1 Introduction
The northern part of the North-South Tectonic Zone in China (NNSTZ), located along 102°–106° east longitude, includes the Alashan block, Yinshan block, Qilian block, Ordos block, Qaidam block, Songpan-Ganzi block, South China block, and Qinling orogenic belt (Fig. 1) (He et al., 2014; Li et al., 2006; Zhang and Wang, 2009; Zhang et al., 2013). This zone divides China into an eastern segment with a crustal thickness of 30–44 km and a western segment with a thickness of 54–70 km (He et al., 2014; Li et al., 2006) with a strong lateral gradient in crustal thickness and north–south-trending gravity anomaly (He et al., 2014; Li et al., 2006; Zhang et al., 2013).
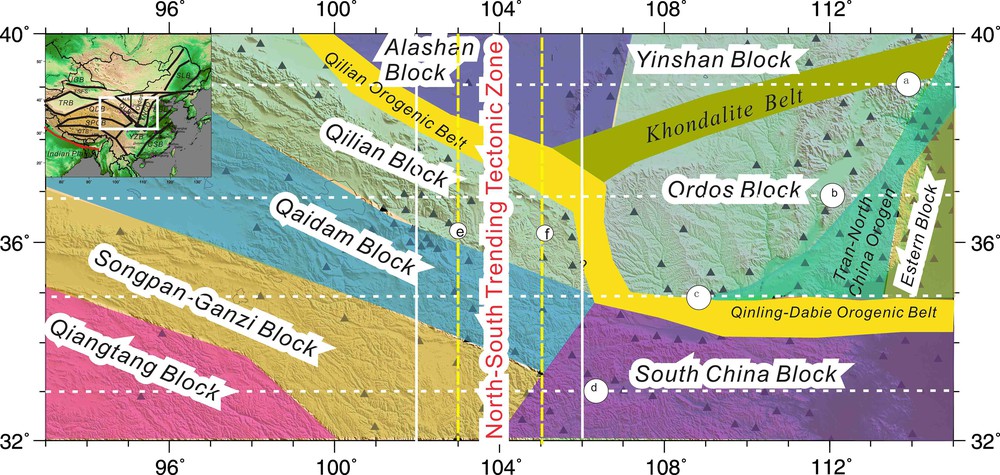
Tectonic framework in the NNSTZ and near-by area showing profiles for the velocity perturbation (a–f profiles). Black triangle: seismic station. Insert figure (upper left corner): the study area within the East Asian region (marked by box). SLB (Songliao Basin), WBL (Western Block), EBL (Eastern Block), TNCO (Tans North China Orogen), Q-DOB (Qinling–Dabie Orogen Belt), YZB (Yangtze Block or South China Block), CSB (Cathaysia Block), JGB (Junggar Basin), TSFS (Tianshan Fold System), TRB (Tarim Basin), QDB (Qaidam Block), SPGB (Songpan–Ganzi Block), QLB (Qilian Block), QTB (Qiangtang Block), ALS (Alashan Block).
The NNSTZ preserves a complex tectonic history. In its eastern side, the Ordos and Yinshan blocks amalgamated at ca. 1.95 Ga (Dan et al., 2012; Zhao et al., 2010). The collision zone between the two blocks is popularly known as the Khondalite Belt or the Inner Mongolia Suture Zone (Santosh, 2010; Santosh et al., 2007, 2013; Zhao et al., 2005). The Ordos basin (within the Ordos block) in central China is a large intracratonic compressional basin (Li, 1996; Wang et al., 2005), and its tectonic evolution was affected by both the Triassic collision between North and South China Blocks (Ames et al., 1996; Ratschbacher et al., 2000) and the subduction of the Pacific plate since the Mesozoic (Yin and Nie, 1996). In the western part of NNSTZ, the Qilian orogenic belt along the northern margin of the Tibetan Plateau of Caledonian age was formed by the convergence and collision of the Alxa, Qilian and Qaidam blocks during Late Ordovician to Devonian (Xu et al., 2006, 2010). Among these blocks, the Qilian-Qaidam blocks are considered to represent a composite fragment of the Neoproterozoic Rodinia supercontinent and shows affinity with the Yangtze craton in South China (Song et al., 2010). The Kunlun and Qilian orogenic belts represent a suture zone marking the closure of the Proto-Tethyan Ocean (Bian et al., 2004; Tseng et al., 2009; Tung et al., 2007; Xiao et al., 2015).
The critical tectonic feature and cause of frequent earthquakes along the NNSTZ (Deng et al., 2003; Zhang et al., 2003) has been the topic of a series of geophysical studies along this zone in the last decades, such as deep seismic sounding (e.g., Gao et al., 2006; Li et al., 2002), tomography, receiver function and shear-wave splitting (e.g., Ding et al., 1999; He et al., 2014; Liu et al., 1989; Wang et al., 2008), etc. These investigations revealed the crustal and lithospheric structure as well as the upper mantle structure. However, the possible link between the earthquake-prone region and deep continental dynamics has not been well elucidated.
In this study, we employed P-wave teleseismic tomography to evaluate the velocity structure of the lithosphere and upper mantle. Based on tomographic results, in conjunction with those from our previous study (He et al., 2014), we attempt to evaluate the geodynamic processes beneath the NNSTZ in order to gain insights on the relationship between the deep dynamic process and earthquake initiation.
2 Data and method
In the modeling space, a 3-D grid is set up and Vp (P-wave velocity) perturbations at the grid nodes are taken as unknown parameters. The Vp perturbation at any point in the model is calculated by linearly interpolating the Vp perturbations at the eight grid nodes surrounding that point. In our optimal Vp model, the lateral grid interval is 1°, and grid meshes are set at depths of 70, 100, 200, 300, 400, 500, 600, 700, and 800 km. An efficient 3-D ray-tracing technique (Zhao et al., 1992) is used to calculate theoretical travel times and ray paths. A conjugate-gradient inversion algorithm (Paige and Saunders, 1982) with damping and smoothing regularizations is adopted to solve the large and sparse system of observation equations (Zhao et al., 1992, 1994, 2002). The iasp91 1-D Earth model (Kennett and Engdahl, 1991) is taken as the starting model for the 3-D tomographic inversion (e.g., Lei and Zhao, 2007; Yang et al., 2014; Zhao et al., 1992).The first P-wave arrival times are picked from the origin seismograms of teleseismic events (Fig. 2). Travel-time residuals (tij) are determined by subtracting theoretical travel times and origin times from the observed arrival times. It can be expressed as:
(1) |
(2) |
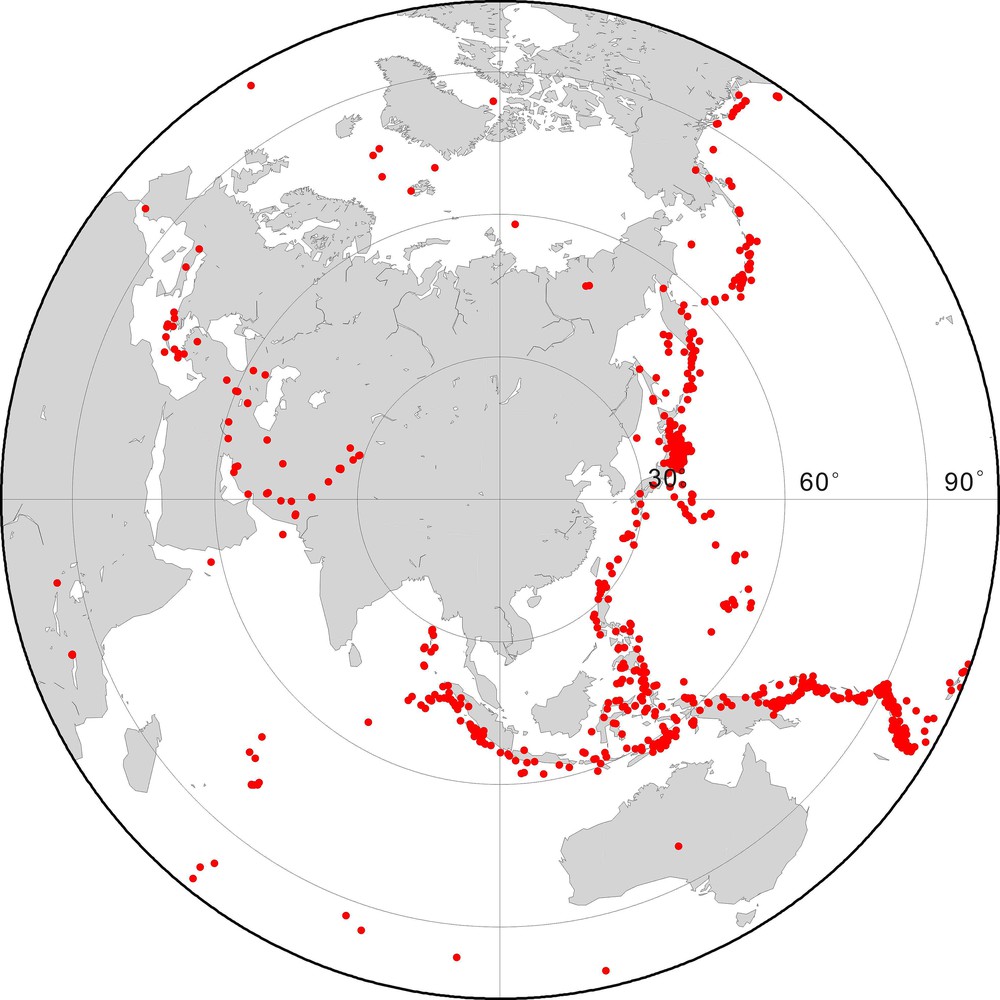
Earthquake events (red circle) that were used for tomographic inversion, recorded between July 2007 and March 2014 with epicenter distance ranging from 30° to 85° for each station-event pair.
In this study, we collected data recorded by China seismic network between July 2007 and March 2014, which comprise 203 seismic stations and the 325 events that were selected with epicentral distance ranging from 30°–85 °correspond to an earthquake magnitude > 6.0 (Fig. 2). P arrivals were correlated on the vertical component after bandpass filtering between 0.3 and 3 Hz. Our assembled data set contains 40330 P-wave arrivals from these events. The data of great than −2 s and less than 2 s were selected to use tomographic inversion (Fig. S1).
In teleseismic tomography, rays do not crisscross well in the crust and the uppermost mantle beneath the study region. Therefore, the effect of crustal heterogeneity should be removed through correcting the relative travel-time residuals, which is called crustal correction (Jiang et al., 2009, 2015). We calculated the crustal correction for the upper 70 km of the earth, which is the upper depth limit of tomography. In this work, the CRUST1.0 model (Laske et al., 2012) is used to apply the crustal correction to the relative travel-time residuals following the scheme of Jiang et al. (2009, 2015).
In order to obtain a balance between the reduction of travel time residuals and the smoothness of the 3-D velocity structure (Eberhart-Phillips, 1986; Huang et al., 2015; Lei and Zhao, 2007), we carried out a series of inversion tests with different values of damping, and eventually selected the optimal value of damping parameter as 15 (Fig. S2).
In the checkerboard resolution test, we also designed the grid spacing as 1° × 1° and the vertical grid spacing as 70, 100, 200, 300, 400, 500, 600, 700, and 800 km, respectively. Positive and negative velocity perturbations of 5% were assigned to all the 3-D grid nodes and synthetic travel times were calculated by tracing the rays for the checkerboard so that we can evaluate the adequacy of ray coverage and reliability of the main features of the tomographic images (e.g., Wang and Zhao, 2013; Zhao et al., 1992). Following this method, the synthesized data were inverted to evaluate whether the assigned checkerboard pattern could be recovered or not. The results show that the resolution is generally high in most parts of the study area such as 100, 200, 300, 400, 500 and 600 km depth sections (Fig. S3) except for the western part, whereas in the 70, 700 and 800 km depth sections, the resolution is low. We also launched the checkerboard resolution test for four east–west profiles and two north–south profiles, and the results show high resolution along most part of the east–west profile sections, except for the western part and the marginal domain (Fig. S4), and high resolution along most parts of north–south profiles, except for the marginal domain (Fig. S5).
3 Results
The tomography results show a large-scale low-velocity perturbation (Lv1) beneath the western side of the NNSTZ at 70, 100, 200, 300 km depth sections, whereas a large-scale high-velocity perturbation (Hv1) is seen at 70, 100 and 200 km depth sections in the eastern side of the NNSTZ (Fig. 3). A previous shear-wave tomographic study also identified a low-velocity structure beneath the western side of the NNSTZ, whereas the eastern side is characterized by high-velocity structure at 100 km depth (Pandey et al., 2014). Other P-wave teleseismic tomographic studies also demonstrate low-velocity perturbation along the western side and high-velocity perturbation to the eastern side of the NNSTZ at 70, 120, 140, 160, 200 and 300 km depth (Li et al., 2006; Tian et al., 2009; Wang et al., 2015). In the 300 km depth section, there is a high-velocity perturbation (Hv2) at south part of the NNSTZ and a large-scale low-velocity perturbation at the eastern side of the NNSTZ (Lv2) (Fig. 3). In the 400- and 500-km depth sections, there is discontinuous high-velocity perturbation (Hv3) (Fig. 3). In the 500- and 600-km depth sections, there is a large-scale high-velocity perturbation (Hv4) at the eastern side of the NNSTZ (Fig. 3). The velocity structure at depth sections of 700 and 800 km depth sections are mostly inferred due to lower resolution, and we exclude these from further discussion on the depth sections.

P-wave velocity perturbations at 70, 100, 200, 300, 400, 500, 600, 700 and 800 km detpth sections relative toiasp91 1D velocity model (Kennett and Engdahl, 1991). We hide portions of the model where the recovery of the starting model in the CRT was below 10% (Fig. S3).
In order to further check the above results, we performed four east–west profiles (see Fig. 1 for profile location, and Fig. 4) and two north–south profiles (see Fig. 1 for profile location, and Fig. 5). The results show a large-scale low-velocity perturbation (Lv1) in the upper mantle beneath the western side of the NNSTZ (Fig. 4a–d), whereas in the eastern side, there is a large-scale high-velocity perturbation (Hv1) (Fig. 4b–d). At the same time, the high-velocity perturbation (Hv3 and Hv4) can be clearly seen at all profile in the mantle transition zone (Fig. 4). The Hv2 is beneath the NNSTZ and at about 300–400 km in depth (Fig. 4d). The north–south profiles show a high-velocity perturbation (Hv3) in the mantle transition zone and another high-velocity perturbation (Hv2) at 300–400 km beneath the southern part of the NNSTZ (Fig. 5), and a low-velocity perturbation (Lv2) at 300–400 km in depth beneath the NNSTZ (Fig. 5f).
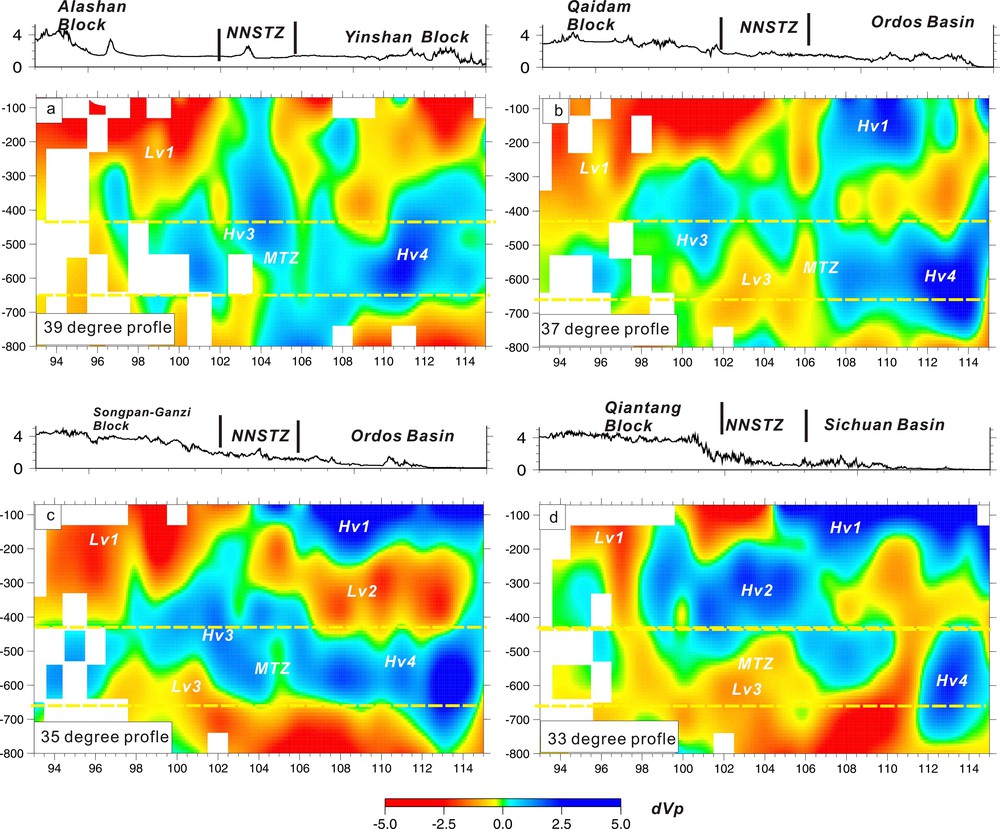
Velocity perturbation along the west–east profiles. We hide the portions of the model where the recovery of the starting model in the CRT was below 10% (Fig. S4). NNSTZ: North part of North-South Tectonic Zone. Tomographic results reveal high-velocity perturbation in the eastern side of the NNSTZ and low-velocity perturbation in the western side of the NNSTZ at the lithospheric scale. The discontinuous high-velocity perturbation (Hv3 and Hv4) in the upper mantle and mantle transition zone may be associated with the crustal and lithospheric delamination, which resulted in mantle upwelling or large-scale low-velocity perturbation (Lv2) in the upper mantle.

North–south profiles for the velocity perturbation (see Fig. 1 for profile location); a and b are 103° and 105° profiles, respectively (see Fig. 1 for profile location). We hide portions of the model where the recovery of the starting model in the CRT was below 10% (Fig. S5). The prominent high-velocity perturbation in the upper mantle and mantle transition zone (Hv2 and Hv3) and low-velocity perturbation at 300–400 km in depth (Lv2). SZ block: Songpan-Ganzi block, QT block: Qaidam block, QL block: Qilian block, AL block: Alashan block, SC block: south China block.
4 Discussion
The plate boundaries are the major regions of magmatism, metamorphism, deformation and tectonics including major earthquakes associated with oceanic plate subduction or continental collision. However, recent studies reveal that micro-continental boundaries, palaeosuture zones, mobile belts and ancient deformation zones play a critical role intra-plate tectonics (e.g., Aitken et al., 2013; Gorczyk and Vogt, 2015). Especially, the compressed lithosphere can develop a wide range of lateral heterogeneities in such settings, leading to magmatism, deformation, metallogeny, topographic evolution, tectonics, and earthquakes (e.g., He et al., 2014).
The continental lower crust is considered as the bridge between the mostly felsic upper crust and the ultramafic upper mantle (Christensen and Mooney, 1995; Rudnick and Fountain, 1995; Wang et al., 2012). The high density or the ultramafic/mafic composition of the lower crust might lead to instability, resulting in the lower crustal and (or) lithospheric delamination induced by the compressed crust and (or) lithosphere (He et al., 2013, 2014, 2015; Jull and Kelemen, 2001; Kay and Kay, 1993; Kay and Mahlburg-Kay, 1991; Rudnick, 1995; Rudnick and Fountain, 1995; Seber et al., 1996; Willbold and Stracke, 2010). The process of delamination is also important in the cycle of mantle convection, and the heterogeneous lower crustal composition contributes to the variation in mantle velocity structure (Hart, 1988; Hofmann, 1997; Wang et al., 2013).
Geological investigations have suggested large-scale delamination of the crust and lithosphere following the convergence between the Yangtze, North China Cratons and the North Tibetan continental blocks in the Triassic (Zhang et al., 2008), with the delaminated lower crust and lithosphere gradually sinking into the mantle transition zone. The large-scale high-velocity perturbation at the mantle transition zone (Hv3) (Fig. 3, 4a–c, and 5) beneath the NNSTZ might be linked with the crustal and lithospheric delamination in the Triassic, which resulted in a cold domain there, as suggested by the shallowing of both the 410- and 660-km discontinuities (Fig. 6) (He et al., 2014). The crustal and lithospheric components undergo melting in this region, which trigger plume-like upwelling due to buoyancy reflected as low-velocity structure (Lustrino, 2005) (Lv2) (Fig. 3, Fig. 4c, Fig. 5f). On the other hand, the westward subduction or compression of the Pacific plate in the NNSTZ generated an obvious gradient in crustal thickness (Fig. 7a) since the Mesozoic (He et al., 2014), leading to continuing crustal and (or) lithospheric delamination in this area. The high-velocity perturbation at 300–400 km in depth (Hv2) (Fig. 3, Fig. 4d and Fig. 5) is shallower than that of Hv3 (Fig. 3, Fig. 4a–c and Fig. 5), which might indicate new delamination beneath this area. The delamination also induces asthenosphere upwelling into the void (He et al., 2014; Kay and Kay, 1993). Our results suggest that the delamination, both past and present, in the area generates vertical convective circulation of the upper mantle (He et al., 2014). The delamination of the lower crust and lithosphere also resulted in felsic composition of the lower crust (or low Vp/Vs ratios) in the NNSTZ (Fig. 7b) (He et al., 2014; Kay and Kay, 1993).
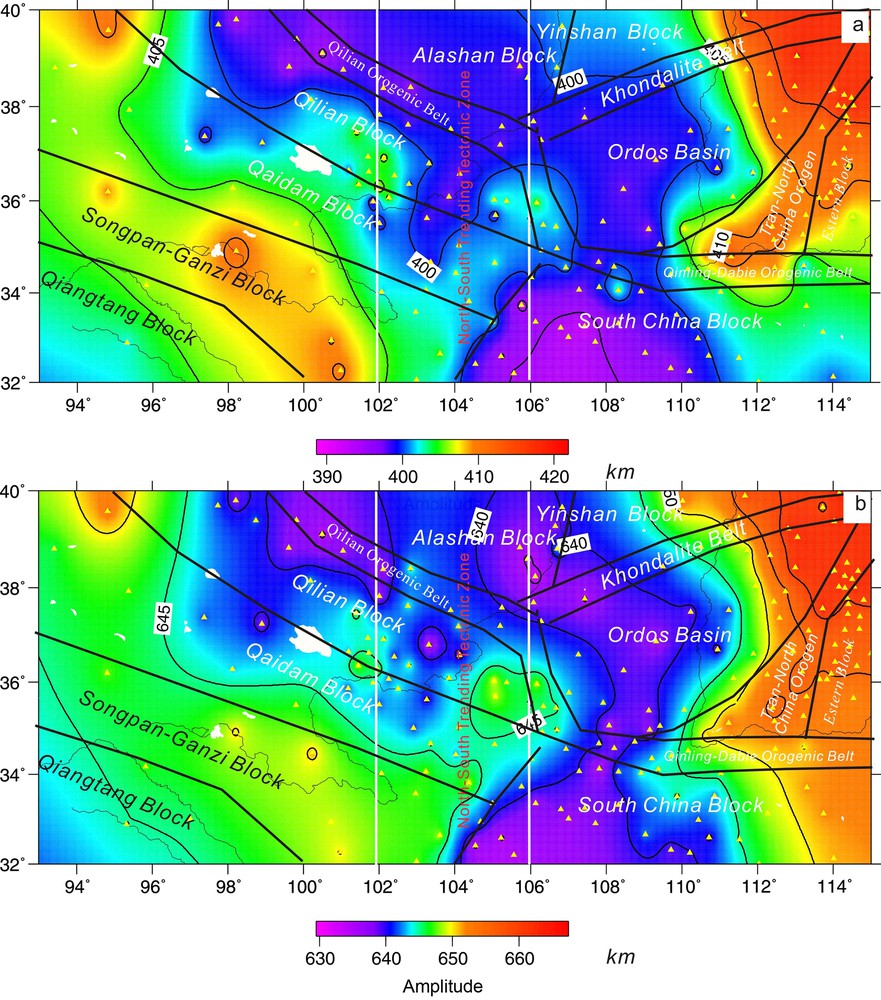
Topography of the 410 and 660 km discontinuities. A prominent shallowing of both 410 (a) and 660 km (b) topography along the NNSTZ can be seen, possibly correlated with crustal and (or) lithospheric delamination into the mantle transition zone and the resulting cold domain (He et al., 2014).
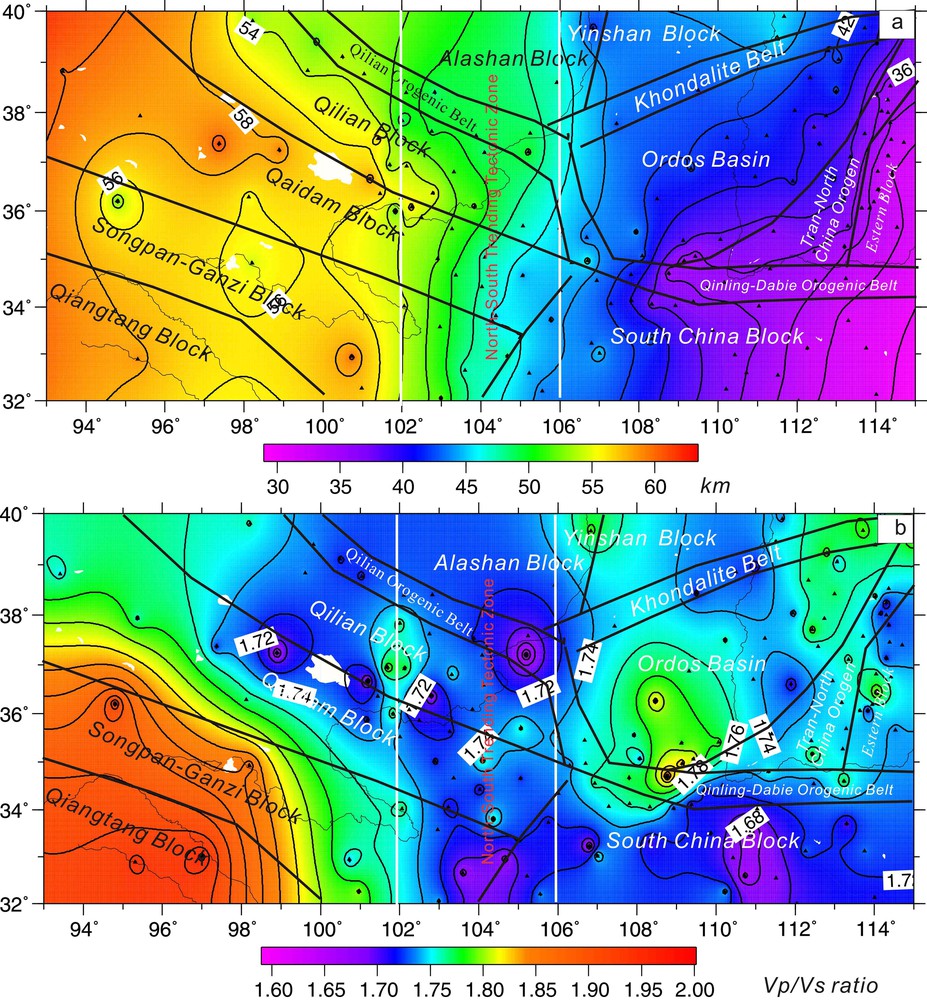
Distribution of crustal thickness (a) and Vp/Vs ratio (b). A west–east-trending prominent gradient of crustal thickness along the NNSTZ (a) and a prominent low Vp/Vs ratio along the NNSTZ (b) imply that the NNSTZ underwent later crustal delamination (He et al., 2014).
Previous seismic studies reveal that the lithosphere–asthenosphere boundary lies at a depth of 125–135 km beneath the northeastern Songpan–Ganzi terrane and the western Qinling orogen, between 145 and 175 km beneath the East Kunlun and Qilian orogens, between 175 and 190 km beneath the Qaidam Basin, about 170 km beneath the Ordos Basin and about 200 km beneath the Alxa platform (Zhang et al., 2012; Zheng et al., 2013), respectively. These results suggest the existence of a lithospheric root in the western side of the NNSTZ. However, our results show a large-scale low-velocity perturbation (Lv1) in this region (Fig. 3, Fig. 4a–d), which might suggest that the lithospheric root is weak or absent and also mark the eastward flow from Tibetan plateau. At the eastern side of the NNSTZ, the high-velocity perturbation (Hv1) (Fig. 3, Fig. 4b–d) correlates with the lithospheric root of the Ordos basin and/or that of the Yangtze block (Sichuan basin), which might block the eastward flow from the Tibet Plateau and resulted in stress accumulation at the NNSTZ. At same time, the marked difference in the velocity structure of the lithosphere between the two sides of the NNSTZ implies that there might be not only stress accumulation but also material exchange between the two sides of the NNSTZ, leading to the generation of earthquakes in the NNSTZ.
5 Conclusions
The tomographic results presented in this study confirm the deductions from a previous receiver function study that the delamination of the crust and (or) lithosphere resulted in the mantle upwelling and vertical convective circulation beneath the NNSTZ.
Our results also reveal low-velocity perturbation in the western side of the NNSTZ and high-velocity perturbation in the eastern side on a lithospheric scale, suggesting material exchange and stress accumulation leading to earthquakes beneath this region.
Based on the above results, we suggest that the vertical convective circulation and the velocity perturbation difference in the lithospheric scale between the two flanks of the NNSTZ resulted in stress accumulation and release along this zone, marking the region as earthquake-prone.
The NNSTZ appears to represent a typical case where deformation and the associated tectonic processes trigger large earthquakes, which are associated with mantle dynamics within intra-continental setting related to far-field tectonics.
Acknowledgements
We thank associate editor M. Campillo, and the two referees Prof. Wenjiao Xiao and Prof. Yanghua Wang for their constructive comments. We thank Prof. D. Zhao, who offered the source codes for tomography. We are also thankful to the guidance and constructive comments of Prof. Shunping Pei. The waveform data for this study were provided by Data Management Centre of China National Seismic Network at Institute of Geophysics (SEISDMC, http://dx.doi.org/10.11998/SeisDmc/SN), China Earthquake Networks Center and GS, GX, NX, QH, SC, XZ Seismic Networks, China Earthquake Administration (Zheng et al., 2010). This study contributes to the Foreign Expert funding from China University of Geosciences, Beijing, and Professorial support from the University of Adelaide to M. Santosh.