Version française abrégée
Introduction
La zone non saturée du karst détermine le transit de pluie efficace vers la nappe et homogénéise le signal d'entrée. On peut différencier deux modalités d'infiltration : dans les drains (fort débit, courte durée), dans la matrice et les fissures (faible débit et vitesse). Dans le but de comprendre le rôle de la zone non saturée dans l'alimentation des aquifères karstiques, nous avons étudié le signal d'entrée (la pluie) et le signal de sortie (le suintement) dans la grotte de Nerja (Fig. 1).
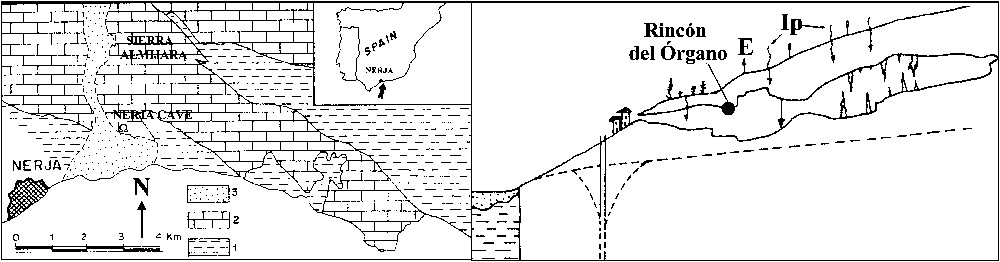
Location of the study zone and hydrogeologic scheme (not at scale). 1: Metapelites; 2: marbles; 3: postorogenic sediments; E: evaporation; Ip: infiltration (after [12]).
Localisation de la zone d'étude et schéma hydrogéologique (échelle non respectée). 1 : Métapélites ; 2 : marbres ; 3 : sédiments post-orogéniques ; E : évaporation ; Ip : infiltration (d'après [12]).
Caractéristiques géologiques et hydrogéologiques
Un aquifère karstique s'est développé dans des carbonates triasiques (Fig. 1). La grotte est située à une profondeur de 4 à 90 m [2]. Au toit des galeries, les points de suintement sont rares et à faible débit.
Méthodologie
Soixante-dix-neuf fractions de pluie ont été collectées (Fig. 1) entre 1995 et 1999. Le débit des eaux de suintement (stalactite située sous 30 m de marbres) est mesuré par un pluviographe ; 43 échantillons mensuels ont été pris.
Résultats
Pluviométrie et débit de suintement
Les 490 mm de précipitations annuelles sont concentrés entre octobre et février (Fig. 2). Deux épisodes majeurs ont été enregistrés (Fig. 3) : 428 mm (12/95–01/96) et 481 mm (12/96–01/97). Au point de suintement du Rincón del Órgano, considéré comme représentatif de la grotte, le débit moyen est de 89 cm3 j−1 [28]. La relation chronique de pluie–débit du suintement montre l'existence de deux modalités d'infiltration : l'une lente, au long de l'année, et l'autre rapide, qui ne se produit que de manière discontinue. L'évolution du débit de suintement (Fig. 3), avec des maxima d'été–automne et des minima d'hiver–printemps, reflète l'existence d'une circulation lente. L'utilisation des teneurs en K+ naturel comme traceur de l'infiltration a permis d'évaluer ce temps de transit [5,14] à 5–8 mois, (selon la hauteur et l'intensité de la pluie et selon le transit dans les fissures). Ce déphasage corrobore les résultats d'une analyse corrélatoire et spectrale effectuée sur les chroniques journalières précipitations/débit [4] : la zone non saturée a un temps de régulation de 85 jours, le déphasage entrée/sortie est de 6 mois pour des séquences à périodicité annuelle (Fig. 4). Pendant les périodes de recharge importante (début 1997), l'infiltration rapide dépend du montant de la recharge et de l'eau stockée dans l'épikarst avant l'épisode. Le fonctionnement est moins inertiel en année humide qu'en année sèche.

Mean monthly precipitation.
Précipitations moyennes mensuelles.

Temporal evolution of rainfall and drip water flow in the Nerja cave (Rincón del Órgano).
Évolution temporelle de la pluie et des suintements d'eau dans la grotte de Nerja (Rincón del Órgano).

Results of the correlatory and spectral analysis, with rainfall and drip flow rate. Hydrological years: 1993/94–1997/98. (A) Simple correlogram of drip discharge (short-term analysis, m=125 d, time step = 1 day); (B) simple spectrum of drip discharge (short term); (C) simple correlogram of drip discharge (long-term analysis, m=600 days, time-step = 5 days); (D) simple correlogram of dripping discharge for two contrasted rain value years (1: humid year, 858 mm; 2: dry year, 145 mm).
Résultats des analyses corrélatoires et spectrales réalisées sur les données de précipitations et débits de suintement recueillis à la grotte de Nerja, années hydrologiques 1993/94–1997/98. (A) Corrélogramme simple du débit de suintement (analyse à court terme, m=125 j, pas de temps : 1 j) ; (B) spectre simple du débit de suintement (analyse à court terme) ; (C) corrélogramme simple du débit de suintement (analyse à long terme, m=600 j, pas de temps : 5 j) ; (D) corrélogrammes simples du débit de suintement pour deux années hydrologiques à pluviométrie contrastée (1 : année humide, 858 mm ; 2 : année sèche, 145 mm).
Contenu isotopique de l'eau de pluie et de suintement
Le de l'eau de pluie varie entre et , son entre et , alors que dans le suintement, le varie de à , le de à . Le coefficient de variation des eaux de suintement est plus faible que celui de la pluie (Tableau 1).
Statistical parameters for the isotopic content (‰) of the analysed samples
Paramètres statistiques concernant la composition isotopique (‰) des échantillons analysés
Rainwater | Effective rainfall | Drip water | ||||||
d | d | |||||||
n | 79 | 79 | 79 | 47 | 47 | 47 | 43 | 43 |
max | 1.2 | 13.7 | 20.8 | −2.6 | −11.6 | 20.8 | −0.3 | 3.0 |
min | −10.4 | −69.4 | −4.8 | −10.4 | −69.4 | −4.8 | −5.1 | −32.9 |
med | −4.9 | −29.6 | 9.8 | −5.7 | −34.5 | 10.7 | −3.8 | −22.1 |
med p | −5.2 | −30.9 | 10.3 | −5.5 | −33.1 | 10.8 | −4.4 | −26.0 |
v (%) | 51.6 | 61.9 | 47.9 | 35.3 | 42.2 | 45.3 | 32.9 | 38.5 |
Interprétation des résultats des analyses isotopiques
Eau de pluie
Le diagramme (Fig. 5) montre que la pluie est alignée au voisinage de la droite météorique mondiale (LMM) [18], sauf certains échantillons évaporés (pente 5,5, A sur la Fig. 5), qui n'engendrent pas d'infiltration (selon calcul par bilan de Thornthwaite avec RFU = 25 mm) [28]. L' « excès » en deutérium ( à ) démontre la prédominance atlantique des précipitations [3,19]. Les pluies des mois chauds (Fig. 6) ont un (faible hauteur et température élevée) [20,32,34]. En mai (grande lame d'eau, Fig. 6), les valeurs de de la pluie sont . Il en est de même pour les précipitations des mois froids, appauvries .

diagram of rainwater and drip water obtained from the Nerja cave. LMM: World meteoric water line, A: evaporation line for rainwater, B: evaporation line for drip water.
Diagramme de la pluie et des suintements d'eau dans la grotte de Nerja. LMM : Droite météorique mondiale, A : droite d'évaporation de l'eau de pluie, B : droite d'évaporation de l'eau de suintement.

Mean values of monthly precipitation and isotopic content of rainfall during the study period.
Valeurs moyennes des précipitations mensuelles et composition isotopique de la pluie pendant la période d'étude.
Eau de suintement de la grotte
Les échantillons s'alignent sur la LMM (origine météorique) [13,14] ; il apparaît des échantillons évaporés (débits ), qui s'ajustent sur une pente 5,5 (B sur la Fig. 5). La composition du suintement est plus homogène que celle de la pluie. Les eaux les moins négatives sont observées pendant l'hiver et le printemps, les plus négatives pendant l'été et l'automne (Fig. 7). La teneur moyenne du suintement pondéré par son débit est moins appauvrie que celle de la pluie efficace pondérée par sa hauteur (, Tableau 1). Cet enrichissement est attribuable à l'évaporation (sol, épikarst et intérieur de la grotte), surtout pour les débits très faibles (temps de contact prolongé avec l'atmosphère de la cavité).

Temporal evolution of the in rainwater and in drip water inside the Nerja cave.
Évolution temporelle de dans l'eau de pluie et dans l'eau de suintement à l'intérieur de la grotte de Nerja.
Flux d'eau à travers la zone non saturée
Les pluies d'octobre à février engendrent l'essentiel de la recharge. Sur la Fig. 8, le 18O révèle un flux d'entrée en novembre–décembre. Les maxima de flux de sortie dans la cavité s'observent en août–septembre, et occasionnellement à des périodes (décembre–mars) associées à des épisodes pluvieux exceptionnels (fin 1996–début 1997). La relation entrée/sortie du montre un déphasage de 8 mois (1 sur la Fig. 8). Ce temps nécessaire à traverser 30 m de zone non saturée par un écoulement de type piston suppose une matrice à faible teneur en eau, résultat cohérent avec la teneur en eau mesurée de 0,37%, moyenne de 27 mesures sur échantillons de marbre prélevés dans une carrière située au voisinage. Cependant, lorsqu'une recharge significative a lieu, le flux de sortie répond avec un déphasage n'excédant pas 1 à 3 mois (2 sur la Fig. 8).

Fluxes of 18O stored through the unsaturated zone of the Sierra Almijara carbonate aquifer. 1: slow flow, 2: rapid flow.
Flux de 18O à travers la zone non saturée de l'aquifère carbonaté de la Sierra Almijara. 1 : flux lent, 2 : flux rapide.
Conclusions
La grotte de Nerja est située dans la zone non saturée d'un karst [2], épaisse de 4 à 90 m. L'étude des isotopes stables a démontré que les pluies hivernales, qui engendrent la recharge, sont plus pauvres en isotopes lourds, alors que les pluies estivales, moins abondantes, sont plus enrichies. À l'intérieur de la grotte, le débit des points de suintement est plus fort pendant les mois d'été–automne que pendant ceux d'hiver–printemps, à l'exception des pluies très intenses. Les teneurs de l'eau de suintement sont appauvries pendant les mois d'été–automne, et sont plus riches en hiver–printemps (déphasage entre l'entrée et les suintements). Il existe une différence significative entre la teneur isotopique moyenne de la pluie et celle des eaux de suintement, due à l'existence de processus évaporatoires (épikarst, zone non saturée et intérieur de la cavité). Il existe deux modalités d'infiltration, l'une lente et l'autre rapide. L'infiltration lente, qui prédomine toute l'année, se produit dans les petites fissures de l'épikarst et de la zone non saturée, ce qui permet accumulation de l'eau, existence de processus d'évaporation, et homogénéisation des flux d'infiltration. L'infiltration rapide se produit de manière localisée dans le temps, en réponse à des précipitations importantes. La comparaison des maxima de flux d'entrée et de sortie en démontre que la circulation lente a un temps de transit de 8 mois, la circulation rapide de 1 à 3 mois. L'approche isotopique valide les résultats de l'hydrodynamique et l'hydrogéochimie. Ces résultats rappellent l'existence d'un réservoir permanent dans la zone dite « non saturée » d'un système karstique, avec un temps de séjour moyen plurimensuel, qui contribue au débit des sources karstiques.
1 Introduction
Understanding the unsaturated zone of carbonate aquifers is of great importance, because it determines the passage of water towards the aquifer, and is involved in karstification processes and in the types of water flow that may occur. Moreover, as water flows through this zone towards the inner part of the system, it is mineralised and the input signal is homogenised, i.e. the chemical and isotopic differences of the rainwater are reduced.
Two types of infiltration can be distinguished in the unsaturated zone of carbonate aquifers: the first of these corresponds to the circulation of water through karst conduits, which ensures the rapid transit of water towards deeper zones, with relatively high, but short-lived flow volumes. The second type features the slow circulation of water through the matrix bedrock and fissures, with a small flow volume and a low velocity.
Karst aquifers present an organised structure comprised of different types of drainage [6,30], in which caves may be formed. Such caves can give rise to significant volumes of water flow. The evolution of a karstic structure over time leads to some drains losing their function and to caves being located in the unsaturated zone [23,25]. These caves are subjected to the two types of infiltration described above and are therefore excellent sites for studying behaviour patterns of the unsaturated zone of carbonate aquifers and the transit time of rainwater.
Different methods may be used to study the unsaturated zone of karstic aquifers [6], one of which is the analysis of the isotopic composition of infiltration water. The stable isotopes of water constitute natural tracers that are widely applied in karstic hydrogeology [8,11,19,27,31,36]. Caves are privileged sites for palaeoclimatic investigations [24,33] (their speleothems having recorded thermal and hydric variations of the last millennia), but also for observation of the behaviour of the unsaturated zone of carbonate aquifers and of residence time of seepage water. The isotopic content of drip water in caves has been the object of various studies. Yonge et al. [37] analysed the stable isotopes of the drip water within numerous caves in the USA, and Bakalowicz and Jusserand [7] used this methodology to investigate infiltration into the karstic massif of the Niaux cave (French Pyrenees), while Caballero et al. [13] obtained the first isotopic characterisation of the drip water within the Nerja cave (Spain).
The objective of the present study is to determine the functioning of the unsaturated zone of a carbonate aquifer by examining the stable isotopes of rainwater (input signal) and of the drip water within a cave situated in the unsaturated zone of the aquifer (output signal). The experimental area is the Nerja cave (Fig. 1), located in the southern part of Sierra Almijara (southern Spain).
The Nerja cave has been the object of palaeoclimatic reconstructions [26], and of earlier hydrodynamic studies relating the volume of precipitation to that of seepage within the cave [4,28] and of hydrochemical studies comparing the natural K+ content of rainwater with that of the drip water [5,14]. This monitoring was performed during a long period (03/1991–11/1999). It was found that the unsaturated zone of the cave behaves like an inertial system with a long transit time. We now address this question by means of an isotopic study of the two water types.
2 Geologic and hydrogeologic setting
The materials outcropping in the region (Fig. 1) correspond to the Almijara Unit of the Alpujárride Complex of the Betic Cordillera. The lithologic sequence is made of Palaeozoic metapelites and of a Middle to Upper Triassic carbonate group of dolomitic and calcareous marbles. These marbles, which are permeable by fracturing and karstification, comprise the carbonate aquifer of Sierra Almijara, the recharge of which is mainly due to the infiltration of rainwater.
Today, the Nerja cave is located in the unsaturated zone of the aquifer [2], over 10 m above the piezometric level. The thickness of the marble above the cavity ranges between 4 and 90 m. Rainwater infiltrates through fissures and diaclases in the marble, and seeps through the roof of the cave. There are few drip points, and the water volume is generally very low.
3 Methodology
Rainwater was collected at the Nerja cave, situated 165 m a.s.l., at a distance of some 500 m from the Mediterranean Sea. The volume of precipitation was measured using the raingauge installed in the weather centre, and the isotopic study was performed using 79 samples, obtained during the main rainy periods between December 1995 and December 1999. The water in the unsaturated zone corresponds to a drip point in the cave (Rincón del Órgano), above which the marble is approximately 30 m thick. The seepage volume was determined by means of a pluviograph installed within the cave. We analysed 43 samples of water obtained at monthly intervals between December 1995 and December 1999.
The isotopic analyses were carried out at the Stable Isotope Laboratory of the Universidad Autónoma (Madrid) and at the Stable Isotope Laboratory of the Consejo Superior de Investigaciones Científicas (Granada). The 18O was determined using the method based on the CO2–H2O equilibrium at 25 °C [22] and the 2H was measured from the reaction of the water with Zn at 550 °C [17,35].
4 Results
4.1 Rainwater and drip water flow
The mean precipitation in the area is 490 mm yr−1, with a distinct wet season between October and February and a dry season during the summer months (Fig. 2).
Two periods of significant rainfall occurred during the study period (Fig. 3); the first of these was during December 1995 and January 1996, with 428 mm of precipitation, thus ending a long period of drought in the region. The second episode of considerable rainfall took place during December 1996 and January 1997, with a total precipitation of 481 mm. From this date until the end of the study period, there has been a pattern of declining precipitation.
There are few drip points in the Nerja cave and the drip water flow is, in general, very low. At a point considered representative of the cave (Rincón del Órgano), the mean drip water flow has been calculated as amounting to 89 cm3 d−1 [28]. The thickness of the unsaturated zone situated above this dripping point is about 30 m. The relation between evolution of rainfall and drip discharge versus time displays two infiltration modalities through the epikarst and the unsaturated zone: a slow infiltration, which predominates along the year, and a rapid infiltration, which occurs during short time periods.
Taking into account the yearly distribution of rainfall, the general evolution of the drip water rate (Fig. 3), with maximum values during summer and autumn, and minimum ones during winter and spring, reveals the existence of a slow rainwater flow from its input within the aquifer until its output at the dripping points of the cave. Using natural K+ contents as a tracer of seepage water enabled to compute approximately this transit time [5,14]: 5 to 8 months, depending on the value and intensity of rain, which fell before, during and after the K-rich rainfall, and from the pathway that is followed through the fissures of the marble. This seasonal outphasing is corroborated by results of a Correlatory and Spectral Analysis performed with daily precipitation and discharge data, corresponding to five hydrologic years [4]. These demonstrate that the unsaturated zone of the Nerja cave displays a very inertial behaviour; it takes much time to respond to a rain input, and has a high modulating power of the input. The memory effect is very high (about half a year), the regulation time is long (85 days), the spectral band is narrow, with a cut-off frequency of 0.10 (10 days) and the input/output outphasing is about 6 months for sequences of yearly periodicity (Fig. 4). The quantity of water that is stored within the epikarst before precipitations plays a prominent role in the hydrodynamic functioning of the system, which displays a less inertial behaviour during humid years than during dry ones.
During high-recharge periods, both in terms of quantity and of intensity (e.g., at the beginning of 1997), we can observe the existence of a rapid infiltration, which is demonstrated by a clear and rapid increase of the drip flow rate within the cave, whose magnitude depends on the amount of the recharge, but also on the water volume that is stored within the cave epikarst, before the rainfall.
The maximum values for drip water flow are recorded during summer and autumn, and minimum values correspond to winter and spring (Fig. 3). This observation confirms the slow circulation of rainwater through the unsaturated zone, thus producing a seasonal lag from when the water enters the aquifer until it appears at the drip points. During the significant recharge periods (e.g., early 1997), the increase in drip water flow occurs sooner; the magnitude of this increase depends on the quantity of the recharge and on the quantity of water stored in the epikarst of the cave before the precipitation occurs.
4.2 Isotopic content of rain and drip water
Table 1 shows the results of the isotopic contents obtained from the analysed samples. Results are expressed as parts per thousand of the internationally accepted standard deviation [18]. The analytical error was for the and for the .
The of the rainwater ranged from −10.4 to , with a mean value of , while the ranged from −69.4 to , with a mean value of . In the drip water, the measured was between −5.1 and , with a mean value of , while the ranged from −32.9 to , with a mean value of . The variation coefficient of the isotopic composition of drip water was lower than that observed in the rainwater samples.
5 Interpretation of the results of isotopic analysis
5.1 Rainwater
By plotting the results obtained on a graph, we see that the rainwater samples analysed are aligned according to the world meteoric water line (LMM) established by Craig [18], except several clearly evaporated samples, which fit a straight line with a slope of 5.5 (A in Fig. 5), and that they do not produce any effective infiltration in the aquifer, as it has been estimated from computation of effective rainfall by the Thornthwaite method with a daily time-step, with a 25-mm soil reserve [29]. The deuterium excess (, [20]) is a characteristic parameter of the source area of the water vapour from which rainfall originates. In the study area, this parameter varied from −4.8 to , with a mean value of . These data reflect the fact that precipitation of both Atlantic and Mediterranean origin falls on the region, although most rainfall is of Atlantic origin, as has been shown by various authors [3,19].
In general, the rainwater samples corresponding to the warmest months (Fig. 6) present less negative values of (with figures higher than ) and , due to the low quantity of water collected and to the higher air temperature. Thus, the rainwater drops tend to evaporate during their descent through a very dry atmosphere; there is also an enrichment of the isotopic content, depending on the quantity effect and on the seasonal effect [20,32,34]. On the contrary, during the winter months (and also in May), rainfall presents more negative values. A similar distribution was obtained by Celle et al. [15,16] for precipitation in the Avignon region (southern France). In May, due to the higher rain value that has been recorded (Fig. 6), the figures of rainwater are more depleted, lower than . In addition, precipitations of colder months are more depleted in heavy isotopes, with figures lower than .
5.2 Drip water within the cave
The drip water samples fit well to the world meteoric line (LMM), which reveals their meteoric origin, as reported by Caballero et al. [13] and Carrasco et al. [14].
As in the case of rainwater, evaporated samples appear clearly; they fit on an evaporation line with a slope of 5.5 (B in Fig. 5). In every case, these are samples with a very low drip flow rate, lower than 19 cm3 d−1. The isotopic composition of the drip water in the cave is more homogeneous than that of rainwater. Moreover, the highest isotopic contents recorded for the drip water correspond to the samples obtained during winter and spring, while the most negative values for the isotopes correspond to the samples obtained during summer and autumn (Fig. 7).
At other sites, the mean value for the of the drip water in a karstic cave has been utilised as the mean value of the rainfall in the area in which the cave is located [37]. In the present study, however, the mean value obtained for within the cave is less negative than that corresponding to rainwater . A similar result is obtained when comparing the average isotopic composition of drip water with the drip water flow and the weighted rainfall composition, weighted with the quantity of precipitation , although in the latter case the difference is smaller. This isotopic enrichment, from when the rain falls onto the cave exterior until its appearance as seepage inside the cave, is related to evaporation processes within the soil and in the epikarst.
On the contrary, the differences are more marked if we compare the average of the effective rainfall with that of dripping water (Table 1). This isotopic enrichment can be attributed to water enrichment in the soil, in the epikarst and inside the cave (the clearly evaporated samples correspond to low drip flow rates, in order that the drop of water remains longer in contact with the cave atmosphere). Nevertheless, evaporation measured at the drip point is lower in summer than in winter, and the differences are tiny, about 0.2 mm d−1. A larger difference exists in evaporation at the ground surface (1–6 mm d−1), demonstrating that the isotopic enrichment should mainly occur within the epikarst. Moreover, a slight modification of the isotopic composition can occur inside the cave; it is accentuated in some samples taken during low-discharge periods.
5.3 Water flow through the unsaturated zone
The flow of 18O through the unsaturated zone of the aquifer was determined (Fig. 8). The input flow was calculated from the effective rainfall (Thornthwaite's method, daily time step, with a 25-mm soil reserve), multiplied by . In general, the winter rainfall episodes are the ones that produce recharge, and so the isotopic inputs to the unsaturated zone are between October and February, together with those that occasionally occur in the spring (April and May). In Fig. 8, we have represented the 18O fluxes of the input, gathered by rain periods (assigning the obtained sum to the centre of gravity of the period) in order to obtain the time distribution of the major recharge episodes of the aquifer. As a general case, we observe the existence of an input flux to the aquifer, during November and December.
The output flow of 18O into the cavity was determined by multiplying the drip water flow by its value. Maximum values are obtained in summer and autumn (August and September), and occasionally in other periods (December–March) associated with significant rainfall episodes (e.g., end of 1996 and early 1997).
The 18O input/output ratio (Fig. 8) for rainwater and drip water reveals the existence of a seasonal lag of about 8 months (1 in Fig. 8) between the input of rainfall and the output of seepage within the cave. This lag, observed for a 30 m flow through the unsaturated zone, implies a low moisture content in the limestone matrix, according to the piston flow model applicable to this medium. This result is consistent with the average of moisture content of 27 marble samples taken in a quarry situated in the vicinity of the Nerja Cave: 0.38%, measured by Prof. J. Motyka at the University of Krakow (Poland). Nevertheless, when an important recharge episode occurs (such as in early 1997), the drip water response is faster, the lag never exceeding 1 to 3 months (2 in Fig. 8).
According to the high Total Organic Carbon (TOC) contents measured in the soil water situated above the cave, the TOC range observed in the dripwater (0.95–5 mg l−1, average: 2.23 mg l−1) demonstrates that the drip cannot be several years old, at most several months [1,9,10,21].
6 Conclusions
The Nerja cave is currently situated within the unsaturated zone of a carbonate aquifer which is made of fissured and karstified dolomitic marble [2]. The thickness of the unsaturated zone which is situated above the cave, very variable, ranges between 4 and 90 m.
Our study of the stable isotopes of the water molecule in this southern Spain region, under a Mediterranean climate, reveals that the isotopic content of rainwater varies during the hydrologic year. Thus, the rainfall in winter, which is normally the most abundant, tends to present the lowest isotopic content, whilst the scarce rainfall in spring and summer is more enriched in heavy isotopes. The main rainfall episodes, which induce a recharge of the aquifer, are recorded during November–December, though it is possible to observe occasionally heavy rainfalls in the spring.
Inside the cave, dripping points exist. Their discharge is generally higher during the summer months than during those of winter and spring, excepting several very intense rainfalls that induce a fast increase of the drip flow rate. The isotopic contents of drip water are depleted during summer and autumn, and more enriched in winter and spring. A seasonal lag has therefore been observed between the entry of rainwater into the aquifer and its appearance at a drip point inside the cave. A significant difference exists between the average isotopic content of rainwater and that of dripwater, thanks to the existence of evaporation processes, as much within the epikarst-unsaturated zone complex, as inside the cave.
In the Nerja cave, two infiltration modalities exist, one is slow, the other is rapid. Slow infiltration, which prevails all around the year, occurs in thin fissures of the epikarst-unsaturated zone, which enables water storage and evaporation, and also a homogenization of the downward migrating fluxes of seepage water. Rapid infiltration occurs during short time periods along the year, as a response to heavy rainfalls characterizing the Mediterranean climate, which induces a higher velocity flow in more open fractures.
Comparing the maxima of input and output 18O fluxes has demonstrated that the slow flow across the epikarst-unsaturated zone takes about 8 months, whilst the rapid one takes 1 to 3 months, for a thickness of 30 m of the epikarst–unsaturated zone complex. This aspect, already displayed with hydrodynamics and hydrogeochemistry, is validated by the isotopic approach. These results demonstrate the existence of a permanent water reserve within the so-called ‘unsaturated zone’, with a pluri-monthly residence time, which contributes to the discharge of karstic springs.
Acknowledgements
This research has been supported by the Nerja Cave Foundation and it is a contribution to the projects CGL2005-05427 of the DGI, IGCP-513 of the UNESCO and to the Integrated Action HF02-0158. It was carried out by the RNM-308 Research Group of Junta de Andalucía. We also acknowledge Prof. J. Motyka, from the University of Krakow (Poland) for petrophysical measurements, Prof. Ghislain De Marsily and Prof. Bernard Blavoux for their suggestions to improve the manuscript.