1 Introduction
The Edough massif represents the easternmost crystalline massif of the Algerian littoral. It is known for the presence of magnetite ore deposits, some of which have been exploited while and others are in the course of reassessment (Fig. 1). A number of studies have been undertaken on the formations hosting these deposits and showings. We discuss in this note the nature of these formations which correspond to the carbonated iron formations (Gross, 1980, 1990, 1995, 1996a; James, 1992). The mineral associations observed in these formations are the result of an isochemical regional metamorphism (Henni, 2005, 2006). The unusual and particular association “fayalite + calcite” was observed in Edough massif. A thermodynamic analysis based on phase diagrams constructed using thermodynamic data taken from Holland and Powell (1998) indicates the stability of the fayalite + calcite association restricted to a small domain of μO2 and μCO2 conditions during the decarbonation and the reduction of the original iron formations. This association is generally considered as impossible on the basis of qualitative arguments (Burt, 1970; Verkaeren and Bartholomé, 1979). However, its existence has been reported in several studies of eulysite-type ores (Aksamentova, 2008; Guilloux et al., 1972; Schreyer et al., 1978) and recently described in the Edough massif (Henni, 2006).
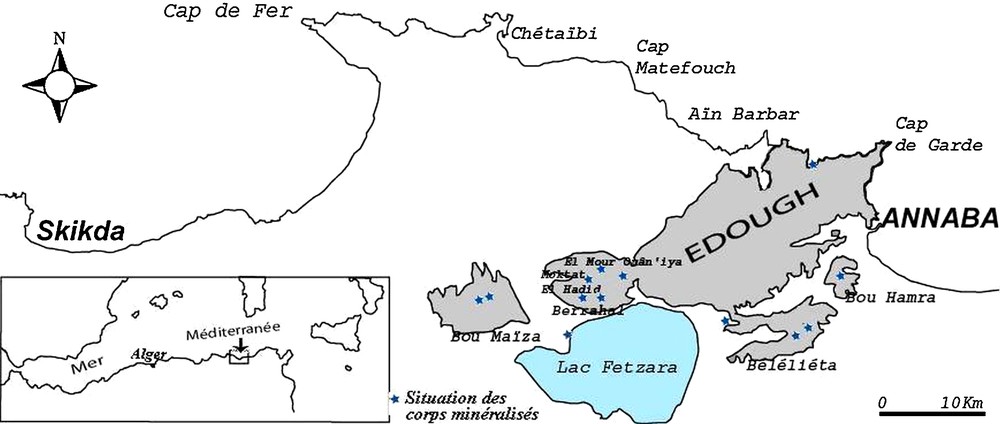
Location of the iron formations of the Edough massif. The ore deposits and showings are indicated by blue stars.
Situation des formations ferrifères du massif de l’Edough. Les gisements et minéralisations sont représentés par des étoiles bleues.
2 Regional geology
According to the study of Caby et al. (2001) on the metamorphic evolution of the Edough massif, metasedimentary rocks were formed as a result of the medium grade (6 kb, 600 °C) metamorphism of Precambrian and Paleozoic iron formations, whereas in the North of the massif, higher temperatures (700–750 °C) and pressures (12–14 kbar) are suggested from the observed mineral assemblages. According to these authors, the high-pressure metamorphic mineral assemblages formed as a result of a north-dipping subduction of the African pre-Permian crust under a flap of the suboceanic lithosphere which separated Africa from Palaeo-Europe. Both petrostructural studies (Mahdjoub et al., 1997; Saadallah and Caby, 1996) and geochronological studies (Monié et al., 1988, 1992; Peucat et al., 1996) show that the Proterozoic and Paleozoic material was remobilized by thermo-tectonic events of Cretaceous and Miocene ages. A tentative four-stage geotectonic scenario has been proposed by Caby et al. (2001) which includes:
- • sinistral transtensional setting between Kabylia and Africa;
- • oblique, dextral plate convergence and a north-dipping subduction of both the thinned crust and Africa margin;
- • a general extensional period for the entire Mediterranean region. Part of the subducted continental African crust is exhumed by tectonic denudation through low-angle extensional faults (metamorphic core complex);
- • calc-alkaline volcanism and plutonism on the northwestern side of the rising “Edough metamorphic core complex” and in the Petite Kabylie.
3 The mineralizations and their geological evolution
Geological and geochemical studies indicate that the orebodies are of metasedimentary (or volcano-sedimentary) origin and correspond to mixtures in varying proportions of a carbonate-iron ± silicate endmember of chemical origin and a pelitic endmember of detrital, volcano-sedimentary origin (Henni and Aïssa, 2007). The location of the ore bodies at several sites extending over 40 km from Bou Hamra to the east to Bou Maiza to the west (Fig. 1), and their petrographic similarities suggest that regional extent of mineralization is inherited from protoliths of sedimentary or volcano-sedimentary nature, which were subsequently transformed by regional isochemical metamorphism. The relatively high Mn content of these formations (consistent with the geodynamic context of subduction mentioned above) and their association with volcano-clastic make them comparable to the ‘Algoma’ type defined by Gross (1996b). The relative enrichment in Mn could derive from the same source as that of iron (and silica), probably related to exhalation of volcanic rocks or late volcanic-hydrothermal fluids (Adekoya, 1998; Gole, 1981; Gross, 1990, 1996a, 1996b).
Without considering the different hypotheses concerning the genesis of iron formations, the progressive evolution of the iron formations of the Edough massif can be summarized as follows (Caby et al., 2001; Henni, 2006):
- • Stage 1: deposition of a calcareous iron sequence (calcite + siderite) containing variable proportions of clay minerals with intercalated layers of ferruginous cherts. The age of this sequence is very ancient, probably Precambrian to Cambrian. This is suggested by the fact that most formations of the Edough massif are located below the least metamorphosed black metapelites of the Série Pélitique Supérieure, for which Acritarchs yielded a Lower Palaeozoic age (Ilavsky and Snopkova, 1987);
- • Stage 2: transformation by Hercynian or older metamorphism into the amphibolite facies (and beyond for the deepest units), followed by a metamorphic recrystallization accompanied by decarbonation of siderite, oxidation-reduction, and other processes which resulted in the various parageneses observed in the ore deposits of the Edough massif (see below);
- • Stage 3: additional transformations during Alpine metamorphism caused the development of a foliation as well as of a N140 lineation produced by mineral stretching in a dynamics of metamorphic core complex;
- • Stage 4: post-metamorphic precipitation of sulphides assemblages in relation (?) with Miocene magmatism in the North of the Edough massif.
Finally, the primary ore deposits were subjected to supergene alteration, which resulted in the transformation of chalcopyrite into covellite, of pyrrhotite into pyrite + marcasite, and magnetite into hematite (martite), goethite and limonite.
4 Description and characterization of the mineralized bodies
The ore-bearing bodies of the Edough massif correspond to stratiform (sometimes lenticular) layers of magnetite associated with both calcitic and calc-silicate rocks hosted in kyanite-staurolite-garnet schists in the vicinity of marbles. Three types of facies can be distinguished on the basis of petrographic and geochemical observations (Figs. 2 and 3, Table 1): The first facies denoted Mc corresponds to a carbonate ore. This ore is characterized by a carbonate gangue with alternating layers of calcite and calcite + magnetite. The relative proportions of the layers vary from one sample to the other. Siderite is sporadically present and has been observed in the eastern part of the massif (mainly at Belelieta and Bou Hamra). Quartz is also present. The second facies, denoted Ms, is a silicate ore characterized by a silicate gangue consisting of fayalite and hedenbergite. Amphibole occurs as ferroedenitic to hastingsitic hornblende, while garnet is present as almandine. The third facies, denoted Mi, is considered as an intermediate facies because of its lower silica content compared to that of Ms. The gangue consists of olivine, with some intercalated calcitic bands. Calcite and fayalite appear to coexist at equilibrium (Fig. 4).
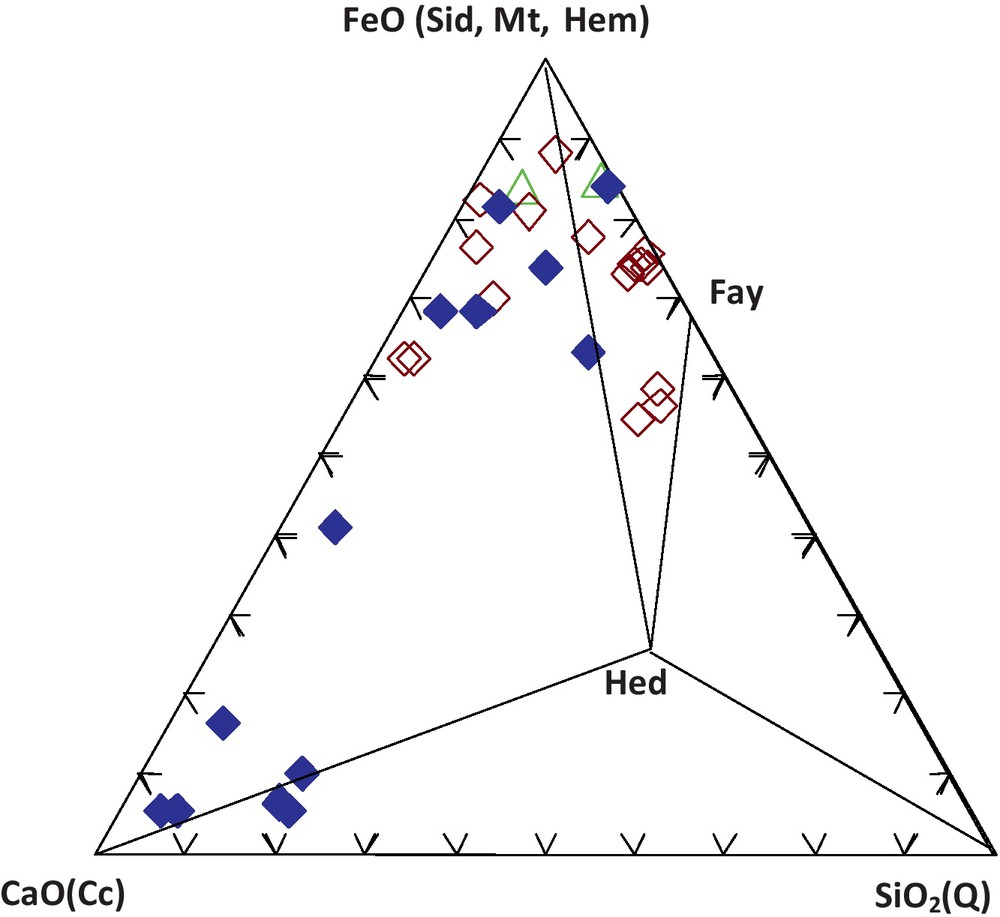
The iron formations of the Edough massif in the ternary Ca-Fe-Si diagram. Localities: Béléliéta, Berrahal, Bou Maïza. Mineral phases: Mt: magnetite; Hem: hematite; Sid: siderite; Fa: fayalite; Hed: hedenbergite; Q: quartz; Cc: calcite.
Les formations ferrifères du massif de l’Edough dans le diagramme triangulaire Ca-Fe-Si. Localités : Béléliéta, Berrahal, Bou Maïza. Phases minérales : Mt : magnétite ; Hem : hématite ; Sid : sidérite ; Fa : fayalite ; Hed : hédenbergite ; Q : quartz ; Cc : calcite.
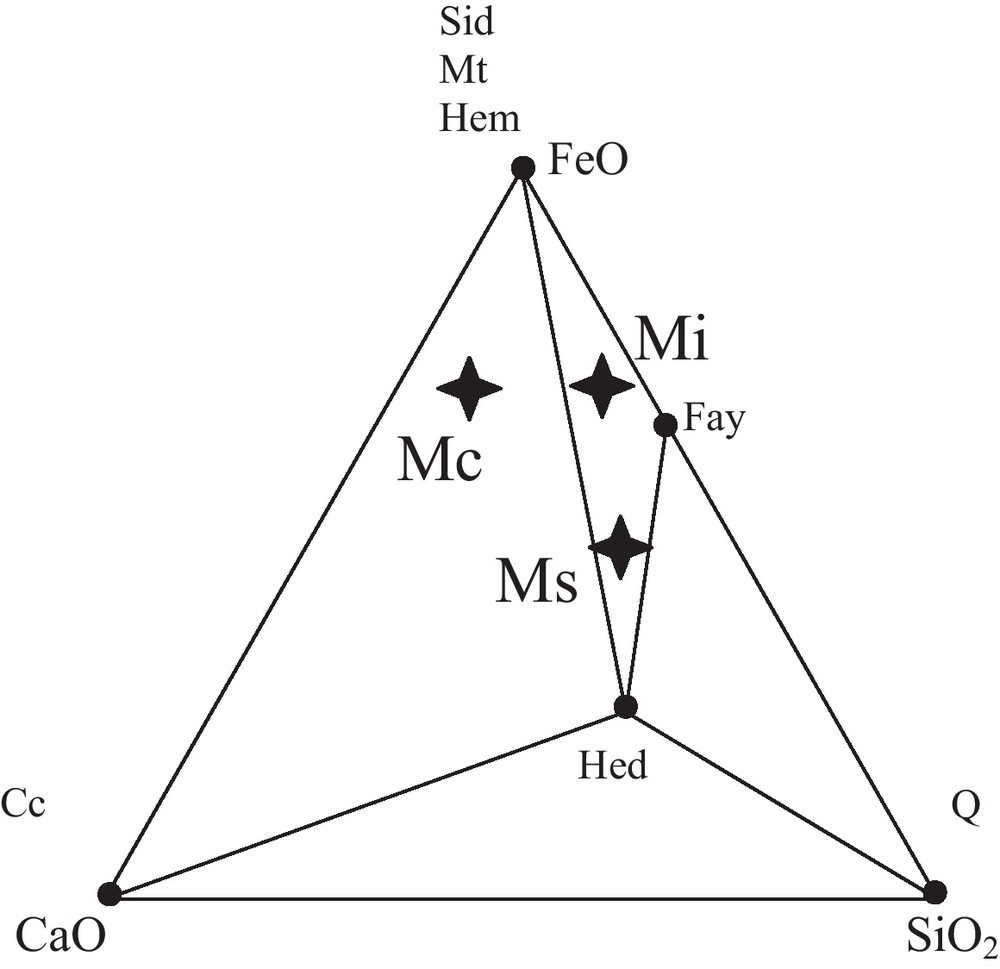
The facies of the ores in the ternary diagram CaO, SiO2, FeO. Mi: intermediate ore, Ms: silicate ore; Mc: carbonate ore.
Les faciès de minerais dans le diagramme triangulaire CaO, SiO2, FeO. Mi : minerai intermédiaire, Ms : minerai silicaté, Mc : minerai carbonaté.
Compositions (pourcentages molaires) choisies pour les principaux faciès de minerai.
FeO (%) | SiO2 (%) | CaO (%) | |
Mc (carbonate ore) | 70 | 7 | 23 |
Ms (silicate ore) | 50 | 36 | 14 |
Mi (intermediate ore) | 70 | 25 | 5 |
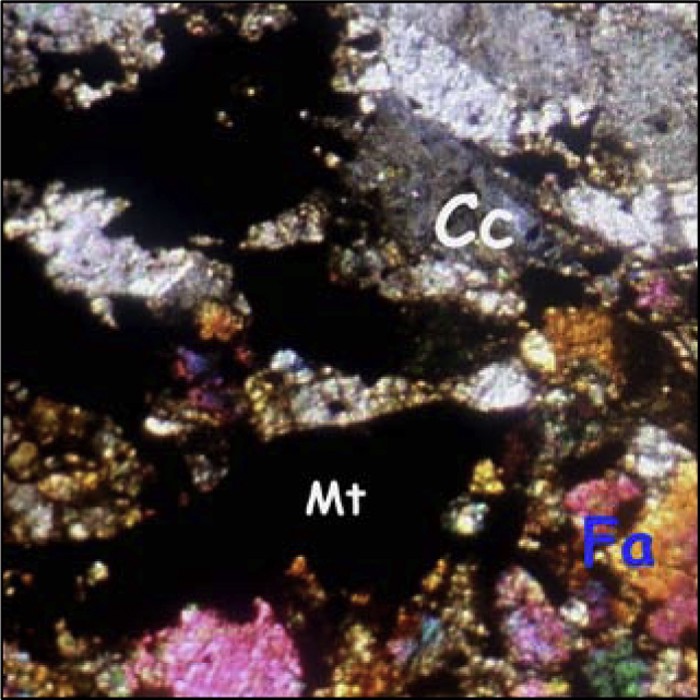
Fa + Mt + Cc association. Fa: fayalite; Mt: magnetite; Cc: calcite.
Association de Fa + Mt + Cc. Fa : fayalite ; Mt : magnétite ; Cc : calcite.
5 Thermodynamic approach
Since the chemical composition of the rocks considered in the present study may be essentially accounted for by Si, Fe, Ca, C and O (Al, Mn and Mg being much less abundant), we will restrict our thermodynamic analysis to the five-component system Fe-Si-Ca-C-O (or FeO-SiO2-CaO-CO2-O2). In this system, the mineral phases which are present in the ore-bearing bodies of the Edough massif are the following (Table 2):
- • magnetite (Fe3O4), hematite (Fe2O3), calcite (CaCO3), siderite (FeCO3), fayalite (Fe2SiO4), hedenbergite (CaFeSi2O6), quartz (SiO2); we will consider also graphite (C), wollastonite (CaSiO3) and andradite (Ca3Fe2Si3O12); the fact that these minerals are not observed in the rocks of the Edough massif can provide indirect information on the conditions of formations of ore-bearing bodies.
Matrice de composition du système étudié ; données thermodynamiques pour T = 600 °C, P = 6 kb.
Sid | Q | Cc | Fa | Hed | Mt | Wo | Hem | Andr | Gph | |
FeO (i) | 1 | 0 | 0 | 2 | 1 | 3 | 0 | 2 | 2 | 0 |
SiO2 (i) | 0 | 1 | 0 | 1 | 2 | 0 | 1 | 0 | 3 | 0 |
CaO (i) | 0 | 0 | 1 | 0 | 1 | 0 | 1 | 0 | 3 | 0 |
CO2 (m) | 1 | 0 | 1 | 0 | 0 | 0 | 0 | 0 | 0 | 1 |
O2 (m) | 0 | 0 | 0 | 0 | 0 | 0.5 | 0 | 0.5 | 0.5 | −1 |
G0 (Kj/mol) | −861.39 | −953.51 | −1301.61 | −1637.77 | −3030.2 | −1282.72 | −1718.9 | −928.76 | −6117.4 | −6.66 |
S (J/mol/K) | 204.53 | 76.29 | 226.77 | 413.64 | 411.8 | 297.46 | 197.1 | 220.65 | 787.53 | −1.16 |
V (cm3) | 2.94 | 2.27 | 3.69 | 4.64 | 6.79 | 4.45 | 3.99 | 3.03 | 13.19 | 0.53 |
We will make here the assumption that the major petrogenetic process having operated is a progressive decarbonation of the initial iron-bearing silico-carbonated rocks (siderite was present as a carbonate), with CO2 departure, 3Sid + ½ O2 = Mt + 3CO2 3FeCO3 + ½ O2 = Fe3O4 + 3CO2 as well as a limited reduction (disappearance of such oxides as hematite).
This hypothesis has been taken by several authors who studied similar types of rocks (Burt, 1970; Verkaeren and Bartholomé, 1979). In our system, we can consider that the system is open with respect to CO2 and O2, or that these elements are mobile, i.e. are free to be transferred by a general degassing process from the system to its environment. These elements will be represented by their chemical potentials; these parameters are equivalent to the logarithms of the fugacities and activities of the elements. The use of chemical potentials allows a more comprehensive and straightforward approach when all the thermodynamic data on the mixtures, in the gas or aqueous phase, are not known (the general relation between chemical potential μ, Gibbs free energy g and activity a of a phase is written as: μ = g + RTLoga). In contrast, the elements CaO, FeO and SiO2 will be regarded as inert and will be represented by their concentrations. Although the temperatures and pressure conditions obviously changed during the metamorphic process which affected the rocks of the Edough massif, we will restrict our analysis to T = 600 °C and P = 6 kb. These conditions, which are suggested by the garnet-staurolite-kyanite paragenesis observed in the schists hosting the mineralized bodies, are representative of the subduction metamorphism (stage 2, see above). Other authors made identical assumptions (Burt, 1970; Verkaeren and Bartholomé, 1979). The possible variation of P and T during the decarbonation and reduction process is actually equivalent to a change in μO2 and μCO2 conditions, as noted by Burt (op. cit.). Therefore, although P and T may have changed, we gather the influence of all the physical and chemical parameters within the sole μCO2 and μO2 parameters, and maintain P and T as constant in the following model.
The above-mentioned mineral phases of the iron formations of Edough massif are represented in the ternary diagram CaO-SiO2-FeO within which the chemical compositions of the different iron ore facies are projected (Figs. 3 and 4, Table 1). The chemical compositions of the phases of the system are given in the matrix in Table 2. In order to construct the chemical potential diagrams, the Gibbs free energies of the different phases were computed at 600 °C and 6 kb using thermodynamic data taken from Holland and Powell (1998). The uncertainties on the values of Gibbs free energies of the phases are a few joules to tens of joules, for values expressed in kJ.
6 Study of the model system
Let us consider the model system described above: SiO2, CaO and FeO components are inert and CO2 and O2 mobile. We therefore expect that parageneses within divariant fields will have three phases, those along univariant reactions will have four phases and those for invariant associations will have five phases. The following parageneses will be discussed:
- • parageneses containing calcite: they are observed mostly in the carbonated ore: Cc + Sid + Q, Cc + Mt + Q, Cc + Hed + Mt, Cc + Fay + Mt;
- • parageneses containing little or no calcite (silicate and intermediate ores) with the possible presence of hedenbergite and fayalite. In addition to the previous parageneses these are: Fay + Hed + Mt and Cc + Fay + Hed.
We use the Zen + k software (Guy and Pla, 1997b). It is based on the concept of affigraphy (Guy and Pla, 1997a) that makes a systematic use of the affinities g-μ of the reactions responsible for the appearance or disappearance of the phases of the system. The analysis of parageneses has been developed by various authors (Korzhinskii, 1959; Zen, 1966) according to the Gibbs phase rule and Schreinemakers analysis (1915–1925). Whereas Zen's approach is restricted to the systems with n + k phases for which k ≤ 3, the affigraphy approach is general to n + k systems with k arbitrary (n is the number of independent components and k the number to be added to find the total number of phases in the systems). More information about affigraphy approach and use of chemical potentials may be found in Guy (2010a, 2010b) and Albert et al. (2006) (the affigraphy approach allows to handle all metastability levels of phase associations). Implicit here is the use of phase rule for open systems, where the degrees of freedom represented by the μ’s are counted in addition to the standard P and T degrees of freedom which are not considered here (refer to the paper by Korzhinskii (1950) for phase rule in open systems, and also to Guy (2010c) for a generalization of phase rule to systems of metasomatic zones).
We construct diagrams for particular compositions of the systems (“restricted” diagrams): all possible univariant reactions are then not available to a particular composition. We study a typical composition for the carbonate ore (Fig. 5a and b), for the intermediate ore (Fig. 6) and for the silicate ore (Fig. 7). The compositions of the three ores are given in Table 1.
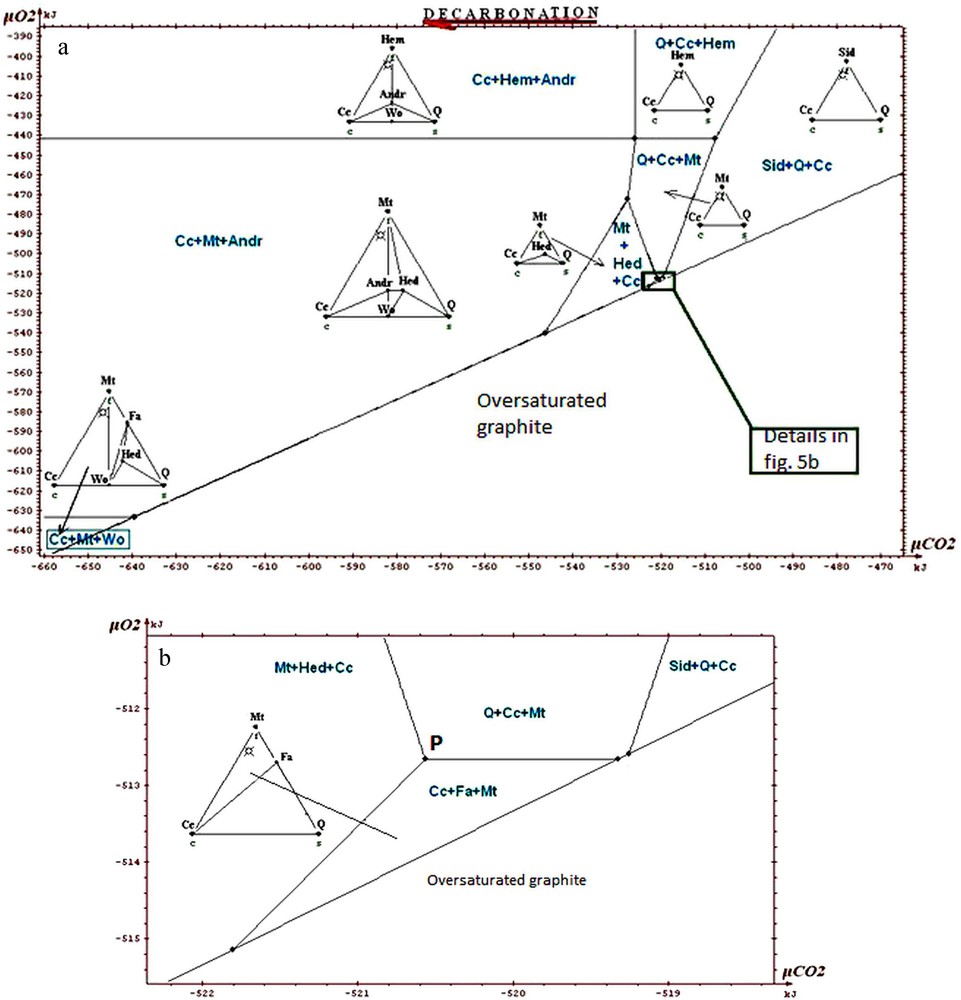
a: isotherm and isobaric diagram (600 °C, 6 kb) μCO2-μO2 of the phases in the system Ca-Fe-Si-C-O. Whole restricted diagram, carbonate ore; b: portion of restricted diagram, carbonate ore.
a : diagramme isotherme isobare (600 °C, 6 kb) μCO2-μO2 des phases dans le système Ca-Fe-Si-C-O. Diagramme restreint complet, minerai carbonaté ; b : partie du diagramme restreint, minerai carbonaté.
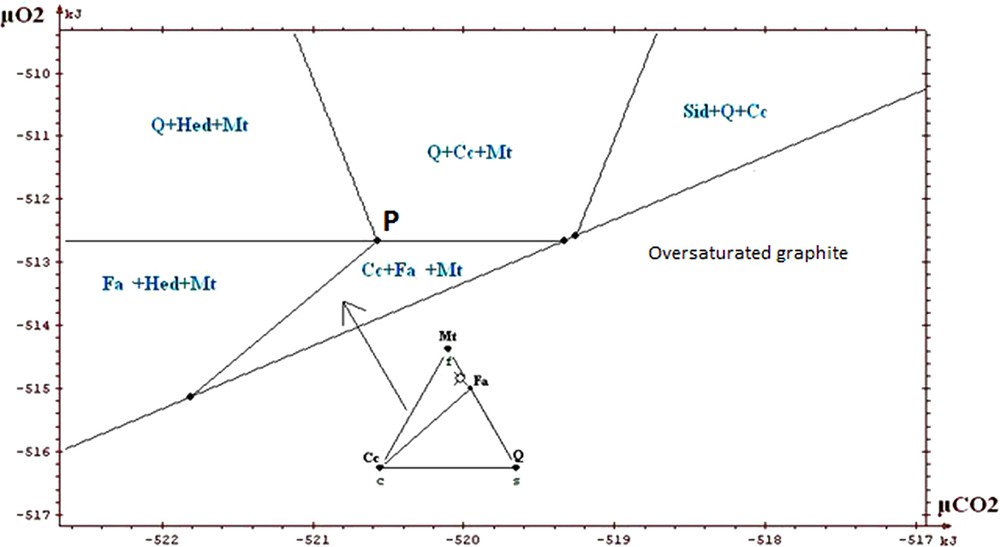
Portion of restricted diagram, intermediate ore.
Partie du diagramme restreint, minerai intermédiaire.
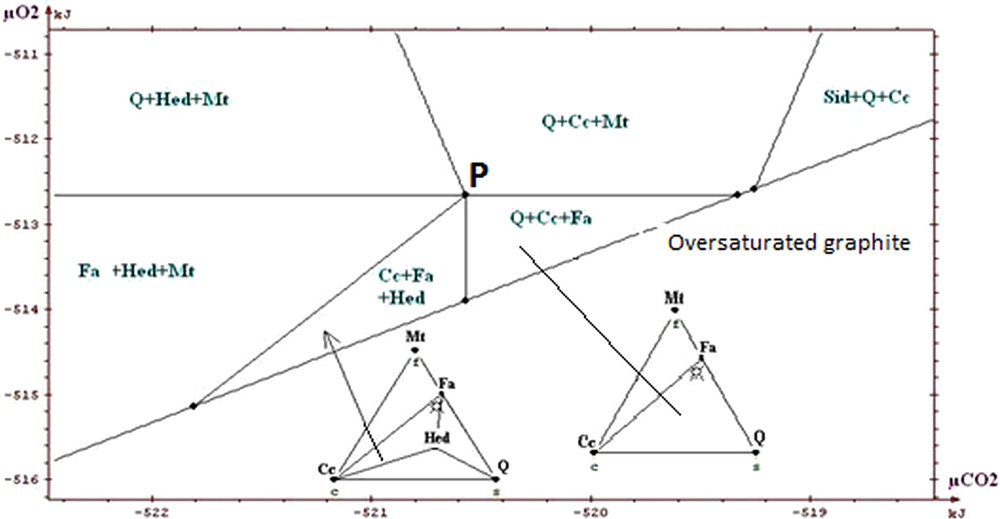
Portion of restricted diagram, silicate ore.
Partie du diagramme restreint, minerai silicaté.
The information that we can draw from the study of these diagrams (Fig. 5a to 7) is as follows:
- • general information on the situation of the rocks in the field μO2 μCO2: absence of graphite, andradite and wollastonite; scarcity of hematite. The values of parameters lie above the curve of graphite stability, but close to it, because of the existence of associations with fayalite; this gives intermediate values for μO2 and μCO2 (the conditions are exceptionally in the field of hematite for higher μO2); the conditions are neither in the field of wollastonite nor in that of andradite, which suggests that they were not too low in μCO2. The conditions stay in the vicinity of invariant point called P on the diagrams, which allows the simultaneous appearance of quartz, magnetite, hedenbergite, fayalite, calcite (invariant point with five phases);
- • on the magnification of a portion of the diagrams (Figs. 5b, 6 and 7) one sees the main differences between the various ores. Compared to the carbonate and intermediate ores, the field Cc + Fa + Mt is divided into two for the silicate ore. The influence of the starting rock is mostly revealed in the duality and two fields appear: Cc + Hed + Fa and Q + Cc + Fa. We find that the stability field of fayalite + calcite association is relatively small for the carbonate, intermediate and silicate ores. Its amplitude is of the order of a few kilojoules. We said that the uncertainty of our data was a few joules to tens of joules. By changing randomly the data, adding or subtracting a few joules, the topology of the diagram is not changed;
- • one can draw a decarbonation and reduction evolution, starting from associations with siderite, close to the hematite field, and going to the studied associations (dotted arrow on Fig. 5a). Magnetite represents reduced conditions when compared to hematite; the associations containing calcite + oxide + silicate represent a decarbonation with respect to the starting association with two carbonates (calcite and siderite).
7 Conclusion
In conclusion, we will stress the consistency of the thermodynamic study: all the observed associations correspond to the same zone of the diagrams, close to the conditions of point P, characterized by μO2 values of about −513 kJ and μCO2 values of about −520 kJ, for T = 600 °C and P = 6 kb. Several authors proposed petrogenetic grids to explain the mineral associations observed in the metamorphism of the iron-bearing formations. Burt (1970) proposed a grid based on qualitative and topological reasoning, with no use of thermodynamic data (see also the work of Verkaeren and Bartholomé (1979)). One finds on the diagrams produced by Burt the main reactions and invariant points which we found ourselves. In the sector of point P which interests us, the difference between our work and those of other authors relates to the possibility of having both calcite and fayalite, as actually observed, whereas these two minerals were considered as incompatible. In their work on the magnetite deposit of Forsyth, Guilloux et al. (1972) present a genetic model very similar to that presented here (metasedimentary origin of the deposit; decarbonation and reduction of an initial rock containing siderite, in metamorphic conditions similar to those adopted here) and observe an association of calcite and fayalite. This reinforces our conclusion on this point, although these authors did not conduct a specific thermodynamic study.
Acknowledgements
The authors thank D.E. Aïssa, J.M. Pla, M.L. Pascal, D. Garcia, J. Moutte, J. Verkaeren.