1 Introduction
Malaria remains the most important parasitic disease affecting humans, with around 214 million people being infected and 500,000 deaths per year [1]. Given the emergence of parasite resistance against most of the clinically established drugs and even with the last developed Artemisinin-based Combination Therapies, there is a permanent and urgent need to develop new chemical compounds with original mechanisms of actions to circumvent the drug resistance.
Inspired by the anti-cancer activity of cis-platin, a large number of metal-based compounds have been investigated for their medicinal activities [2] in particular for the treatment of malaria [3]. Among the metal-based therapeutic compounds, gold complexes have gained more attention in the search of anti-cancer [4,5] or antimicrobial [6] agents.
Based on the clinical use of auranofin, a thiolate-phosphine gold(I) complex, for the treatment of arthritis, the search of a gold(I) complex as a therapeutic compound can be envisioned from a toxicological point of view [7]. Moreover Messori and coll. reported in 2008 that auranofin exhibited promising antimalarial activity with an IC50 of 142 nM on the Plasmodium falciparum 3D7 strain [8]. Recent computational studies suggested that a thioredoxin reductase of P. falciparum (PfTR) could be the target of auranofin and that the triethylphosphine-gold was the active therapeutic agent, with the tetraacetylthioglucose ligand being excreted in vivo [9]. Varying the structure of the phosphine ligand should therefore modulate the biological activity of the complex.
Various ligands have been investigated in the search of a gold(I) complex showing potential antimalarial activity. Navarro et al. reported the preparation of gold(I) complexes bearing chloroquine as an N-donor ligand [10–12], which showed improved efficacy against chloroquine-resistant strains of P. falciparum with respect to chloroquine. However, gold complexes of the ferrocenyl-derivative of chloroquine [13] exhibited an antagonistic effect on the overall efficiency, which indicates that the quinoline moiety remains the pharmacophore of these compounds. Ylideneamines were also evaluated as N-donor ligands by Cronje and coll [14]: the corresponding gold(I) complexes exhibit moderate activity against P. falciparum with IC50 ranging from 6.9 to 8.5 μM on the 3D7 strain. C-donor ligands were exemplified by Hemmert and coll. describing the preparation of gold(I) complexes based on bis(N-heterocyclic carbene) ligands [15]. These complexes showed a weak activity against P. falciparum (IC50 above 15 μM on the FcM29-Cameroon strain) except when a pendant quinoline or bipyridine ligand was added to the NHC structure (IC50 of 1.1 and 0.33 μM, respectively). Gold(I) alkynyl complexes were also proposed and evaluated against 3D7 or K1 P. falciparum strains with IC50 in the 7.2–23.6 μM range [16]. Moderate antiplasmodial activities were also reported by Chibale and coll [17] with gold (I) complexes based on thiosemicarbazone ligands acting as S-donor ligands (IC50 of 2.0–6.9 μM against D10 or W2 strains). Finally, data concerning gold(I) complexes bearing P-donor ligands can also be found in the literature [18]. [Au(PEt)3Cl] [8] has an IC50 of 2.1 μM on the P. falciparum strain 3D7 [8], while gold(I) complexes bearing 1,1′-bis(diphenylphosphine)metallocene ligands [19] have IC50 in the 1.8–4.2 μM range on the W2 strain [19].
On the other hand, Réau and Davioud-Charvet reported the preparation and the biological evaluation of gold(I) phosphole complexes as inhibitors of human glutathione reductase and thioredoxin reductase [20–22]. Given the putative key-role of the antioxidant enzyme thioredoxin reductase PfTR in P. falciparum metabolism [9], gold(I) phosphole complexes should therefore be explored as antimalarial agents.
Following our report on the synthesis of gold(I) complexes bearing monophosphole ligands and their evaluation as catalysts for enyne cycloisomerisation [23], we report here the synthesis of new gold(I) monophosphole complexes and the evaluation of their in vitro antiplasmodial activity.
2 Results and discussion
2.1 Synthesis and characterization of gold(I) phosphole complexes
Gold(I) complexes 1–4 (Fig. 1) bearing phenyl-substituted phosphole ligands TMP L1, DBP L2, TPP L3 and DMP L4 respectively were prepared under mild conditions using [Au(SMe2)Cl] as the precursor, as previously described [23]. These complexes were obtained in good yields and were isolated as air- and moisture-stable solids, except for [Au(DMP)Cl] 4.
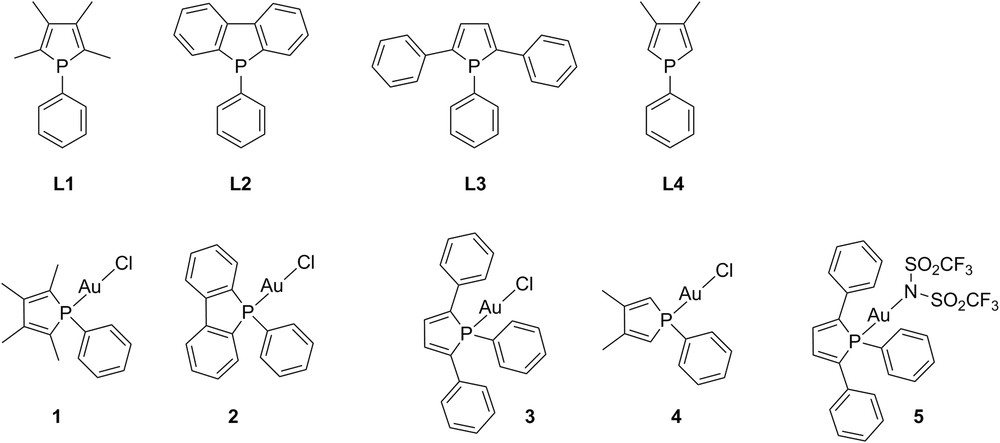
Phosphole ligands L1–L4 and their gold(I) complexes 1–5.
In order to study the influence of the X-ligand on the biological activity of the complex, the phosphole gold(I) complex 5 (Fig. 1), analogous to complex 3 and bearing a bis(trifluoromethanesulfonyl)imidate ligand instead of the chloride one, was also prepared.
The procedure described by Gagosz and coll [24] was followed and the spectroscopic data were in agreement with those previously reported.
Suitable single crystals of complex 5 were grown from diffusion of pentane into a dichloromethane solution and the molecular structure has been established by X-ray diffraction studies (Fig. 2). Crystal data confirm that [Au(TPP)NTf2] 5 is in a slightly distorted linear geometry, with an AuP distance similar to that observed for [Au(TPP)Cl] 3 (Au(1)P(1) 2.2241(6)) [23]. As expected, the AuN distance is smaller than the AuCl distance in [Au(TPP)Cl] 3 (Au(1)Cl(1) 2.2817(6)) [23] but consistent with the AuN distances observed in [Au(PPh3)NTf2] (Au(1)N(1) 2.101(2)) [24] or in its derivative [Au(PPh3)NTfR] recently developed by Newcombe et al. (Au(1)N(1) 2.084(5)) [25].
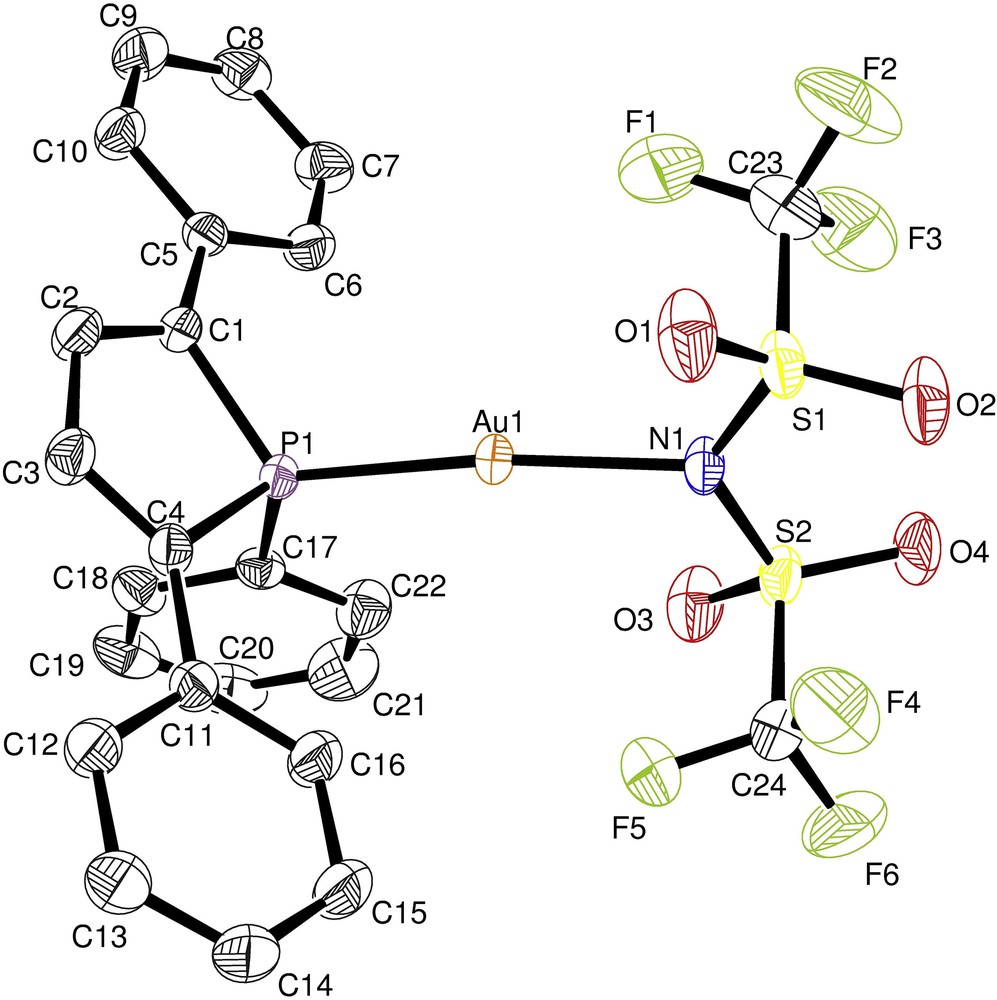
Ortep plot of 5. Thermal ellipsoids are drawn at the 50% probability level, and the hydrogen atoms have been omitted for clarity. Selected bond lengths (Å) and angles (deg) with estimated standard deviations: Au(1)P(1) 2.2282(4), Au(1)N(1) 2.1053(12), N(1)Au(1)P(1) 173.20(4), and C(4)P(1)C(1) 94.00(7).
Then, the introduction of an amine moiety on the phosphole skeleton was envisioned to increase the number of H-bond acceptors in the compound. Following the synthetic route we described in 2002 [26], we prepared a new pyrrolidinophosphole ligand and the corresponding gold(I) complex 6 (Scheme 1). 1-Cyano-2,3,4,5-tetramethylphosphole 6a was easily obtained from TMP L1 through the reductive cleavage of the phosphorus-phenyl bond with an excess of metallic lithium followed by the addition of BrCN after the neutralisation of PhLi using AlCl3 (Scheme 1). The pyrrolidinophosphole ligand L6 was then prepared from the crude cyanophosphole 6a and the preformed amide salt 6c, obtained by action of n-BuLi on (R)-2-(diphenylmethyl)pyrrolidine 6b (Scheme 1). The new gold(I) pyrrolidinophosphole complex 6 was finally prepared using [Au(SMe2)Cl] as the precursor under mild conditions: a white air-stable powder was obtained in a 48% yield from the cyanophosphole precursor.
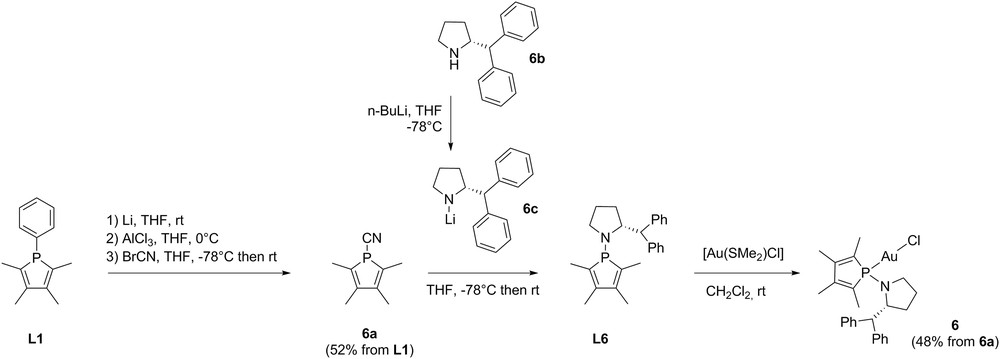
Synthesis of the pyrrolidinophosphole ligand L6 and its corresponding gold(I) complex 6.
Complex 6 was fully characterized by spectroscopic techniques. In comparison with the 31P chemical shift of [Au(TMP)Cl] 1 (δ = 42.8, s, CD2Cl2) [23] a downfield shift was observed for complex 6 (δ = 79.6, s, CDCl3) as expected since the pyrrolidinophosphole ligand L6 is a more electron rich P-donor ligand than the corresponding TMP L1. This 31P NMR chemical shift higher than 70 ppm is also consistent with those observed by Bella et al. [27] or Zhang et al. [28] for a phosphorus atom in a similar R1R2NPAu environment.
The molecular structure of 6 has been established by X-ray diffraction studies (Fig. 3) on suitable single crystals grown from diffusion of pentane into a dichloromethane solution of the complex. Complex 6 exhibits a linear geometry (PAuCl 178.24(2)) with the pyrrolidinophosphole acting as a P-donor monodentate ligand. The AuP and AuCl distances compare well with those observed for [Au(TMP)Cl] [23] 1 (Au(1)P(1) 2.2241(12), Au(1)Cl(1) 2.2854(13)), indicating that the introduction of the amino moiety does not significantly change the structural features of the gold environment. The NP distance is comparable to that observed in platinum-complexes bearing aminophosphole ligands (1.652–1.666) [29] and smaller than that observed in a gold complex bearing an amino-diphenylphosphine ligand (1.679) [30].

Ortep plot of 6. Thermal ellipsoids are drawn at the 50% probability level, and the hydrogen atoms have been omitted for clarity. Selected bond lengths (Å) and angles (deg) with estimated standard deviations: Au(1)P(1) 2.2278(5), Au(1)Cl(1) 2.3012(6), N(1)P(1) 1.6577(17), P(1)Au(1)Cl(1) 178.24(2), and N(1)P(1)Au(1) 113.16(6).
2.2 In vitro antimalarial activity
Complexes 1–6 were tested against the chloroquine-resistant P. falciparum strains FcM29-Cameroon (IC50 of chloroquine (CQ) = 494 nM; of artemisinin (ART) = 12.7 nM) or FcB1-Colombia (IC50 of CQ = 187 nM; of ART = 5 nM) and compared to the commercially available phosphine gold(I) complex [Au(PPh3)Cl]. The results of these in vitro antiplasmodial tests are presented in Table 1.
In vitro antiplasmodial activities of gold(I) complexes on the P. falciparum strain FcM29-Cameroona and on FcB1-Colombiab; [n: number of independent experiments performed].
Compound | IC50 (μM) |
[Au(TMP)Cl] 1 | 13 ± 0.4 (n = 4)a |
[Au(DBP)Cl] 2 | 9.7 ± 2.0 (n = 6)a |
[Au(TPP)Cl] 3 | 24 ± 3.6 (n = 7)a |
[Au(DMP)Cl] 4 | 18.5 ± 3.0 (n = 7)a |
[Au(PPh3)Cl] | 7.9 ± 0.5 (n = 6)a |
[Au(TPP)NTf2] 5 | 24 ± 1.8 (n = 5)a |
[Au(L6)Cl] 6 | 11.5 ± 0.9 (n = 3)b |
Chloroquine | 0.494 ± 0.05 (n = 4)a |
0.187 ± 0.02 (n = 3)b | |
Artemisinin | 0.0127 ± 0.005 (n = 4)a |
0.005 ± 0.003 (n = 3)b |
All the gold(I) complexes 1–4 bearing a monophosphole ligand as the L-ligand and a chloride ligand as the X-ligand showed moderate activity with IC50 values between 9.7 and 24 μM which are several orders of magnitude higher than that observed for CQ itself (0.5 μM). No correlation could be established between the biological activity of the complex and the electronic (σ-donor ability) or steric (%VBur) properties of the corresponding ligand [23] and no phosphole gold(I) complexes 1–4 permitted to increase the activity of their phosphine counterpart [Au(PPh3)Cl] (IC50 7.9 μM).
According to these first results, pharmaco-modulations of the gold(I) phosphole complexes were attempted to increase the antiplasmodial activity. Moreover, in order to increase the stability of the complexes, their solubility in a physiological medium and their potential biocompatibility, the introduction of a nitrogen moiety was envisioned either in the X-ligand or in the L-ligand structures. [Au(TPP)NTf2] 5 was easily obtained as an air-stable compound but the replacement of the chloride ligand by the bis(trifluoromethanesulfonyl)imidate NTf2 did not lead to any improvement of the in vitro antiplasmodial activity: both [Au(TPP)NTf2] 5 and [Au(TPP)Cl] 3 showed a moderate activity with an IC50 of 24 μM (Table 1). In contrast, Hoffmann and Viseux [25] showed that good cytotoxic activities on two cancer cell lines could be achieved with [Au(PPh3)NTf2] while [Au(PPh3)Cl] was totally inactive on the same cells.
The introduction of an amine moiety in the L-ligand backbone was achieved through the synthesis of the pyrrolidinophosphole ligand L6 and its corresponding gold(I) complex [Au(L6)Cl] 6. Unfortunately, this new structural modulation did not lead to any significant improvement of the biological activity: an IC50 of 11.5 μM was obtained on the FcB1 strain for [Au(L6)Cl] 6, which is in the same range as the IC50 of 13 μM obtained on the FcM29-Cameroon strain for the analogous [Au(TMP)Cl] 1.
Finally, the six prepared gold(I) phosphole complexes 1–6 showed IC50 in the range 9.7–24 μM against P. falciparum chloroquino-resistant strains.
3 Conclusions
In summary, a series of gold(I) complexes featuring a monophosphole ligand has been prepared, fully characterized and evaluated by the in vitro antiplasmodial reference assay.
Whatever the modulations achieved on the phosphole skeleton or on the X-ligand, the prepared gold(I) phosphole complexes showed moderate IC50 in the range 9.7–24 μM against chloroquine-resistant strains of P. falciparum, which precludes such as their development as antimalarial compounds. The weak antiplasmodial activities of our gold(I) phosphole complexes may be due to their incapacity of reaching their biological target; further studies are ongoing in our laboratory to address this issue [31]. Moreover, other biological activities are currently investigated for these gold complexes such as anti-cancer properties [32].
4 Experimental section
4.1 General remarks
All commercially available reagents were used as received. Silver salts were stored under argon in Schlenk tubes. Unless otherwise stated, all reactions were run under argon using Schlenk techniques. Dichloromethane, THF, Et2O and pentane were dried under nitrogen using a solvent purification system (SPS). NMR spectra were recorded at 25 °C on a Bruker Avance 500, 400 Ultrashield, a DPX300 or Fourier 300 Ultrashield apparatus. 1H NMR and 13C{1H} NMR chemical shifts are referenced to the solvent signal. 31P{1H} NMR chemical shifts are referenced to an external standard (85% aqueous H3PO4). Multiplicity is as follows: s = singlet, d = doublet, and t = triplet. ESI analyses were performed on an API-365 spectrometer.
4.2 Synthesis of gold(I) complexes
4.2.1 Synthesis of [Au(TPP)NTf2] 5
The gold chloride complex [Au(TPP)Cl] 3 (0.2 mmol) was dissolved in CH2Cl2 (5 mL), and AgNTf2 (78 mg, 0.2 mmol) was added resulting in the instantaneous formation of the expected silver chloride precipitate. The mixture was stirred for an additional 15 min and filtrated over Celite to remove the silver chloride salts. After evaporation to dryness and drying in a vacuum, complex 5 was obtained quantitatively as a yellow solid. NMR data were in agreement with those reported in the literature [24].
4.2.2 Synthesis of [Au(L6)Cl] 6
In a flamed Schlenk, (R)-2-(diphenylmethyl)pyrrolidine 6b (86.15 mg, 0.363 mmol, 1 equiv) was diluted in 5 mL of THF and cooled down to −78 °C. n-BuLi (226 μL, 0.363 mmol, 1 equiv) was added dropwise. The solution turned orange. After 30 min, a solution of TMP-CN 6a [26](60 mg, 0.363 mmol, 1 equiv) in 5 mL of THF was added dropwise. The solution was left for 1 h at −78 °C and allowed to warm up to room temperature for over 2 h. The dark red solution was then concentrated to dryness and extracted with approximately 3 × 8 mL of Et2O. These extracts were then concentrated again to dryness and extracted with 3 × 8 mL of pentane. Ligand L6 was finally obtained as a yellow oil and was used for the complexation step without further purification (31P{1H} NMR (121.5 MHz, Told8) δ 62.5).
To a solution of the ligand L6 (80 mg, 0.21 mmol, 1.4 equiv) in dry CH2Cl2 (5 mL), was added chloro(dimethylsulfide)gold(I) [Au(SMe2)Cl] (45 mg, 0.15 mmol, 1 equiv) and the reaction mixture was stirred at room temperature for 2 h. After concentration and precipitation with pentane, a white solid was obtained in 48% yield from 6a.
31P{1H} NMR (121.5 MHz, CDCl3) δ 79.6; 1H NMR (300 MHz, CDCl3) δ 7.49–7.21 (m, 10Har), 4.76 (m, 1H, HC-N), 3.9 (d, 3JHH = 12 Hz, 1H, HC(Ph)2), 2.88 (m, 1H, HCHN), 2.61 (m, 1H, H′CHN), 1.9 (m, 4H, CH2), 1.86 (s, 3H, CH3), 1.82 (s, 3H, CH3), 1.79 (d, 3H, CH3, 3JHP = 12 Hz), 1.30 (d, 3H, CH3, 3JHP = 12 Hz).
13C{1H} NMR (75 MHz, CDCl3) δ 146.3 (C), 145.9 (C), 145.4 (C), 142.3 (C), 142.2 (C), 129.3 (CH), 129.2 (CH), 128.8 (CH), 126.8 (CH), 126.7 (CH), 125.6 (CH), 69.2 (CH, 2JCP = 7.5 Hz), 56.3 (CH2), 45.6 (CH), 30.9 (CH2), 24.7 (CH2), 13.6 (CH3), 11.0 (CH3, 2JCP = 18 Hz), 10.7 (CH3, 2JCP = 18 Hz).
Mass: ESI m/z calc. for C25H30AuClNP: 607.1, found: 572.1 [M−Cl].
4.3 Crystal structure determination
Diffraction data were collected at low temperature (180 K) on a Bruker Kappa Apex II using graphite-monochromated Mo Kα radiation (λ = 0.71073 Å). The diffractometer is equipped with an Oxford Cryosystems Cryostream cooler device. The structures were solved by direct methods SHELXS-97. All non-hydrogen atoms were refined anisotropically by means of least-squares procedures on F2 with the aid of the program SHELXL-97 [33]. Crystallographic data have been deposited at the Cambridge Crystallographic Data Centre with deposition numbers CCDC 1039395 and 1039396.
4.4 Biological evaluation: P. falciparum cultures and antiplasmodial assays
The chloroquine-resistant P. falciparum strains FcM29-Cameroon and FcB1-Colombia were in vitro continuously cultured as already published [34,35]. The standard radioisotopic micro-method [35,36] was used to evaluate the antiplasmodial activity of these complexes. Parasite cultures were incubated with various concentrations of the drug for 48 h. The molecules tested were firstly dissolved in dimethyl sulfoxide and then in RPMI 1640 (Gibco Invitrogen, USA) to obtain solutions ranging from 50 μg/mL to 50 ng/mL. In parallel, both antimalarial compounds chloroquine (Bufa, Holland) and artemisinin (TCI, France) were tested and used as controls. Results obtained with standard antiplasmodial drugs chloroquine and artemisinin for both strains FcM29-Cameroon (IC50 CQ = 494 nM; IC50 ART = 12.7 nM) and FcB1-Colombia (IC50 CQ = 187 nM; IC50 ART = 5 nM) are in accordance with the literature [35]. Parasite growth was estimated by [3H] hypoxanthine incorporation (PerkinElmer, France). The IC50 values reported are the mean of at least three independent experiments (the number of independent experiments performed is reported in Table 1).
Acknowledgements
Thanks are due to the Centre National de la Recherche Scientifique (CNRS) for financial support and the French “Ministère de l'Éducation Nationale et de la Recherche” for the Ph.D. grant awarded to Kévin Fourmy. The authors also thank Sonia Mallet-Ladeira for crystal structure determination.
Supplementary material
CCDC 1039395 and 1039396 contain the supplementary crystallographic data for this paper. These data can be obtained free of charge from the Cambridge Crystallographic Data Centre via www.ccdc.cam.uk/data_request/cif.