[1,2-b]phthalazine-2-carboxylate (4k)
Yield 91%; yellow powder; mp 220–222 °C. 1H NMR (400 MHz, DMSO-d6): δ 8.25–8.23 (m, 1H), 8.10 (s, 2H), 8.08–8.06 (m, 1H), 7.96–7.92 (m, 2H), 6.90 (d, J = 8.2 Hz, 1H), 6.72 (d, J = 9.2 Hz, 2H), 6.05 (s, 1H), 4.20 (s, 4H), 4.02–3.95 (m, 2H), 1.02 (t, J = 7.2 Hz, 3H). 13C NMR (100 MHz, DMSO-d6): δ 163.5, 155.5, 153.6, 149.5, 146.4, 135.0, 133.9, 133.0, 129.3, 128.0, 127.2, 126.8, 120.5, 118.5, 116.5, 112.9, 80.5, 60.2, 59.5, 58.2, 14.2. HRMS (ESI, m/z): calcd for C22H19N3O6 (M+H+) 421.127; found: 421.121.
4.2.7 Ethyl 3-amino-1-(benzo[d][1,3]dioxol-5-yl)-5,10-dioxo-5,10-dihydro-1H-pyrazolo[1,2-b]phthalazine-2-carboxylate (4l)
Yield 88%; yellow powder; mp 211–213 °C. 1H NMR (400 MHz, DMSO-d6): δ 8.27–8.24 (m, 2H), 8.16 (s, 2H), 8.09–8.05 (m, 1H), 7.95–7.91 (m, 2H), 6.70–6.68 (m, 2H), 6.50 (s, 1H), 6.12 (s, 2H), 4.00–3.94 (m, 2H), 1.03 (t, J = 7.0 Hz, 3H). 13C NMR (100 MHz, DMSO-d6): δ 162.9, 156.2, 152.5, 151.5, 150.0, 146.4, 144.9, 135.5, 134.3, 133.0, 129.2, 128.2, 127.0, 126.8, 117.5, 116.0, 112.5, 104.5, 80.5, 60.5, 58.6, 13.9. HRMS (ESI, m/z): calcd for C21H17N3O6 (M+H+) 407.112; found: 407.115.
4.2.8 Ethyl 3-amino-1-(2-chloro-6-fluorophenyl)-5,10-dioxo-5,10-dihydro-1H-pyrazolo[1,2-b]phthalazine-2-carboxylate (4m)
Yield 88%; yellow powder; mp 217–219 °C. 1H NMR (400 MHz, DMSO-d6): δ 8.22–8.18 (m, 1H), 8.10 (s, 2H), 8.08–8.04 (m, 1H), 7.94–7.90 (m, 2H), 7.35 (t, J = 8.0 Hz, 1H), 7.20 (d, J = 7.8 Hz, 1H), 7.05 (dd, J = 2.2, 11.0, Hz, 1H), 6.10 (s, 1H), 3.99–3.94 (m, 2H), 1.05 (t, J = 7.2 Hz, 3H). 13C NMR (100 MHz, DMSO-d6): δ 163.6, 161.0, 156.5, 153.0, 150.2, 134.7, 133.8, 129.4, 129.0, 128.4, 127.5, 125.7, 124.3, 118.7, 117.6, 112.3, 81.1, 58.9, 56.5, 14.3. HRMS (ESI, m/z): calcd for C20H15ClFN3O4 (M + H+) 415.074; found: 415.074.
4.2.9 Ethyl 3-amino-1-(3,5-difluorophenyl)-5,10-dioxo-5,10-dihydro-1H-pyrazolo[1,2-b]phthalazine-2-carboxylate (4n)
Yield 90%; Yellow powder; mp 246–248 °C. 1H NMR (400 MHz, DMSO-d6): δ 8.25–8.22 (m, 1H), 8.12–8.08 (m, 1H), 8.06 (s, 2H), 7.98–7.92 (m, 2H), 6.85 (d, J = 7.2 Hz, 2H), 6.70 (d, J = 7.2 Hz, 1H), 6.25 (s, 1H), 3.96–3.90 (m, 2H), 1.02 (t, J = 7.0 Hz, 3H). 13C NMR (100 MHz, DMSO-d6): δ 164.0, 162.2, 159.5, 156.6, 153.6, 151.3, 141.3, 135.7, 133.3, 128.9, 128.3, 127.7, 117.4, 110.4, 104.6, 80.3, 60.8, 58.4, 13.8. HRMS (ESI, m/z): calcd for C20H15F2N3O4 (M + H+) 399.103; found: 399.100.
4.2.10 Ethyl 3-amino-1-(4-bromo-2-fluorophenyl)-5,10-dioxo-5,10-dihydro-1H-pyrazolo[1,2-b]phthalazine-2-carboxylate (4o)
Yield 89%; yellow powder; mp 215–217 °C. 1H NMR (400 MHz, DMSO-d6): δ 8.27–8.24 (m, 1H), 8.10 (s, 2H), 8.09–8.06 (m, 1H), 7.96–7.93 (m, 2H), 7.30 (t, J = 8.8 Hz, 2H), 7.01 (dd, J = 2.2, 12.8, Hz, 1H), 6.23 (s, 1H), 4.00–3.94 (m, 2H), 1.03 (t, J = 7.0 Hz, 3H). 13C NMR (100 MHz, DMSO-d6): δ 162.9, 160.0, 156.5, 154.4, 151.8, 134.7, 133.2, 130.2, 128.2, 128.6, 128.3, 127.2, 126.9, 121.4, 118.4, 116.7, 80.5, 58.0, 56.5, 13.9. HRMS (ESI, m/z): calcd for C20H15BrFN3O4 459.023 (M+H+) 459.023; found: 459.023.
Acknowledgements
The authors express their grateful thanks to Department of Science and Technology (DST), New Delhi for providing financial assistance (CS/014/2013 dated 06/05/2013).