1. Introduction
Molecular chirality is one of the most powerful concepts of the twentieth century due to its scope and applications [1]. This natural characteristic integrates all the regulatory processes of development and impacts a wide range of applications, including health (medicines and vitamins); the food industry (flavourings, food additives); agronomy (insect control, herbicides, fungicides); and cosmetics (perfumes, beauty products). Essential oils are complex substances that are particularly affected by this property of nature. They are volatile, lipophilic, odoriferous, and rich in hundreds of molecules produced by the secondary metabolism of plants. These varied molecules are largely involved in the plant's interactions with its environment (protection, communication, energy reserve, etc.). Their components, mainly terpenes (monoterpenes, sesquiterpenes, diterpenes) and their oxygenated derivatives (alcohols, aldehydes, esters, ketones, phenols, etc.) are predominantly chiral molecules [2, 3].
The trend towards "natural" consumption is creating momentum for plant-based product development [4], especially in the beauty and wellness industries [5]. The essential oils market is experiencing significant growth. It exceeded US$7.51 billion in 2018 and is projected to have an annual growth rate of 9% between 2019 and 2026 [6]. These herbal concentrates demonstrate a multitude of virtues: they are anti-inflammatory, anti-infective, immunomodulatory, bronchodilating, antiviral, anti-depressant, and relaxant. Demand for "natural" substances to relieve physical ailments and/or psychological problems increased with the SARS-CoV-2 pandemic, whether for strengthening immunity, integrating into treatment protocols [7, 8], or treating the anosmia often resulting from the disease [9, 10, 11]. The development of an olfactory stimulation protocol [12, 13] based on essential oils has enabled more than a third of people suffering from hyposmia to feel better and regain their sense of smell. In recent months, numerous research studies have explored the antiviral potential of essential oils [14, 15, 16, 17, 18, 19, 20, 21, 22].
We owe the origin of chiral chemistry to the works of Louis Pasteur [23] on molecular asymmetry. However, the French-language aromatherapy literature [24, 25, 26, 27, 28] does not integrate this structural aspect of the molecules, thus severely restricting understanding of the mechanisms involved in their molecular activity. The chemical classification of essential oils is based on a classification by functional groups [29], into which they are first divided in a somewhat reductivist manner where the allocation criteria of the therapeutic activity is based on a function of the molecule (alcohol, ketone, ether, ester, oxide, etc.). Next, specifying the chemotype designates the predominant distinct chemical entity for an individual plant species. This approach to chemical classification [30] has been established since 1975 and was standardised in 2006 within the European Union with the adoption of the REACH Regulation.1 In recent years, many conceptual shortcomings in the classification of molecular families related to the stereochemistry of molecules have been reported [31, 32]. They also target the efficacy and/or toxicity linked to certain functions, and the polyvalence of essential oils that would have several levels of action in synergy.
In this text, the concept of molecular chirality, its importance, and its impacts are introduced. The configurations of the main molecules composing essential oils are presented, highlighting the impact of stereochemical profiles on the biological activity of certain essential oils. The article concludes with a presentation of the pharmacological activity of linalool enantiomers, a chiral molecule naturally present in almost all essential oils. Beyond the chemotype, the importance of the natural chiral signature will be demonstrated with precise molecular configurations and their implications for biological activity and olfactory properties. Towards enantiotypes for essential oils?
2. What is molecular chirality?
Chirality comes from the Greek "kheir" which means hand. It is the property of an object that cannot be superimposed on its image in a mirror, like a right hand and a left hand, which results in a lack of symmetry. The image of the right hand in a mirror corresponds to the left hand and vice versa; however, the two hands cannot overlap. More simply, a right glove can only be put on with a right hand. Likewise, when two molecules in three dimensions, symmetrical with respect to an axis, are referred to as chiral, this indicates an absence of a centre of symmetry or plane of symmetry (Figure 1).

Molecular chirality.
Nature is the most important chiral reservoir. Thus, only (−)-morphine, isolated from the opium poppy plant (Papaver somniferum) [33], has analgesic activity; its synthetic (+) isomer is devoid of it. All of the 𝛼-amino acids have the same configuration (S) with the exception of cysteine. In their natural state, sugars exist as a single enantiomer. Moreover, because of their helical shape and also because of their composition, DNA and RNA are chiral molecules [34, 35].
The two forms of a chiral molecule are called enantiomers. They can have very different biological activity as they interact on different receptors. Several possibilities may be encountered, which are defined as follows:
- (1) The eutomer. This is the enantiomer which has the strongest biological activity, or has the best relative affinity for binding to a biological receptor or enzyme;
- (2) The distomer. This is the enantiomer that is inactive, less active, or has any activity other than the desired activity, and/or may be found to have a different toxicity.
2.1. Enantiomers and medicinal products
This phenomenon is crucial for medications [36] consisting of enantiomers that have very different biological activities, where one can be beneficial and the other very toxic (Figure 2). One can cite the case of Dopa, whose S enantiomer treats Parkinson's disease while the (R) enantiomer is a poison, or Ethambutol, wherein the S enantiomer is an anti-tuberculosis drug while the R enantiomer causes blindness. One of the biggest pharmaceutical scandals of all time is the case of Thalidomide, which led to an awareness of the importance of chirality in the toxicity of molecules in medicines. Prescribed to pregnant women in the early 1960s to relieve nausea, Thalidomide hit the headlines for causing irreversible damage to the development of the foetus. It is estimated that between 10,000 and 20,000 were victims of this drug and born with malformations. In fact, only the R enantiomer is sedative; the other (S) is teratogenic.
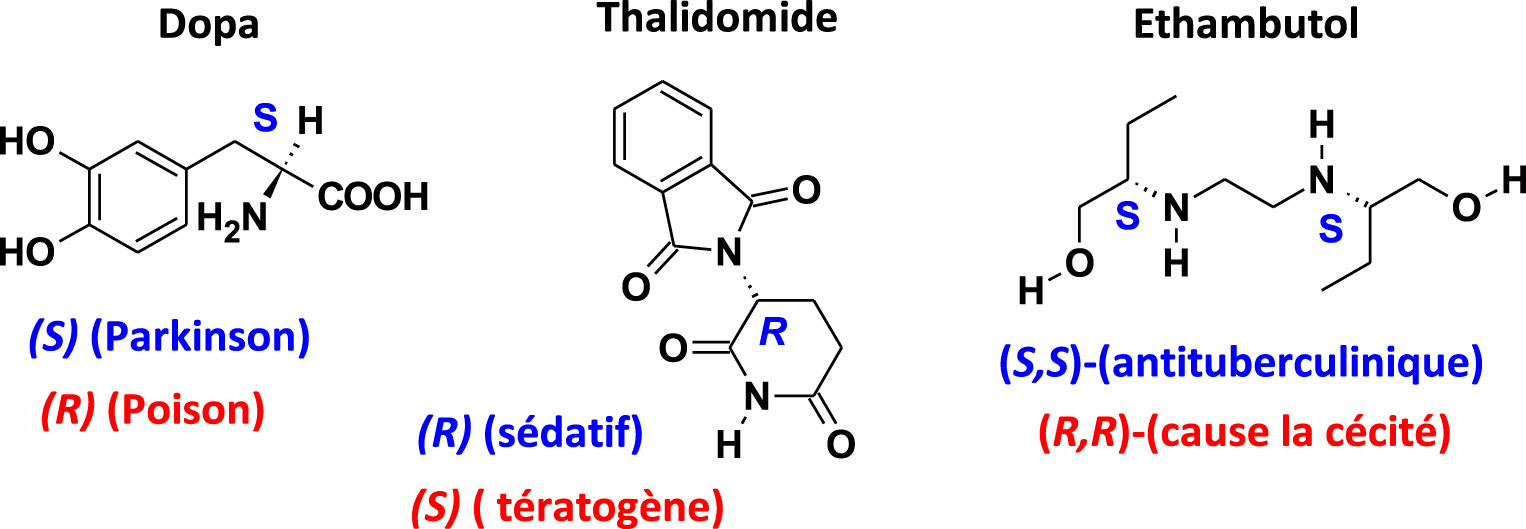
Biological activity of drug enantiomers.
Since 1990, the marketing authorisation for new synthetic medications is subject to very strict regulations [37, 38]. The activity of each of the enantiomers of a new chiral molecule must be evaluated. These restrictions have led to the emergence of chiral chemistry, a field which is experiencing considerable growth, as opposed to traditional organic chemistry synthesis which produces racemic mixtures (two enantiomers in equal quantity).
Today, it is estimated that about 50% of medicines have a chiral structure, and numerous therapeutic active ingredients having one or more centres of chirality are still used today in the form of racemic mixtures. The use of an enantiomer in the place of a racemic mixture is only favourable when "the other" enantiomer adversely influences the benefit-risk ratio of racemic mixing. Pharmacodynamic and pharmacokinetic properties of both enantiomers must be evaluated. In order to do this, the separation of the first milligrams of pure enantiomers for conducting initial activity and toxicity tests is often carried out using chromatography on a chiral support [39, 40]. Strategies for synthesising a unique enantiomer are utilised in a second step if its activity is recognised, in order to access it as needed via asymmetric catalysis or by racemic splitting. The development of asymmetric catalysis [41], which consists of using a chiral catalyst (organometallic complex, biocatalyst, or organocatalyst), has made it possible to produce many industrial syntheses of enantiopure drugs. One can note the impact of "atropisomerism" (axial-type chirality due to blockage of the rotation around a single bond) on the discovery of drugs or natural products [42, 43]. This type of enantiomer has advantageous characteristics, showing that conformational chirality should be treated with as much interest as chirality that is centred on the atom.
This year, asymmetric catalysis was once again honoured via the 2021 Nobel Prize recognising organocatalysis. This important field of research, which makes it possible to create right and left molecules while respecting the principles of green chemistry, was already the recipient of the 2001 Nobel Prize in Chemistry [44], through organometallic catalysts.
3. Recognising a chiral molecule
In simple terms, it can be said that a molecule is chiral if it has at least one centre of asymmetry, which is, most often, a carbon atom (denoted as C*) bonded to four different atoms or groups of atoms (Figure 3).

Centre of chirality.
A molecule having n C∗ may exist at most under 2n spatial isomers, called stereoisomers, consisting of enantiomers, diastereomers, and conformational isomers. Only the enantiomers are mirror images, containing chiral centres and having the characteristic of being non-superimposable. Diastereomers are stereoisomers that are not mirror images of each other. Their physico-chemical properties are different (potential energy, dipole moment, melting point, boiling point, refractive index) [1].
The absolute nomenclature of Cahn, Ingold, and Prelog (CIP) [1] makes it possible to designate the enantiomers and to name them separately. The spatial arrangement of the molecule, the absolute configuration, depends on a set of classification rules for the four ligands attached to the chiral centre, according to a specific sequence in order of priority that is applicable to all types of molecules, on the basis of defined criteria. The four substituents a, b, c, d of asymmetric carbon are classed in order of priority. By placing oneself in the axis of the carbon — the minority substituent, one observes the direction of rotation of the molecule in the order a > b > d: if the molecule rotates to the right, the configuration is R (Rectus); if it rotates to the left, the configuration is S (Sinister) (Figure 4). With opposite spatial geometries, they interact differently with their environment. Central chirality is the most common stereogenic element, but other elements also induce molecular chirality, such as the chirality axis (axial chirality), the chirality plane (planar chirality), or helicity (helical chirality).

Absolute configuration Rectus (R)/Sinister (S).
4. Chiral molecules and the biological activity of essential oils
The same species of plant can synthesise variable proportions of chiral terpenes. The reasons for this phenomenon are not very clear, even if the consequences of this chirality are very important for all living organisms. The enantiomers of a chiral molecule do not react with the same receptors, which are themselves chiral, thus not necessarily inducing the same biological activity. This property is of fundamental importance [45]. This is a decisive factor in controlling the naturalness of essential oils and for the detection of counterfeits.
In olfaction, for example, the binding of the chiral odorant molecule with the chiral receptor triggers the series of molecular events that constitute the transduction of the sensory signal by the sensory cell and ultimately the brain's recognition of the characteristic smell [46]. Smell has become a central focus of research since the discovery of the largest family of genes involved in the production of proteins that are capable of detecting odours, which was awarded the 2004 Nobel Prize in Medicine [47]. These studies show that each olfactory receptor is specifically activated by the structural characteristics of the molecules.
Thus, the olfactory detection threshold may differ strongly between two enantiomers of the same molecule [48]. This is the case, for example, of limonene, carvone, and linalool, present in abundance in citrus essential oils. Their enantiomers have very different fragrances: (S)-(−)-Limonene has a lemon smell while (R)-(+)-Limonene smells of orange; the (R)-(−)-Carvone enantiomer smells like spearmint while the (S)-(+)-Carvone smells like cumin. As for the enantiomers of linalool, (S)-(+)-Linalool has a sweet, floral, citrus fragrance, and (R)-(−)-Linalool has a woody aroma reminiscent of lavender (Figure 5). Moreover, very frequently, the human nose does not detect two enantiomers in the same way. For the (R)-Linalool form, its powerful floral note is perceived at a concentration of 0.8 ppb ,2, while it takes up to 7.4 ppb to appreciate the much less intense smell of (S)-Linalool [49].
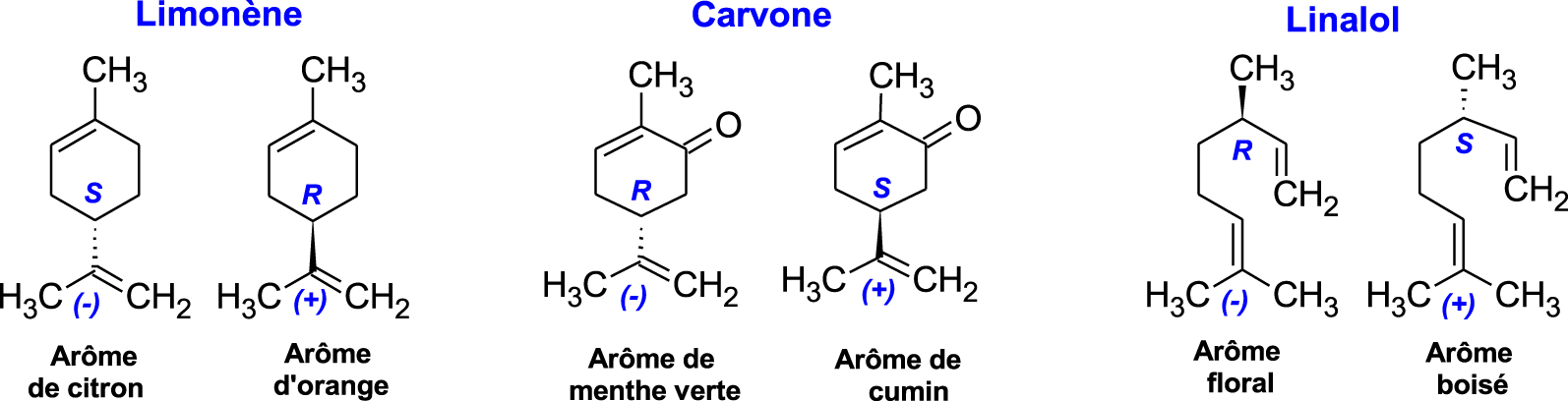
Aromas of the limonene, carvone, and linalool enantiomers.
With three asymmetric carbons (3C ∗) in the molecule, the mint family has eight stereoisomers (23). In nature, the most common enantiomer is(1R,2S,5R)-(−)-Menthol,[(1R,2S,5R)-5-methyl-2-(propan-2-yl)cyclohexanol]. It is the only one that possesses anti-inflammatory, antiviral, anaesthetic, pesticide, and disinfectant properties. It belongs to the family of monoterpenols and is naturally found in the essential oil of peppermint (Mentha piperita). Japanese menthol also contains a small percentage of (+)-Neomenthol[(1S,2S ,5R)-2-Isopropyl-5-methylcyclohexanol] (Figure 6). Chiral analysis of essential oil samples showing the presence of the (1S,2SR,5S)-(+)-Menthol enantiomer is evidence of adulteration.

Diastereomers and enantiomers in the mint family.
The example of rose essential oil, one of the most precious in aromatherapy and much appreciated by perfumers, is particularly interesting. Among its main components are citronellol [3,7-dimethyloct-6-en-1-ol] and rose oxides [4-methyl-2-(2-methylprop-1-enyl)oxane] which have highly specific enantiomeric profiles [50]. Rose oxide, the star molecule of many prestigious perfumes, has several diastereomers due to an endo/exo isomerism (position of the substituent on/under the ring) and the presence of two asymmetric carbons (Figure 7).

Diastereomers and enantiomers of the components of rose essential oil.
The other main component of rose oil that merits mentioning is citronellol, with more than 50% present in its enantiomeric form (S)-(−)-Citronellol. Its floral rose smell is reminiscent of geranium oil, while the aroma of (R)-(+)-Citronellol is similar to citronella (lemongrass) oil. Their olfactory thresholds are close to 40–50 ppb for the (S) enantiomer and 50 ppb for the (R) enantiomer [51, 52].
In the rose essential oil of Bulgaria and Turkey, the levorotatory enantiomers (2S,4R)-(−)-cis-rose oxide and (2R,4R)-(−)-trans-rose oxide have a high enantiomeric purity (>99.5%) [53, 54]. The presence of the (+)-cis-rose oxide and (+)-trans-rose oxide enantiomers allows for the identification of this essential oil as “unnatural“. Conversely, the two dextrorotatory enantiomers (2R,4S)-(+)-cis-oxide of rose and (2S,4S)-(+)-trans-rose oxide are detected with high enantiomeric purity in the essential oil of Melissa officinalis (lemon balm). They are indicators of the authenticity of this essential oil. The aromas and olfactory thresholds of these four enantiomers [55, 56, 57] are notable. They are described as follows:
- (4R,2S)-(−)-cis-rose oxide has a clear floral green note, light, green pink, diffusive, strong. It has been described as powerful fruity. Its olfactory threshold of 0.5 ppb is very low. It is the only enantiomer responsible for the typical rose fragrance.
- (4S,2R)-(+)-cis-rose oxide, with a floral note, green hay, earthy, heavy. With a 50 ppb olfactory threshold, it is odourless.
- (4R,2R)-(−)-trans-rose oxide has a green, minty, fruity note. Its olfactory threshold is 160 ppb.
- (4S,2S)-(+)-trans-rose oxide with a green vegetal note, fruitily floral, rose with herbs, citrus fruits (bitter bark). Its olfactory threshold is 80 ppb.
The perception of aromas is a highly complex mechanism that incorporates many factors, such as molecular structure, concentration, the release medium, and the olfaction mechanism, but also genetic differences in the expression of odour receptors. The scientific bibliography is very rich for the structure-odour relationship of chiral molecules [58, 59, 60, 61, 62, 63, 64, 65, 66, 67] and on the impact of the absolute configuration on the olfaction threshold of the enantiomers. The most striking example is that of nootkatone [68, 69], a sesquiterpenoid ketone whose dextrorotatory enantiomer (4R,4aS,6R)-(+)-Nootkatone has a strong grapefruit smell and an olfactory threshold of 800 ppb, whereas the levorotatory enantiomer (4S,4aR,6S)-(−)-Nootkatone has a strongly fresh, green, woody, and spicy scent, and an olfactory threshold of 600,000 ppb, 750 times higher!
The enantiomeric composition of the molecules of an essential oil constitutes its identity card. It makes it possible to specify its origin and its biological activity. The majority of molecules that constitute essential oils consist of one predominant enantiomer, or sometimes a mixture of the two. For the same species of plant, there is a high natural variability in the enantiomeric concentration of essential oils' constituents. This is due to various factors, such as environmental, genetic factors, growth conditions, harvesting, and distillation techniques. Chirality is a fundamental aspect of nature, even if the phenomenon is complex and sometimes difficult to identify [70].
5. Structure of the main chiral molecules constituting essential oils
The classification of essential oils by biochemical family is a prerequisite for understanding their therapeutic properties and making the best use of them safely. Chemistry alone is not enough to explain all of the properties, nor all of the potential toxicities of essential oils. Studying the biochemical activity of the main molecules individually helps to clarify the therapeutic effect, in particular according to the main enantiomers, for which recognition with a substrate is conducted based on the lock-and-key model. However, the effect of the essential oil collected in its entirety after a gentle, complete, and unfractionated distillation often turns out to be more significant, and may differ from that of the active ingredients taken in isolation.
The main chemical molecules that make up essential oils are terpene and sesquiterpene hydrocarbons, alcohols and esters, aldehydes, ketones, phenols, ethers and peroxides; the majority of these molecules are chiral and exist in the form of enantiomers or diastereomers. We will present the enantiomeric forms of some significant chiral molecules that compose the terpene hydrocarbons and sesquiterpenes most frequently found in essential oils (Table 1).
Terpene and sesquiterpene hydrocarbons and their chiralities
Acyclic | Nba | Monocyclic | Nb | Bicyclic | Nb | |
---|---|---|---|---|---|---|
C∗ | C∗ | C∗ | ||||
Monoterpenes and monoterpenoids | Linalyl acetate | 1 | 𝛼-Terpineol | 1 | Sabinene | 2 |
Lavandulyl acetate | 1 | Terpinen-4-ol | 1 | 𝛼-pinene | 2 | |
Carvone | 1 | 𝛽-pinene | 2 | |||
Camphor | 2 | |||||
Camphene | 2 | |||||
Sesquiterpenes and sesquiterpenoids | (Z),(E)-Nerolidol | 1 | Germacrene D | 1 | (E)-𝛽-caryophyllene | 2 |
a Number of C∗: asymmetric carbon in the molecule.
The stereochemistry of the enantiomers of all of the molecular structures in Table 1 is detailed in Figure 8. For molecules with a single asymmetric carbon, the two enantiomer forms are represented by: 𝛼-terpineol, terpinene-4-ol, carvone, lavandulyl acetate, linalyl acetate, and germacrene D. In the case of molecules with two asymmetric carbons, four stereoisomers are possible, sometimes less in the case of a bridge in the molecule. The geometry of one of the carbon atoms fixes that of the other carbon, thus there will be only two enantiomers. Such is the case for camphor, camphene, 𝛼-pinene, 𝛽-pinene, and sabinene.

Elements of chirality of terpene and sesquiterpene structures.
In addition to their asymmetric carbon, nerolidol and 𝛽-caryophyllene have a double bond (olefins) with Z∕ E conformations (cis/trans), non-chirotopic stereogenic elements; this allows for four possible stereoisomers for (Z,E)-nerolidol, including two pairs of enantiomers: [(E)-(R), (E)-(S)] and [(Z)-(R), (Z)-(S)]. In the case of 𝛽-caryophyllene, only the two E (or trans) form enantiomers are shown (Figure 8).
6. Enantiomeric profiles of selected essential oils
Even if the composition of the essential oil is largely determined by genetics and by climatic, geographical, and seasonal factors [71], it is accepted that from a specific source and under the same conditions, the enantiomeric composition of the main molecules of an essential oil will undergo little variation, making it possible to verify its authenticity. Some species produce the molecules as a single enantiomer while others produce both, either in mixtures enriched with one or other of the enantiomers or in racemic form (with the two enantiomers present equally 50/50).
Table 2 brings together the enantiomeric profile of some selected essential oils, including the chiral molecules previously presented: tea tree, spearmint, fine lavender, aspic lavender, lavandin, neroli, petitgrain bigarade, bitter orange, sweet orange, lemon, and clary sage. The enantiomeric distribution of each essential oil is limited to the three or four main chiral molecules whose presence is >1% and molecules pertinent for authentication (<1%); non-chiral molecules will also be mentioned. The structures of the enantiomers of the various terpene and sesquiterpene hydrocarbons concerned are represented in Figure 8, and the impact of the molecules' chirality on the biological activity of the essential oil is reported.
Analysis of Table 2 shows that all the essential oils described are predominantly composed of chiral molecules, with enantiomeric profiles characterised by a highly predominant enantiomer.
Tea tree essential oil (Melaleuca alternifolia) is the first one whose enantiomeric profile is now imposed by regulations. It has long been the subject of recurrent adulteration, either by mixing with other essential oils (pine, eucalyptus globulus, camphor, etc.) or via the addition of adulterating components [72]. This essential oil can have up to seven different chemotypes [73, 74], the best therapeutic qualities are attributed to the terpinen-4-ol type, which has been subjected to safety studies [75, 76].
The ISO 4730:2004 standard for this chemotype established a minimum of 35% terpinen-4-ol, 14% of 𝛾-terpinen, and 6% of 𝛼-terpinen [77]. The new version, ISO 4730:2017, is more strict; it integrates a chiral enantiomeric analysis by gas chromatography with enantiomeric ratios of (+)-Terpinen-4-ol/(−)-Terpinen-4-ol which vary between 63.3-69.8/36.7-30.2 and ratios of (+)-𝛼-Terpineol/(−)-𝛼-Terpineol which vary between 74.2-79.5/25.8-20.5 in authentic tea tree essential oil [78]. This enantiomeric profile makes it possible to detect the most frequent adulterations of this essential oil (Table 2, entry 1).
In spearmint essential oil (Mentha spicata var. crispa), the (R)-(−)-Carvone enantiomer, present at more than 87%, has a stronger antispasmodic effect than (S)-(+)-Carvone. These effects, described for both (R)-(−)-Carvone and spearmint oil, are explained as a (CCB)-type mode of action (Calcium channel blockers) [79]. (S)-(−)-Limonene is present at 98.8%, and pharmacological data indicate that this enantiomer could also be used in aromatherapy as an anti-anxiety agent [80].
Enantiomeric profile of the majority components of selected essential oils
Essential oil | Main molecules | %a | Enantiomeric profile of the majority components of selected essential oils | %b |
---|---|---|---|---|
Tea tree [81, 82] (Melaleuca alternifolia) | Terpinen-4-ol | 30–48 | (S)-(+)-Terpinene-4-ol | 74.2–79.5 |
𝛾-Terpinene | 10–28 | Non-chiral | — | |
𝛼-Terpinene | 5.0–13 | Non-chiral | — | |
𝛼-Terpineol | 1.5–8.0 | (R)-(+)-𝛼-Terpineol | 63.3–69.8 | |
𝛼-Pinene | 1.5–6 | (R,R)-(+)-𝛼-Pinene | 86–91 | |
Spearmint [83, 84, 85] (Mentha spicata) | Carvone | >40 | (R)-(−)-Carvone | 87.9 |
Limonene | >20 | (S)-(−)-Limonene | 98.8 | |
1.8-Cineole | 17 | Non-chiral | — | |
𝛽-Pinene | >2 | (S,S)-(−)-𝛽-Pinene | >52.1 | |
Fine lavender [86] (Lavandula angustifolia) | Linalyl acetate | 37–48 | (R)-(−)-Linalyl acetate | >99 |
Linalool | 19–32 | (R)-(−)-Linalool | >98 | |
𝛽-Caryophyllene | 3.7–6.3 | (R,S)-(−)-(E)-𝛽-Caryophyllene | >99 | |
Lavandulyl acetate | 2–11 | (R)-(−)-Lavandulyl acetate | >99 | |
Aspic lavender [87] (Lavandula latifolia) | Linalool | 34–50 | (R)-(−)-Linalool | 95 |
1.8-Cineole | 16–39 | Non-chiral | — | |
Camphor | 8–16 | (R,R)-(+)-Camphor | 95 | |
𝛼-Terpineol | 0.2–2 | (R)-(+)-𝛼-Terpineol | 56 | |
Limonene | 0.5–3 | (R)-(+)-Limonene | 95 | |
Abrial lavandin [86] (Lavandula hybrida var. abrial) | Linalool | 33–41 | (R)-(−)-Linalool | >95 |
Linalyl acetate | 23–32 | (R)-(−)-Linalyl acetate | >95 | |
Camphor | 9–11 | (R,R)-(+)-Camphor | >99 | |
1.8-Cineole | 6–10 | Non-chiral | — | |
(E)-𝛽-Caryophyllene | 1–2 | (R,S)-(−)-(E)-𝛽-Caryophyllene | >95 | |
Neroli [88, 89] (Citrus aurantium var. amara) flower | Linalool | 43–54 | (R)-(−)-Linalool | >70 |
Limonene | 6–10 | (R)-(+)-Limonene | >95 | |
Linalyl acetate | 3.5–8.6 | (R)-(−)-Linalyl acetate | >95 | |
E-Nerolidol | 2–6 | (S)-(+)-(E)-Nerolidol | >98 | |
𝛽-Pinene | 3–5 | (S,S)-(−)-𝛽-Pinene | >99 | |
Petitgrain bigarade [88, 89] (Citrus aurantium amara) leaves | Linalyl acetate | 40–80 | (R)-(−)-Linalyl acetate | >97 |
Linalool | 15–35 | (R)-(−)-Linalool | >80 | |
𝛼-Terpineol | 3–10 | (R)-(+)-𝛼-Terpineol | >72 | |
Bitter Orange [90, 91] (Citrus aurantium L var. amara) fruits | Limonene | 91–96 | (R)-(+)-Limonene | >99 |
𝛼-Terpineol | <3 | (R)-(+)-𝛼-Terpineol | >93 | |
Linalyl acetate | <1.5 | (R)-(−)-Linalyl acetate | >99 | |
𝛽-Myrcene | 1–2 | Non-chiral | — | |
𝛼-Pinene | <1 | (R,R)-(+)-𝛼-Pinene | >89 | |
Linalool | <1 | (R)-(−)-Linalool | >89 | |
Sweet orange [92, 93, 94] (Citrus sinensis L.) | Limonene | >96 | (R)-(+)-Limonene | >99 |
Linalool | <1 | (S)-(+)-Linalool | >87 | |
𝛽-Myrcène | Non-chiral | — | ||
𝛼-Pinene | <1 | (R,R)-(+)-𝛼-Pinene | >99 | |
Lemon [95, 91] (Citrus limon) | Limonene | 60–70 | (R)-(+)-Limonene | >98 |
𝛽-Pinene | 10–19 | (S,S)-(−)-𝛽-Pinene | >93 | |
𝛾-Terpinene | 7–9 | Non-chiral | — | |
Sabinene | 1.4–2.3 | (S,S)-(−)-Sabinene | >85 | |
𝛼-Pinene | 1.5–1.9 | (S,S)-(−)-𝛼-Pinene | >68 | |
Clary sage [96] (Salvia sclarea) | Linalyl acetate | 46.6 | (R)-(−)-Linalyl acetate | >99 |
Linalool | 17.1 | (R)-(−)-Linalool | >72 | |
Germacrene D | 11.8 | (S)-(−)-Germacrene D | 99.8 | |
(E)-ß-Caryophyllene | 4.9 | ns | — |
a Percentage of major components. b Percentage of majority enantiomer (for tea tree essential oil, these are the majority enantiomer margins required by the ISO 4730:2017 standard). ns: not specified.
The enantiomeric distribution of lavender essential oil is interesting; the (R)-(−)-Linalyl acetate and (R)-(−)-Linalool enantiomers predominate in fine lavender and hybrid lavandin with an enantiomeric excess of >95%. For aspic lavender, (R)-(−)-Linalool is also predominant at 95%, with an (R, R)-(+)-Camphor enantiomer present at 95%. These molecular markers can be used to establish the authenticity of these essential oils. Abrial lavandin, derived from the natural hybridisation between true lavender and aspic lavender, has a balanced composition with equally high enantiomeric profiles, analogous to those of true lavender (esters) and aspic lavender (camphor).
The Citrus genus is among the most popular natural essential oils and accounts for the majority of commercial natural aromas and fragrances [97]; the citrus enantiomeric profiles presented in Table 2 are noteworthy.
Neroli (Citrus aurantium amara leaf) and petitgrain bigarade (Citrus aurantium ssp. aurantium (fe)) oils [88, 89] are produced from different morphological parts of the bigarade orange (bitter orange) tree: neroli oil comes from the distillation of fresh flowers, and petitgrain oil comes from the distillation of leaves and twigs. However, one also can note differences in the distribution of the enantiomers of α-pinene and limonene molecules between these two essential oils, and also bitter orange essential oil (Citrus aurantium L var. amara), from the zest of the fruit of the same tree.
Neroli oil is rich in (S,S)-(−)-𝛼-Pinene, whereas in petitgrain oil (R,R)-(+)-𝛼-Pinene dominates. (R)-(+)-Limonene is the dominant enantiomer in both oils, even though in neroli oil (S)-(−)-Limonene is found in a smaller amount than in petitgrain oil. (S)-(−)-𝛽-Pinene is found in both oils with high enantiomeric purity.
The (S)-(+)-(E)-Nerolidol enantiomer is present between 2–6% with high enantiomeric purity (>98%) in neroli essential oil. It is an important sensory compound that impacts olfactory properties (soft, light, flowery) and acts as an indicator of the authenticity of this essential oil [88, 89]. The olfactory properties of its other stereoisomers have differing characteristics [98], listed here: R- (−)-(E)-Nerolidol has a pleasant, woody, warm, musty smell; the conformer (S)-(+)-(Z)-Nerolidol has an odour of woody bark, green and fresh; and that of (R)-(−)-(Z)-Nerolidol is intense, flowery, soft, and fresh.
The essential oil of bitter orange (Citrus aurantium L var. amara), from the zest of the fruit of the bigaradier, presents a very high enantiomeric excess (ee) for three components: (R)-(+)-Limonene (>99%), (R,R)-(+)-𝛼-Pinene with ee > 92%, and (S)-(−)-𝛽-Pinenewith ee > 97%. We find (R)-(+)-Limonene as a single enantiomer (>99%) in sweet orange (Citrus sinensis) and lemon (Citrus limon), as well as in Neroli (>95%).
Linalool is present (<1%) in the form of different enantiomers in the sweet orange and bitter orange essential oils. In sweet orange, (S)-(+)-Linalool is present at more than 82%, whereas in the bitter orange it is the (R)-(−)-Linalool that is more than 89%. The ratio of the enantiomers of linalool makes it possible to determine which are frauds that have added sweet orange essential oil, less precious, to the more expensive bitter orange essential oil [91].
Numerous studies attest to the relaxant properties of citrus essential oils due to their richness in (R)-(+)-Limonene. Bitter orange (Citrus aurantium L var. amara) relieves anxiety in patients after administration or inhalation [99, 100]. Inhalation of sweet orange oil (Citrus sinensis) reduces anxiety levels and improves the mood of patients while at the dentist's [101]. The study of the biological activity of limonene on the autonomic nervous system shows that the inhalation of (R)-(+)-Limonene has an effect on systolic blood pressure levels, alertness, and nervousness, whereas (S)-(−)-Limonene only increases systolic blood pressure and has no effect on other psychological parameters [102].
Clary sage essential oil, composed mainly of (R)-(−)-linalyl acetate (>99%) and (R)-(−)-linalol (>72%) enantiomers, provides in particular a spasmolytic and sedative effect [103], which could be a therapeutic agent for patients suffering from depression.
One can note that the enantiomeric profile of an essential oil is linked to a specific pharmacological activity that is differentiated according to enantiomers, which reinforces the importance of the identification of the enantiomeric profile of the essential oils.
7. Chiral analysis and identification of essential oils
The identification and quantification of the chemical components of essential oils is fundamental, and the difficulties of already complex analyses are significantly increased in the presence of chiral discrimination. Enantiomers have identical physicochemical properties, the same molecular masses, the same solubilities, and the same melting and boiling points, which makes them difficult to separate and identify. Enantiomers differ in their behaviour towards polarised light: one deflects it to the right and the other to the left. Moreover, the overall rotatory power of the essential oil has long been the only element of the technical information guide, allowing for an assessment of the overall chiral quality.
To guarantee the authenticity of essential oil batches and determine their therapeutic properties, two categories of quality control are required:
(1) Physico-chemical characteristics, including organoleptic quality controls and physical constants:
- Organoleptic controls: from an aroma library consisting of certified essential oils, we control the colour, smell, and (in some cases) flavour of the essential oils analysed.
- Study of physical constants at a given temperature: density, solubility in alcohol, melting and boiling points, freezing points, rotational power on polarised light, refractive index.
(2) Chromatographic, chiroptical, and spectroscopic identification methods:
Different approaches combining chromatographic separation and methods that allow for evaluating the enantiomeric excesses of chiral molecules in order to obtain a precise identification [104, 105, 106, 107], such as:
- Chiroptical techniques: Polarimetry, optical rotatory dispersion, circular dichroism.
- Isotopic analysis: allows for tracing origin by measuring the isotopic distribution in the compounds (proportion of 13C/14C, amount of 14C or 18O/17O).
- Infrared Spectroscopy and Nuclear Magnetic Resonance at high resolutions (400–700 MHz and multinuclear) with chiral displacement agents.
- X-Ray Diffraction.
7.1. Chiral chromatographic analysis
Chiral chromatographic analysis takes advantage of the very high resolving power of chromatography in order to separate the enantiomers using high-performance synthetic chiral stationary phases (CSPs), used in the gaseous, liquid, or supercritical phase. This is the most widely used method to assess the enantiomeric excess of the major molecules of the essential oils. The recognition mechanism on a chiral stationary phase is based on the different forces of interaction of the enantiomers, with a chiral selector fixed on a stationary phase allowing the formation of diastereomeric complexes eluted at different rates, often implicating several complementary techniques.
The difficulty of separating complex mixtures such as essential oils has led chemists to use mixed approaches, combining several methods to optimise identification. Chiral High Performance Liquid Chromatography (HPLC) with polarimetric detection makes it possible to easily distinguish the two enantiomers by measuring their positive peaks, and to quantify them precisely [108]. Another method, enantioselective multidimensional gas chromatography (enantio-MDGC) [109, 110, 111], has become established. Its use is favoured for a wide range of applications, including the separation of highly overlapping enantiomers and for the analysis of trace compounds, as in the case of essential oils.
Other types of couplings are used, with the objective of improving the analysis by combining either two chromatographic techniques or by coupling two spectroscopic techniques. In recent years, the coupling of a chromatographic technique individualising compounds with a spectroscopic identification technique has been developed. The most common couplings are:
- gas chromatography-mass spectrometry (GC-MS),
- high performance liquid chromatography-mass spectrometry (HPLC-MS),
- high performance liquid chromatography-proton nuclear magnetic resonance (HPLC-1H NMR).
The identification of the constituents is possible by comparison of the spectral data with those of reference products contained in spectral libraries.
8. Regulation and quality control of essential oils
The regulation [112] of essential oils is constantly monitored with periodic reassessments. For the legislator, this implies careful monitoring of their composition because of the potential risks they generate. Essential oils are subject to several legal regimes according to their use: cosmetic, food supplement, medicine, or biocide; their intended purpose determines the applicable regulations and the requirements that the product must meet.
The marketing of essential oils is supervised by several standards which do not guarantee their absolute purity, but rather a minimal level of quality. The standards include: AFNOR (Association Française de Normalisation), ISO (International Organization for Standardization) and CEN (European Committee for Standardization). Essential oils are defined by the standard NF EN ISO 9235 (2014), which specifies natural aromatic raw materials. The standards are very strict; they require traceability starting from the harvest of the plant, from the method of extraction to the quality control of the final product. The high variability in the chemical composition of essential oils (due to physiological and environmental factors) is one of the main limitations to their quality, safety, and effectiveness.
The enantiomeric profile of the essential oils is governed by the international standard ISO 22972:2004 (en) which specifies a general method of gas phase analysis on a chiral capillary column and determines the enantiomeric excess of the chiral compounds of which it is composed. Recently, the ISO 4730:2017 standard that specified the characteristics of tea tree essential oil, type terpinen-4 ol, has been the subject of an amendment (ISO 4730:2017 and addendum ISO 4730/A1:2018) [113]. This restriction specifies the enantiomeric profile required for terpinen-4-ol (corresponding to 67–71% of (S)(+)-Terpinen-4-ol and 29–33% of (R)-(−)-Terpinen-4-ol). These new features aim to guarantee its authenticity and its quality for safe use. They attest to the importance of the addition of the enantiomeric distribution criterion for tea tree essential oil.
We can confirm the absence of adulteration of an essential oil thanks to its enantiomeric profile. We can cite two examples, the cases of lemon balm (Melissa officinalis) and bergamot (Citrus aurantium). Lemon balm (Melissa officinalis) is one of the most expensive medicinal plants due to low oil yield. Most of the lemon balm oil sold on the market is adulterated, but it is possible to ensure the composition of this oil using the enantiomeric profile of the citronellal that composes it. Pure lemon balm oil predominantly contains the enantiomer (R)-(+)-Citronellal (98%), compared to (S)-(−)-Citronellal which is present at only 2%. An adulteration often used is the addition of less expensive essential oils that are rich in citronellal, such as Cymbopogon winterianus (lemongrass) oil or Eucalyptus citriodora. The proportion of enantiomers can change up to [(+)/(−)] [90% /10%]. Bergamot (Citrus aurantium) has a very distinct composition: the oil must contain only the (R)-(−)-Linalool and (R)-(−)-Linalyl acetate enantiomers. Their presence is easily verified by chiral chromatography.
9. The Linalool chemotype, an essential molecule in essential oils
Linalool (3,7-dimethylocta-1,6-dien-3-ol) is a tertiary alcohol. This monoterpenol is naturally present in almost all essential oils, in greater or lesser concentration. It is composed of two enantiomeric molecules present in different proportions in the plants. The enantiomers presented in Figure 5 have different scents: (S)-(+)-Linalool(coriandrol) has a sweet fragrance, very different from that of the woody aroma of (R)-(−)-Linalool [116]. Their olfactory detection thresholds are also different. The specific rotation of the enantiomers reported in the literature [117] is [𝛼]D = −20 and + 19.
Linalool is listed as a deodorant/perfume agent. Its origin is synthetic in many cosmetic products (60–90%) [118]. It is one of the most targeted allergens in our environment [119, 120], yet research results show that it is the oxidised form of the molecule that causes allergies. Linalool is considered to be a pre-hapten in that its oxidation by oxygen from the air leads to the formation of hydroperoxides [121], which potentiate its allergenic effect.
Linalool is the major component in a great number of essential oils in highly variable proportions and with unique enantiomeric profiles for each of them. The (R)-(−)-Linalool enantiomer is the most common in nature, but the enantiomeric distribution of the (R)-(−) and (S)-(+)-Linalool forms and those of linalyl acetate are useful indicators for monitoring the purity of several essential oils, including lavender, bergamot, and coriander [122].
A study on the enantiomeric distribution of linalool enantiomers in several essential oils was conducted [123, 124]; the main results are collated in Table 3. One can note that the (R)-(−)-Linalool enantiomer is very predominant in essential oils such as ho wood, (Cinnamomum camphora CT linalool) (>98%), pau-rosa [125] (Aniba rosaeodora) (>90%), basil [126] (Ocimum basilicum) (>99%), lemon mint (Mentha citrata) (>86%), or true lavender (Lavandula officinalis) (>97%). The (S)-(+)-Linalool enantiomer is found with very high enantiomeric excesses in the essental oils of, sweet orange (Citrus sinensis) (>94%), coriander (Coriandrum sativum) (>88%), ginger rosko (Zingiber roseum) roots/rhizomes (90.9%), false/rattan (Timut) pepper (Zanthoxylum armatum) (>92%), and Indian bay leaf (Cinnamomum tamala) (>99%). In the case of coriander essential oil, if the enantiomeric profile has less than 81% of (S)-(+)-Linalool, essential oil should be considered adulterated. For other essential oils, both enantiomers are present in nearly equal ratios, such as oregano, rose, geranium, lemongrass, lemon, and grapefruit.
Enantiomeric distribution of linalool in selected essential oils
(R)-(−)-linalool dominant | % | (S)-(+)-linalool dominant | % | Mixture of enantiomers | % |
---|---|---|---|---|---|
Essential oil of ho wood, Cinnamomum camphora CT linalool | >98 | Essential oil of sweet orange, Citrus sinensis (83.8%) | >94 | Essential oil of common oregano, Origanum vulgare | 44.5 (R) |
55.5 (S) | |||||
Essential oil of rosewood, Aniba rosaeodora | >90 | Essential oil of coriander, Coriandrum sativum | 88–90 | Essential oil of damask rose, Rosa damascena | 56.2 (R) |
43.8 (S) | |||||
Essential oil of basil, Ocimum basilum | >99 | Essential oil of ginger, Zingiber roseum roots/rhizomes | >90.9 | Essential oil of lemongrass, Cymbopogon flexuosus | 41.6 (R) |
58.4 (S) | |||||
Essential oil of bergamot mint, Mentha citrata | 86-88 | Essential oil of rattan/false pepper (Timut pepper), Zanthoxylum armatum, leaf | 92 | Essential oil of geranium, Pelargonium graveolens | 50.7 (R) |
49.3 (S) | |||||
Essential oil of true lavender, Lavandula officinalis | >97 | Essential oil of Indian cinnamon, Cinnamomum tamala | >99 | Essential oil of lemon, Citrus lime | Close to racemic |
with >99% | Essential oil of grapefruit, Citrus paradisi | [114, 115] |
9.1. Biological activity of linalool enantiomers
The influence of the chirality of the linalool molecule on its biological properties has been the subject of numerous studies [127]. Its use in drug delivery systems has been studied in 2018 [128] and confirms that its multiple benefits (anti-inflammatory, antimicrobial, antihyperlipidemic, antidepressant, neuroprotective and anticancer properties) depend mainly on the type of enantiomer, given that (R)-(−)-Linalool and (S)-(+)-Linalool are chemically distinct.
Inhalation of linalool by a group of humans exposed to a stressor caused physiological responses that depended on the enantiomer of the molecule: (S)-(+)-Linalool acts as an activator agent on arterial pressure and heart rate, while (R)-(−)-Linalool has an opposite effect on heart rate. Other results consider this enantiomer rather as an anti-stress agent [129, 130]. Lavender essential oil, composed of more than 97% (R)-(−)-Linalool, behaves in the same way, although the relaxation effect can be also influenced by its characteristic aroma [131, 132]. In humans, inhalation of lavender essential oil, composed of (R)-(−)-Linalool, causes sedation, relaxation, and reduced aggressiveness [133]. The study shows that this effect is dependent on the configuration of the molecule, but also the tasks assigned to the subjects. Inhalation of (R)-(−)-Linalool after listening to environmental sounds produces a sedative effect associated with a significant decrease in beta waves (14Hz waves, corresponding to routine activities), while after mental labor, inhalation of (R)-(−)-Linalool led rather to agitation and alertness, as well as an increase in beta waves.
In another trial, (S)-(+)-Linalool had a stimulating effect on heart rate, whereas (R)-(−)-Linalool had a sedating effect on heart rate [134]. In Brazil, the essential oil of rosewood (Aniba rosaeodora), with a (R)-(−)-Linalool content close to 90%, is used in traditional medicine as a sedative, anticonvulsant, and antidepressant [135]. These soothing virtues of essential oils would therefore be linked to the majority presence of the (R)-(−)-Linalool enantiomer.
These soothing virtues of essential oils would therefore be linked to the majority presence of the (R)-(−)-Linalool enantiomer. Linalool is a valuable molecule, with significant therapeutic potential. Its enantiomers constitute markers in the assessment of flavours and scents, but more especially as an element of authenticity control for essential oils. The enantiomeric profile or "enantiotype" is a reliable criterion for the evaluation of the origin and quality of the essential oil.
10. Conclusion
In this article, the importance of molecular chirality and its impact on the profile and biological activity of essential oils have been presented. The natural chiral signature of an essential oil "the enantiotype" is part of the continuity of the chemotype. It provides valuable guidance when it comes to single or multiple enantiomers within the same species, which can serve as a fingerprint when authenticity is a problem.
Systematic elucidation of the enantiomeric distribution of essential oils provides a reliable indication and a better visibility of species and their origins. Knowledge of the relative abundance of the correct enantiomeric composition of the main compounds should be part of the essential oil standardisation process. The enantiotype may be the best option in the face of the various regulations of several countries concerning the status of "natural" substances compared to "unnatural."
The configuration of the molecule is a key element controlling its interaction with its receptor that can be imagined as a key unlocking a lock. It consequently impacts the resulting biological and therapeutical responses. The insertion of this fundamental stereochemical aspect to scientific aromatherapy is necessary, for a safer use of essential oils and a better understanding of the mechanisms involved in the evaluation of pharmacological activity.
1Registration, Evaluation, Authorisation and restriction of CHemicals (REACH).
2Value of the olfactory perception threshold ppb: part per billion, the billion being the billion by volume.