1 Introduction
From the Late Triassic to the Cenomanian times, North Africa was characterised by an extensional regime associated with both the opening of the central Atlantic [9,31] and northward drift of the eastern part of the Apulian block [11,13,21,34].
Otherwise, the Mid-Cretaceous is thought to be characterized by a decrease in the tectonic activity and the most intense Phanerozoic flooding event as the Latest Cenomanian eustatic transgression [14,15,36] concomitant to the fast Atlantic seafloor spreading. Rifting occurred along the northern margin of the African plate as a result of left-lateral movement between the African and Eurasian plates, resulting in a complex horsts and grabens system trending NW to SE (Fig. 1) [13,19,21]. Associated fault displacements and uplift of horst blocks controlled sedimentation [21,22,26].
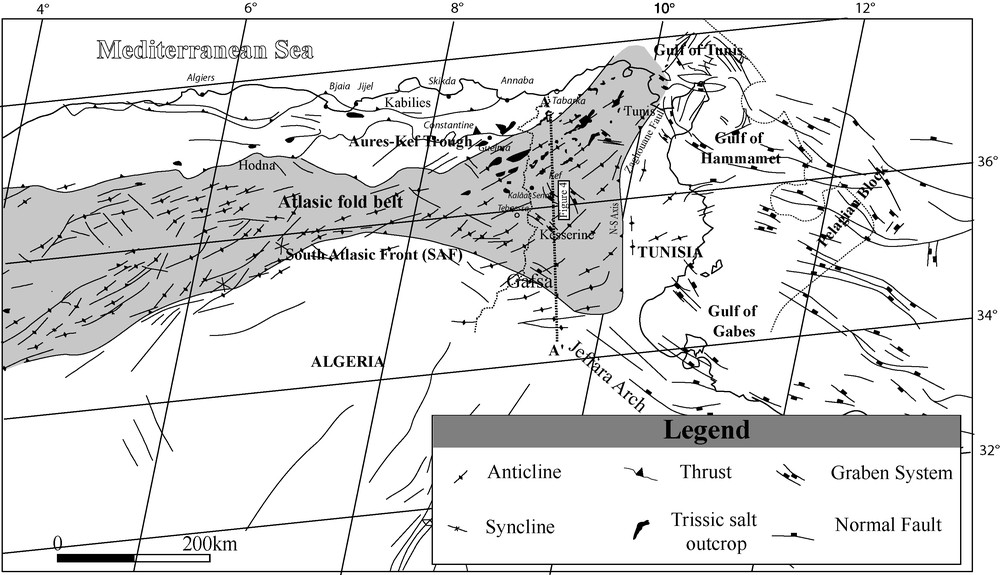
Tectonic map of the eastern Maghrebian Domain, age and nature of the main structural elements across North Africa. The outline of the major Mesozoic basins is well reflected in the pattern of subcropping stratigraphy modified from [5,10,13,16,30].
Carte structurale du domaine maghrébin oriental, âge et nature des principaux éléments à travers l’Afrique du Nord. L’esquisse des principaux bassins mésozoïques est très connue en subsurface, modifiée de [5,10,13,16,30].
Compiling different data including seismic, sedimentological cross sections from petroleum wells or from outcrops integrated with previous works, we tentatively present an isopach paleogeographic map of the Cenomanian-Turonian (C/T) transition in the Maghrebian western Atlas basins in Tunisia and eastern Algeria (Fig. 3).

Facies and thickness of the organic-rich Late Cenomanian-Early Turonian and age equivalent units in Tunisia and eastern Algeria superposed on the structural setting of the eastern Maghrebian Domain during the C/T transition (inferred from the palaeogeographic map). Note the east-west trending faults are related to the Tethys rifting and the NW-SE trending faults are related to the Sirte rifting ([10] and references therein). Base map and isopach data modified after [2,24,32,33,36]; facies zones modified after [2,24,36]. Note that the isopach trends illustrated are simplified and short-distance thickness, and facies changes occur, related to the complex tectono-halokinetic palaeorelief at the time (Fig. 4). Organic-rich and carbonate or age equivalent units are not present on present palaeohigh.
Faciès et épaisseur des sédiments riches en matière organique du Cénomanien-Turonien et leurs équivalents latéraux en Tunisie et en Algérie orientale, superposés sur le cadre structural du domaine maghrébin oriental durant le passage C/T (déduit de la carte palégéographique). Noter que les failles de direction est-ouest sont liées au rifting Téthysien et que les failles de direction NW-SE sont liées à l’ouverture de bassin de Sirte (ex. [10] et les références citées dans cet article). La carte de base et les isopaques sont modifiés de [2,24,32,33,36] ; les zones de changement de faciès sont modifiées de [2,24,36]. Notez que les isopaques figurés sont simplifiés et que les changements de faciès et d’épaisseur à courte distance sont liés au paléorelief complexe tectono-halocinétique (Fig. 4). Les sédiments C/T ne sont pas présents sur les paléo-haut fonds actuels.
Structurally and in a simplified manner, Tunisia and Algeria are composed of the Atlas Fold-and-Thrust belt to the north and the Saharan Platform, south of the South Atlas Front (SAF) (Fig. 1). They can be subdivided into: (1) a northern alpine-deformed subsident zone with thick Mesozoic and Cenozoic strata; and (2) a stable Palaeozoic province to the south where Middle Jurassic-Late Cretaceous is relatively thin [2,3,36]. In general, Cretaceous sedimentation on the Saharan Platform has been poorly affected by tectonic movements [4], with the exception of local strike-slip movements (Fig. 1). In the Atlas belt, however, deposition was strongly influenced by synsedimentary extensional phases.
2 Structural setting
The C/T organic-rich strata in onshore Tunisia/Algeria and offshore Tunisia are mainly found in the first province and the distribution of the organic content is strongly controlled by halfgraben systems [1,12,19,27,36] (Fig. 4), and in some areas by Triassic salt diapiric movements [28,30,36] during the Cretaceous (Figs. 4 and 5).
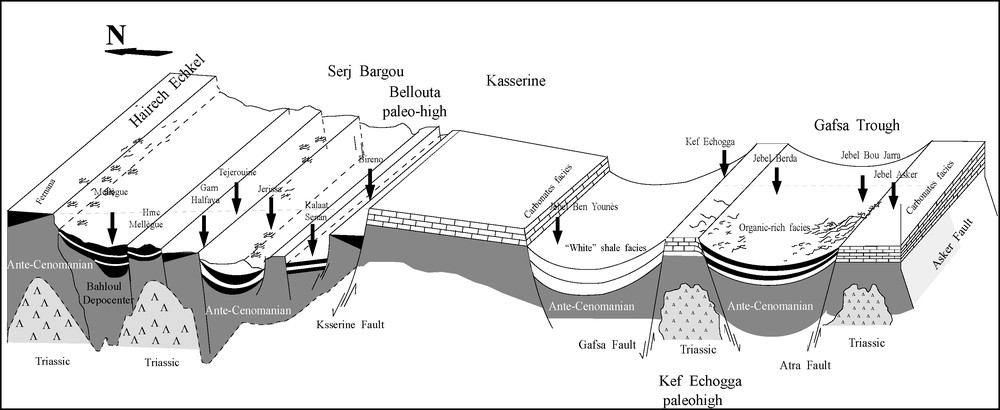
The Cenomanian-Turonian sedimentation evolution amplified by Triassic diapirism creating subsident rim-synclines allowing stratified water conditions and high preserving sedimentation rates that occurred later during Upper-Cretaceous. North-south cross-sectional model along Mellegue-Kasserine-Gafsa trough. Data compiled after [1,28,32,33,36]. Note that Mellegue recorded 80 m thick laminated black shales and is considered as the Tunisian depocenter of the Cenomanian-Turonian sedimentation (lateral and vertical scale not considered).
Évolution de la sédimentation du Cénomanien-Turonien amplifiée par le diapirisme triasique créant des rime-synclines subsidents permettant la stratification de la colonne d’eau et la préservation de sédiments riches en MO qui seront déposés ultérieurement au cours du Crétacé supérieur. Coupe transversale le long d’un transect nord-sud : Mellègue-Kasserine-Sillon de Gafsa. Les données sont compilées d’après [1,28,32,33,36]. Notez que la région du Mellègue a enregistré 80 m d’épaisseur de black shales feuilletés, riches en MO ; celle-ci est considérée comme le dépocentre tunisien de la sédimentation C/T.
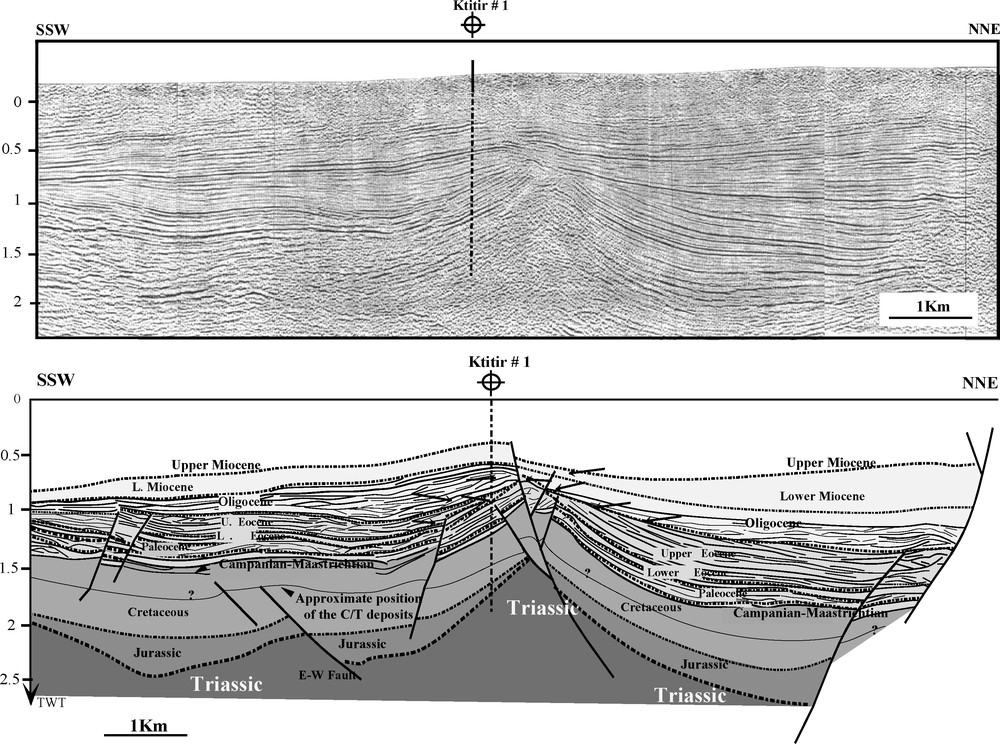
Seismic interpretation in the Sahel foreland of the Atlas outlining Triassic salt movements linked to Tethyan Late Jurassic-Early Cretaceous rifting and subsequent thickening of the Cretaceous series in rim-synclines (adapted from Khomsi et al. [21]). Note that C/T evolution is influenced by Triassic diapirism creating subsident rim-synclines allowing stratified water conditions and preserving high sedimentation rates that occurred later during Upper-Cretaceous. In this figure, an attempt was carried out to draw the approximate C/T organic-rich sediments.
Interprétation sismique dans le Sahel, avant-pays de l’Atlas, mettant en relief d’une part les mouvements salifères du Trias liés au rifting Téthysien du Jurassique supérieur-Crétacé inférieur et d’autre part, l’épaississement du Crétacé dans les rim-synclines. (adapté de Khomsi et al. [21]). Notez que l’évolution de la sédimentation C/T est influencée par le diapirisme qui a créé des rim-synclines subsidents, permettant des conditions de stratification de la colonne d’eau et la préservation de forts taux se sédimentation qui se sont produits plus tard pendant le Crétace supérieur. Sur la figure, on a tenté de dessiner la position approchée du sédiments C/T, riches en matière organique.
The rift grabens formation is related to the opening of the Neotethys to the north [12] and central Atlantic to the southwest [29].
Notably, this rifting in onshore and on the shelf of Tunisia/Algeria did not begin before the Late Jurassic-Early Cretaceous [12,36,37,39]. However, the Late Triassic-Early Jurassic rifting is in fact associated with the separation of the eastern part of the Apulian micro-plate block [9,34] related at that time to the northern African Plate. Alternatively, in Morocco, during this period, related synrift sedimention consisted mainly of Jurassic carbonates and Early Cretaceous deltaic clastics where syndepositional rollover structures are developed [17,23] which might also have locally affected the anoxic C/T interval deposition.
So, two main Mesozoic rifting events occurred in this northern African margin (i.e. Algeria and Tunisia), namely: (1) the Late Jurassic to Early Aptian, associated with east-west-trending halfgrabens and related volcanism e.g. in the Pelagian block; and (2) from the post-Aptian to Early Cenomanian, associated with NW-SE trending halfgrabens [13,29].
In north-eastern Algeria, this extensional regime also resulted in the formation of graben and halfgraben systems, which controlled the distribution of carbonate platform and deeper basin facies, and therefore also of facies and organic richness of the C/T interval [13,16] (Figs. 2–4).
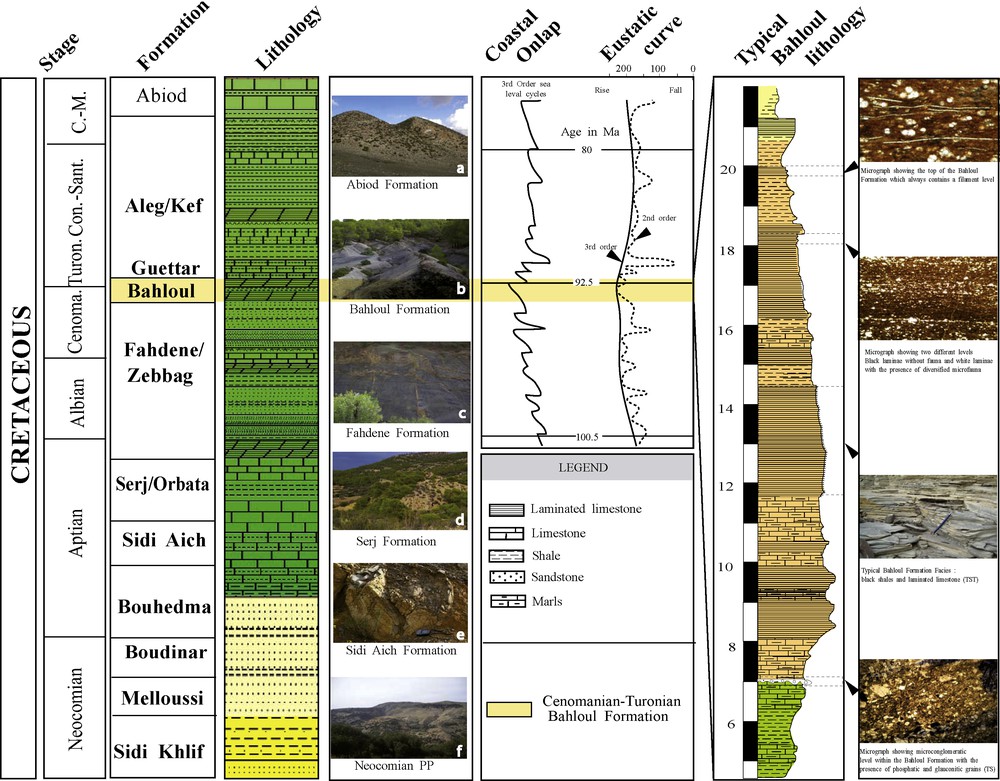
Identification of the Bahloul Formation, main lithology and characteristics superposed to the global eustatic and coastal onlap curves of Haq et al. [14] and Hardenbol et al. [15], Cretaceous lithology as defined by Burollet [3]. Note that the pictures a–d are taken from El Kef area and the pictures e and f are taken from Gafsa area.
Identification de la Formation Bahloul, principales caractéristiques et lithologie superposées à la courbe eustatique globale de Haq et al. [14] et Hardenbol et al. [15], lithologie crétacéé selon Burollet [3]. Les photos a–d sont prises dans la région d’El Kef, les photos e et f sont prises dans la région de Gafsa.
These two recorded extensional phases were interrupted by a regional top-Aptian compressional phase (the Austrian phase) which is responsible for a typical unconformity detected in seismic profiles [13,18,21,22,29] and in outcrops. The general graben trends are locally complicated by other faults which have partly dissected the grabens into smaller segments [6]. This top-Aptian compressional phase affected also the Saharan Platform (e.g., Berkine Basin) and resulted likely from transpressional movements along the north-south trending Transaharian fracture system [13,36].
Commonly, around C/T times, a general change from extension to compression occurred in North Africa, which was related to the closing of the Neotethys and the onset of North-Atlantic rifting. This resulted in the inversion of the former rift grabens from the preceding extensional phase. However, some authors assume that the C/T period was characterized by extension activities associated with a transtension and strike-slip movement [16,24,25].
In Algeria, Mid-Cretaceous extension was most intense in some localities (e.g. Constantine Basin [5,16]), influenced by two principal extensional systems, the NE-SW trending Aures-Kef trough to the north and the NW-SE trending Negrine-Gafsa trough to the south. The first direction is probably inherited from the Tethys rifting; the second corresponds to the direction of the Sirte rift system. (Figs. 1, 3 and 4).
Extensional Infracretaceous tectonics [8] was used to explain C/T halfgraben tectonics, resulting in fragmentation into small tectonic blocks [7].
Some authors assume that the rift movements were somehow blocked [12] or were restrained [6] during the anoxic C/T deposition.
In northwestern and central Tunisia as well as on the Pelagian Province, Cretaceous diapiric movements of Triassic salt played a role locally in controlling C/T deposition [6,30,36] (Figs. 3–5). They are characterized by a marked thickness reduction and partly by development of a detrital level (sandstones, conglomerates) [30,36]. The diapiric rise, starting from Aptian (and even before) to approximately Middle Eocene was probably continuous, but it increased during periods of tectonic instability [12,30,36]. Thus, earlier diapiric movements and rise-up are super-imposed to the extensional features favouring depocenters individualisations in the central parts of rim-synclines (Figs. 3–5). In the eastern foreland, i.e. east of the north-south axis, seismic interpretations by Khomsi et al. [21,22] allowed to visualise properly such features (Fig. 5).
3 Cenomanian-Turonian palaeogeography map
Combination of C/T data identified a number of major features characterizing a key period of geological history of Tunisia and eastern Algeria.
During the C/T times, Tunisia and eastern Algeria, situated at the southern Tethyan margin, were characterised by a general slope almost dipping to the north, promoting variable facies ranging from littoral (Saharan platform), shelfal and probably lagoonal (southeastern Tunisia) to bathyal (central and northern Tunisia). The general facies trend was related to a complex tectonic and halokinetic paleo-elevation resulting in variations of the C/T facies [2,28,36] (Fig. 3).
Organic-rich C/T sediments occur in many places in northern, central and offshore Tunisia and are grouped into the Bahloul Formation. The thickness distribution of the anoxic C/T facies in Tunisia and Algeria is illustrated in Fig. 3. The regional distribution of the Tunisian C/T facies including the distinction of an organic-rich and an organic-lean anoxic facies, is shown in three main Provinces (Fig. 3). Mainly it is represented in Hodna basin, Aures-Kef Trough (eastern Algeria), onshore NW Tunisia, in the Gulf of Hammamet-Gabes and locally in the Negrine-Gafsa trough (Fig. 3). Well and outcrop data confirm these organic-rich provinces, and the exact boundaries have been clearly traced through this study, especially north of the Gulf of Gabes belt. Dealing with the Soua and Tribovillard's [36] model, the palaeogeographic setting suggests that the distribution of the organic-rich facies represents the impingement of an Oxygene Minimum Zone (OMZ) onto the southern Tethyan margin. This latter facies is generally 10–30 m thick, with a maximum thickness of 80 m recorded in the NW Tunisia (Hammem Mellegue section, Figs. 3 and 4), and it consists generally of shale and limestone alternations. Limestone is dark and thinly laminated wackstone/packstone, whereas, shale is organic-rich laminated black shales with TOC values up to 14% [33,36].
In Zaghouan-Bargou area (Figs. 3 and 4), the Latest Cenomanian-Early Turonian black shales facies [34] may continue up to the Early Coniacian age. The same age-equivalent black shales are present in Venezuela under Laluna Formation name [34].
Thus, it appears that the paleogeographic distribution, regional lithofacies, and areas of subsidence were depending on the sea level fluctuation and/or tectonic movements (Figs. 3 and 4). During this interval, installation of the major Uppermost Cenomanian transgression took place inducing the filling of the paleostructures and extension of the open marine sedimentation.
According to Grasso et al. [12], an Aptian-Cenomanian rifting phase took place on the Tunisian shelf and extending towards the north-south axis [38] to the south along the Tripolitania-Jarrafa-Sirte basins [3,19] (Fig. 1). Extension and normal faults during Late Cenomanian-Early Turonian can be inferred from seismic records [21,22] (Fig. 4).
No deposition of the anoxic C/T carbonate on uplifts and paleohighs is recorded (Figs. 3 and 4). During that time, high subsidence led to the accumulation up to 80 m of laminated black shales spanning about 0.4 Ma at least in the Tunisian sections [35].
In summary, we note the reduction of emerged areas since the Cenomanian (Fig. 3):
- – to the west, the subsidence is controlled by a preferential NE-SW faulting trend (Aures basin-Kef) where maximum C/T subsidence is recorded;
- – to the southwest, the subsidence is controlled by a preferential east-west faulting trend;
- – to the east, the subsidence is mostly controlled by NW-SE faults;
- – to the central and southern domains, there is development of a wide platform with low subsidence rate (Figs. 3 and 4).
4 Cenomanian-Turonian tectonic evolution
Basin formation dynamics during the C/T time, in Tunisia and eastern Algeria is characterized by subsidence heterogeneity due to the complex structural organization. Everywhere in the studied area, structural evidence shows that this margin was under extension from Triassic until Late Cretaceous. Basins geometry reflects the inherited pattern of highs and sub-basins, which can be the result of a combination of several mechanisms including Triassic salt movement and extensional regime. Schematic cross-sectional model is used here (Fig. 4) to explain our point of view in order to understand Tunisian and eastern Algerian basins formation during C/T times. So, we propose to give an overview of major structural domain and their tectonic evolution related to tectonic subsidence.
4.1 Structural domains during the Cenomanian-Turonian transition time
During the C/T time, the southern Tethyan margin was organized as several trending Horst and Graben systems, which are from west to east:
- – NE-SW in the pre-Atlas domain;
- – east-west and NE-SW in the Aures-Kef trough;
- – NW-SE to WNW-ESE in Negrine-Gafsa trough;
- – NW-SE in eastern Tunisia.
We note that these tectonic provinces are characterized by synsedimentary normal faults, which controlled facies and thickness variation from south to north and from west to east (Fig. 3). Late Cenomanian-Early Turonian strata become thicker and organic-rich in subsident domains (Aures-Kef trough, Negrine-Gafsa trough, Kerkenah basin and Hodna basin; Fig. 3) and carbonate facies with low subsidence on resistant blocks.
At Late Cenomanian-Early Turonian, a large part of Tunisia and eastern Algeria were flooded by the major eustatic transgression that is thought to have been the most intense Phanerozoic flooding event [15,36].
During this interval, the structural framework is dominated by tilted blocks which underwent individualizing subsidence zones with variable rates (Fig. 3). They are characterized by high subsidence rates (e.g. Mellegue: 200 m/Ma; Bargou: 160m/Ma; Gafsa: 120 m/Ma [35]; Pre-Atlas and Aures-Kef trough: more than 250 m/Ma [5]; Kerkenah area: 110 m/Ma [32]).
In eastern Algeria, the thickness increases towards the Aures-Kef trough, the Hodna basin and Negrine-Gafsa trough (Fig. 3). Synsedimentary normal faults emphasise an extensional event with a regional tilting eastwards and northwards. We note that the sedimentary subbasement become deeper from southwest to northeast (Figs. 3 and 4).
Alternatively, Tunisian trough has the same structural evolution manifested by tilted blocks with an increase of the subsidence. The thickness increases towards the north and the north-east (Fig. 3). This trough organisation is closely related to the Africa-Eurasia relative movement, the opening of the North-Atlantic Ocean in the west and the Sirte basin rifting in southeast (Fig. 1).
Resistant blocks are also trending NE-SW, east-west and NW-SE and are genetically related to inherited structures characterised by low and slow subsidence amplitudes (Figs. 3 and 4).
The C/T subsidence is mainly due to extensional regime coupled with Triassic salt movements and eustasy (Figs. 3–5).
5 Conclusion
The regional C/T tectonic evolution, coupled with subsidence interpreted from palegeographic and C/T isopachous maps on several basins, wells and outcrops of central Tunisia and northeastern Algeria [5,10,13,16,20,21,22,24,36], provides valuable constraints on the C/T structural and sedimentary evolution of this part of the southern Tethyan margin (Fig. 4). Crustal extension along this margin of the African plate started during the Early Cretaceous; however, continental break-up is thought to be occurring at about 180 Ma. Subsidence during C/T time led to the accumulation of a thick sequence (up to 80 m thick in Mellegue area, NW Tunisia and about 100 m thick through the Aures-Kef trough, NE Algeria; Fig. 3) of laminated black shales and marls. Sharp variations in thickness and facies towards the north and northeast indicate that basin subsidence was driven by regional tectonic movements along NW-SE, NE-SW and east-west trending normal faults issued from Tethys and Sirte riftings. The overall evolution is also amplified by Triassic diapirism creating subsident rim-synclines allowing stratified water conditions and high preserving sedimentation rates that occurred later during Upper Cretaceous (Figs. 3–5).
Acknowledgements
The paper substantially benefited from the comments by two anonymous reviewers, Mr. Jaques Angeliers and editor Mr. Michel Petit. Authors would thank ETAP for allowing us to publish some subsurface data.