1 Introduction
In past few years, public awareness of environmental protection is increasing. Ecofriendly agriculture implies the control of plant protection products. These are tools to obtain high crop yields but their uncontrolled use in conjunction with inorganic salts, such as copper nitrate and sulfate, could cause negative impacts to the ground, water, and living organisms.
Pesticides and their metabolites are usual contaminants of groundwater [1] but imidazolinone herbicides (imazapyr, imazapic, imazethapyr, imazamox, and imazaquin) present low toxicity for humans, being an alternative to phenoxy herbicides, such as 2,4-D and 2,4,5-T, or to triazine herbicides, especially those of the atrazine family.
In a previous article [2], the speciation of imidazolinone herbicides in aqueous media was studied from ultraviolet–visible (UV–vis) and potentiometric measurements. The effect of pH in the natural dynamics of these herbicides was discussed. Triazines are pesticides belonging to the group of herbicides used to control weed growth. The action of triazines is most based on the first stages of the plant growth, because they destroy a wide variety of plants in the first days of germination (10–15 days). They are used in agriculture in an extended way and are of pre-emergence and postemergence type. They also make the photosynthesis largely impossible, because many of them are absorbed by the root system and are accumulated in the leaves of the plant.
Herbicides may have a great impact on metal biocycles in soil [3,4], interacting with clay components. These heterocyclic compounds form complexes with metal ions as Co(III), Mn(II), Ni(II), Cu(II), and so forth [3–8]. These complexes have been studied mainly in solid state by EPR, IR or FTIR, and ESI mass spectrometry, but their behavior in aqueous solutions is poorly known.
Copper derivatives are commonly used on crop foliage to control fungal disease [8,9], being an essential metal for plants, and playing a key role in photosynthesis [10,11].
The aim of this work is, first, to determine the stability constants of the complexes formed by the imidazolinone and triazine herbicides when they interact with Cu(II) ions, by means of rapid, easy, and nonexpensive measurements performed by UV–vis spectroscopy, for the imidazolinone herbicides, and by electrochemical measurements, for the triazine herbicides, and second, to relate the stability of these complexes with the dynamics of the herbicides and the copper ions in the soils.
2 Materials and methods
In all cases, Merck analytical grade reagents were used with the exception of imidazolinone herbicides, which were from Riedel de Haën (analytical standards). All other reactants were used without further purification. Solutions were prepared using ultrapure water type I (resistivity 18.2 MΩ cm at 25 °C) obtained by an ultrapure water system Millipore Simplicity.
Spectrophotometric studies were performed using a double beam Varian Cary 100BIO spectrophotometer. Hanna quartz cuvettes of 1 cm path length were used. Electrochemical measurements were performed with an Autolab PGSTAT302N potentiostat using the software package NOVA 1.7. A three-electrode cell equipped with a Pt wire counter electrode and a BAS MF-2079 Ag/AgCl3 M KCl reference electrode was used. The working glassy carbon electrode of 38.5 mm2 area was polished with a silicon carbide paper, followed by diamond (0.25 μm) slurry and alumina (0.3 and 0.05 μm) slurries. Residual polishing material was removed from the surface by sonication of the electrode in water bath for 30 min. Solutions were purged with purified nitrogen and the temperature was kept at 25 ± 0.1 °C.
3 Results and discussion
3.1 Imidazolinone herbicides
Imidazolinone herbicides present three pK values corresponding to acid–base equilibria [2], which means that the possible copper complexes formed depend on the pH, i.e. on the protonation state of the herbicide.
The lowest pK values (pK1) correspond to the simultaneous protonation–dissociation equilibria of the pyridinic (or quinolinic) nitrogen and the carboxyl group. The following pK (pK2) corresponds to the iminium nitrogen and the basic pK (pK3) to the dissociation of the imido nitrogen. The pK values are presented in Table 1.
pK values of the protonation–dissociation reactions of the imidazolinone herbicides in acidic media [2].
Herbicide | R | pK1 | pK2 | pK3 |
Imazapyr | H | 1.9 | 3.6 | 10.8 |
Imazapic | –CH3 | 2.1 | 3.9 | 11.1 |
Imazethapyr | –CH2–CH3 | 2.1 | 3.9 | 10.5 |
Imazamox | –CH2–O–CH3 | 2.09 | 5.04 | 10.8 |
Imazaquin | Quinoline | 1.8 | 3.7 | 11.03 |
At pK1 < pH < pK2 (below pH 6 and until pH ca. 2.5), the predominant form of the herbicide in the solution is a zwitterion having both positive and negative charges (see Scheme 1). In addition, the herbicides will be negatively charged at pH greater than pK2 and lower than ca. 11, which includes virtually all of the soils. These pK values are important in the discussion of the effect of pH in the natural dynamics of imidazolinone herbicides. This is especially true in the case of the soil sorption of the herbicides, because of the possible occurrence of interactions with the soil colloids. So, three pH values were selected to investigate the complex formation (1, 2.8, and 6.8), at which the predominating forms of the herbicides are shown in Scheme 1.

Protonation–dissociation reactions of the imidazolinone herbicides.
The stability constants of the complexes of imidazolinone herbicides with Cu(II) ions were obtained by spectroscopic measurements. First, the UV–vis spectra of both the Cu(II) solutions and the herbicides were recorded to ensure the applicability of the method. At the lower pH values, the Cu(II) spectra showed a band with a maximum wavelength placed around 190–200 nm. Fig. 1 shows the spectra obtained at pH 6.8 in phosphate-buffered solution (PBS) and water. As it can be seen, the spectra obtained in buffered solutions showed a broad band having significant absorbance at wavelengths of 250–350 nm, whereas the spectra for the unbuffered solutions absorbed only at very low wavelengths. At pH 2.8, the spectra corresponding to PBS solutions were virtually the same as those obtained in water, both very similar to the spectrum obtained at pH 6.8 in water. These results must be because of the formation of complexes between Cu(II) and the HPO42− ions, which are not formed with HPO4–.
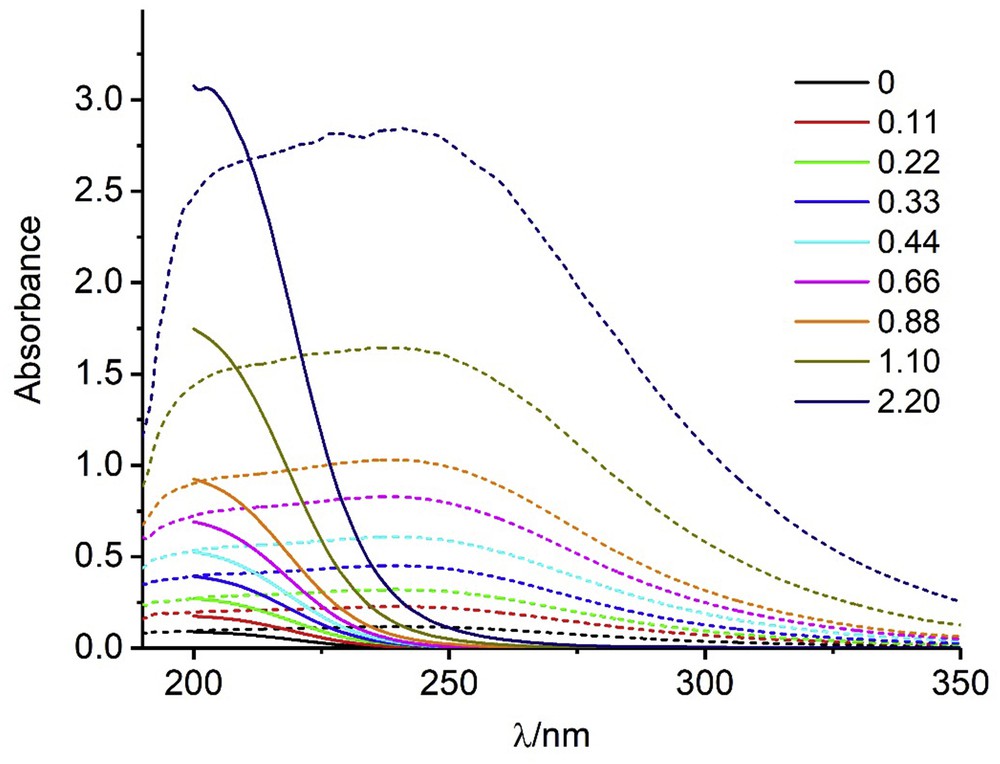
UV–vis spectra at pH 6.8 and different Cu(II) concentrations (in mmol L−1). Continuous lines, water; dotted lines, 0.1 M H3PO4.
The UV spectra of the imidazolinone herbicides at pH < 3 show a main band centered about 192–198 nm, virtually pH-independent, and a second band having less intensity, having a maximum around 232–235 nm; at 3 < pH < 6.5, the main band shifts to a wavelength of 203–208 nm and a new band centered at 268–272 nm appears [2]. For this reason, the use of PBS solution for neutral media was discarded, and the experiments at these pH values were performed in water.
The formation of the complexes between the herbicides and Cu(II) ions is evidenced from the effect of the addition of such ions to a solution of herbicide. In Fig. 2 are shown the results obtained for imazapic as an example for the rest. Because the lower bands of the imidazolinones appear at the same wavelengths as the Cu(II) solutions, the graphs show only the absorbances at λ > 240 nm.

UV–vis spectra of solutions at pH 6.8 at different Cu(II) concentrations (in mmol L−1). Continuous lines, water; dotted lines, 0.1 M H3PO4.
As the Cu(II) concentration increases, the absorbances increase as can be seen in Figs. 2 and 3. The Cu(II) and the herbicide do not present measurable absorbances at wavelengths higher than 250 nm. So, the increases shown in Fig. 3 at wavelengths close to 300 nm are not because of the absorbance of the Cu(II) itself but because of the formation of a complex between the herbicide and the ions.
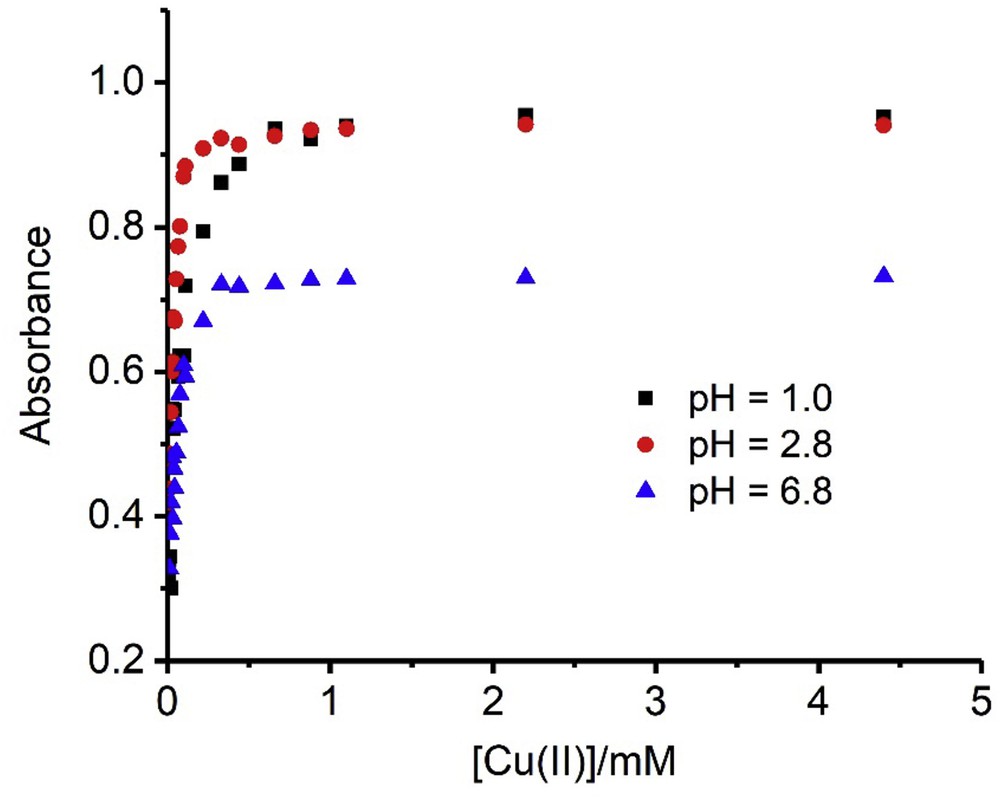
Imazapic 1.0·×·10−4 M. Dependence of the absorbances on the Cu(II) ion concentration. The wavelengths were 320, 290, and 300 nm for pH 1.0, 2.8, and 6.8, respectively.
The above conclusion is supported by the constant absorbance observed in Fig. 3 at high Cu(II) concentrations, which can be attributed to the stoichiometric formation of the complex, its concentration being limited by that of imazapic, which is much lower than the Cu(II) concentration.
From these measurements it is possible to calculate the formation constants of the complexes, provided their stoichiometry is known. So, the formation of the complex between a metal ion, in this case Cu(II), and a ligand L, in this case the imidazolinone herbicide, can be schematized as
The formation constant being
If Cm and CL are the metal ion and ligand concentrations that form a given mixture, and x the concentration of ion, which reacts with nx mol L−1 of ligand, then [M] = Cm – x, [L] = CL – nx, and [MLn] = x.
At an ion concentration high enough with respect to the ligand concentration, the amount of complex formed is virtually CL/n. In this case, it is easy to obtain the molar extinction coefficient of the complex at a given wavelength. From this value and the molar extinction coefficient of the ligand obtained in the absence of metal ion, the values of x for different Cm and CL values can be obtained and Kf can be calculated.
To determine the value of the n parameter one can take advantage of the very low and very high ratios between Cm and CL. Thus, when CL ≫ Cm, x is very high, and the absorbance is roughly proportional to Cm, and when CL ≪ Cm, x is very low and the absorbance is roughly proportional to CL. Fig. 4 shows the plots of absorbance versus CL/Cm for imazapic, from which the stoichiometry of the complexes can be obtained. Two behaviors were obtained for all herbicides, with n = 1, at pH = 1, and n = 2 in the rest of cases.

Imazapic 1.0 × 10−4 M. Dependence of the absorbances on the ratio CL/Cm. The wavelengths were the same as those given in Fig. 3.
Thus, from the intermediate concentrations, that is, comparable CL and Cm values, the Kf values were calculated for each herbicide and the results are gathered in Table 2. At least eight calculations have been performed for each constant and the uncertainty in the log Kf values was ±0.3 units.
Log Kf values of the complex formation between the imidazolinone herbicides and Cu(II) ions in acidic and neutral media.
Herbicide | pH = 1.0 | pH = 2.8 | pH = 6.8 |
Imazamox | 4.35 | 4.37 | 9.87 |
Imazapic | 4.11 | 3.78 | 9.15 |
Imazethapyr | 4.24 | 7.63 | 10.61 |
Imazapyr | 3.89 | 7.61 | 10.35 |
Imazaquin | – | – | 7.70 |
Probably, the two positive charges of the imidazolinone species predominating in very acidic media (see Scheme 1) are responsible for the 1:1 stoichiometry of the complex at pH 1. So, the electrostatic repulsion between these charges and the Cu(II) cation compensates in part the stabilization of the complex. At less acidic pH, the negative charge of the zwitterion allows the formation of the 1:2 complex, but it is much less stable than the complex formed at pH 7, at which the herbicide is an anion. At these neutral pH values the stability of the complex is at least three orders of magnitude higher than at pH 2.8. It must be noted that imazaquin complex can be assessed only at neutral pH. This can be because of the much lower stability of the complexes formed in very acidic media or, more probably, to the much lower modification of the spectra at these pH values due to the quinoline ring.
3.2 Triazine herbicides
Triazine herbicides present only one pK value corresponding to the protonation–dissociation of the pyridinic nitrogen, which are given in Table 3. As it can be seen, the pK values of the chloro-derivatives are lower than 1.7 and those of the rest are around 4–4.3, which means that for virtually any soil, the herbicides are in their unprotonated forms. For this reason, the studies were carried out at neutral pH.
pKa values of the pyridinic nitrogen of the triazine herbicides.
Compound | X | R1 | R2 | pK |
DTa | H | H | H | 1.2 |
CDTb | Cl | H | H | 1.2 |
SDTc | Cl | –OCH3 | –OCH3 | 1.1 |
Atrazine | Cl | CH3CH2– | (CH3)2–CH– | 1.6 |
Atraton | –OCH3 | CH3CH2– | (CH3)2–CH– | 4.3 |
Propazine | Cl | (CH3)2–CH– | (CH3)2–CH– | 1.2 |
Prometryn | –SCH3 | (CH3)2–CH– | (CH3)2–CH– | 4.0 |
Prometon | –OCH3 | (CH3)2–CH– | (CH3)2–CH– | 4.3 |
Simazine | Cl | CH3CH2– | CH3CH2– | 1.7 |
Simetryn | –SCH3 | CH3CH2– | CH3CH2– | 4.1 |
a 2,4-Diamino-1,3,5-triazine.
b 2-Chloro-4,6-diamino-1,3,5-triazine.
c 2-Chloro-4,6-dimetoxi-1,3,5-triazine.
The spectra of triazine herbicides and parent compounds show absorption bands in the same range of wavelengths than Cu(II) solution [12], this fact preventing the use of the same methodology as for imidazolinone herbicides. As an alternative approach, an electrochemical method has been used. At pH > pK and at potentials below 1 V, triazines are not electroactive [13–15], but Cu(II) shows in cyclic voltammetry (CV) a reduction signal, in the direct scan, and an oxidation wave, in the reverse scan [16]. So, the strategy used in this case was the addition of variable concentrations of ligand to a fixed concentration of metal ion and monitoring the change in the oxidation–reduction signals because of the formation of the complex.
In Fig. 5, the linear-sweep cyclic voltammograms of Cu(II) solutions in the absence and presence of increasing concentrations of the parent compound CDT are shown. As it can be seen, the voltammograms change with the CDT concentration, both in the direct (reduction) and in the reverse (oxidation) scans. This can be only because of the interaction between the Cu(II) ions and CDT, that is, to the complex formation. The redox potential of the complex is different to that of Cu(II).
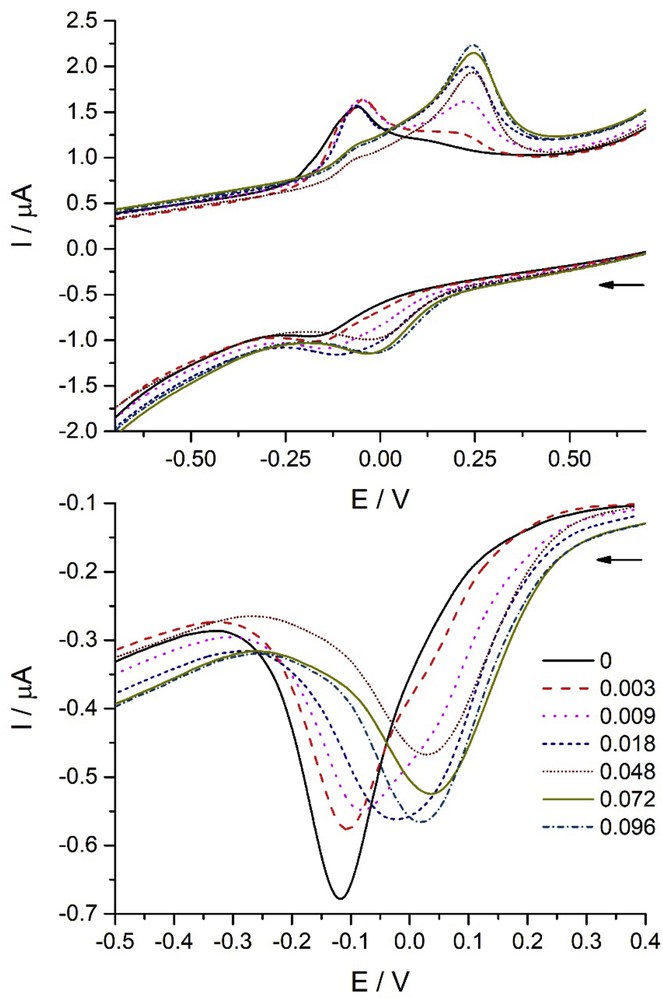
Electrochemical responses of 1.0 × 10−4 M Cu(II) solutions in the absence and presence of different CDT concentrations (in mmol L−1). Upper figure: linear-sweep CV. Scan rate: 0.1 V s−1. Lower figure: differential pulse voltammetry. Scan rate: 0.02 V s−1. Pulse potential: 50 mV. Pulse duration: 50 ms. In both cases, the initial potential was 1 V and the initial scan direction toward negative potentials. Arrows indicate the initial direction of the scan.
The differential pulse voltammograms corresponding to the reduction in Cu(II), also given in Fig. 5, show more clearly the effect. In both cases, the signals change from that corresponding to Cu(II) to that corresponding to the complex, at high CDT concentrations. Because the complex stoichiometry is 1:2 [3,17], its formation reaction is
The formation constant being
The same symbols as in the preceding section will be used.
At a ligand concentration high enough with respect to the ion concentration, the amount of complex formed is virtually Cm. In this case, it is easy to obtain the current of the complex at a given potential from the current at CL = 0 and the actual current. The values of the conversion x for different Cm and CL values can be obtained and Kf can be calculated.
For CDT, the value of log Kf = 10.09 ± 0.25 was obtained from the intensities of the differential pulse voltammograms measured at a potential of 0.1 V. When the calculations are performed by taking the intensity of the oxidation signal in CV at 0.25 V, a value of log Kf = 10.07 ± 0.25 is obtained, in good agreement with the preceding value.
For the rest of triazines, the results were similar to those obtained for CDT, although the effect of the triazine concentration on the electrochemical signals varied from a compound to another. Log Kf values are presented in Table 4. In the singular case of DT, the voltammograms of Cu(II) were not affected by the addition of any concentration of the herbicide, probably because the triazine ring in this molecule is not activated by the presence of –Cl,–OCH3, or –SCH3, thus preventing the formation of the complex.
Log Kf values of the complex formation between the imidazolinone herbicides and Cu(II) ions in acidic and neutral media.
Compound | Log Kf |
CDT | 10.08 |
SDT | 11.09 |
Atrazine | 11.62 |
Atraton | 8.91 |
Propazine | 11.05 |
Prometryn | 9.95 |
Prometon | 9.71 |
Simazine | 11.25 |
Simetryn | 9.10 |
3.3 Agricultural and environmental consequences
The results presented in this article indicate that the imidazolinone and triazine herbicides, at pH > 5 and pH < 10, form very stable complexes with the Cu(II) ions in aqueous solutions. This pH range corresponds to many soils of agricultural use. In agriculture, Cu(II) salts are widely used as fertilizer, fungicide, algicide, and to eliminate snails and slugs. If a given crop treated with these herbicides is treated with a Cu(II) salt (or vice versa), the formation of the complexes between the herbicides and the metal ions solubilizes the herbicides, decreasing its persistence and, consequently, its effectiveness. Moreover, this allows the access of the herbicide (and the metal ions) to the phreatic layer of the soil, increasing the chance of pollution.
4 Conclusions
Fast, easy, and inexpensive measurements performed by UV–vis spectroscopy, for imidazolinones, and voltammetry (cyclic and differential pulse), for triazines, have proved to be adequate for the determination of the stability constants of the complexes formed by such herbicides with Cu(II) ions. In both cases and in the conditions corresponding to the great majority of soils of agricultural use, the herbicides form very stable complexes with the Cu(II) ions, the complexes being integrated by two ligands (herbicides) and one copper ion.
Crops treated with such herbicides in conjunction with Cu(II) salts experience a decrease in its persistence and effectiveness. In addition, the herbicides and the copper ions may pass to the phreatic layer of the soil, increasing the chance of pollution. These types of measurements may be extended to study the interaction of other heavy metal ions, with the same herbicides, or other herbicides, with Cu(II) ions.
Acknowledgments
The authors acknowledge financial support from the Córdoba University within the framework of the “Ayudas puente para el desarrollo de proyectos de I+D precompetitivos XX Programa Propio 2015 and XXI Programa Propio 2016”.