1 Introduction
Nummilitic limestones have been extensively used as building rocks in the Mediterranean basin since the first constructions made by humans. This is the case of important archaeological sites like the ancient Corinth (Greece), several monuments in Cairo (Egypt) such as temples and pyramids (Fitzner et al., 2002), and the cathedral of Girona in Spain (Esbert et al., 1989), among others. The use of these limestones was, and still it is, facilitated by (i) the abundant exposures located in the circum-Mediterranean countries, and (ii) the common easy extraction and shaping process of limestones compared to harder rock types.
Nummulitic limestones, Eocene (Ypresian–Lutetian) in age, belonging to the El Garia Fm (Metlaoui Group) (Fournié, 1978; Moody and Grant, 1989; Perthuisot, 1974, 1979; Tlig et al., 2010) (Fig. 1 and Fig. S1), are the most common building rock used in the Roman City of Dougga (Thugga). Dougga is located in the north-central part of Tunisia, in the Béja Governorate, at around 100 km southwest of Tunis. Dougga has a warm-temperature Mediterranean climate, with a hot and dry summer, which is classified as Csa according to the Köppen–Geiger climate system (Kottek et al., 2006). The average annual temperature is 18 °C, with a minimum average value of 11.4 °C and a maximum average value of 24.7 °C, the annual precipitation is 504 mm, and the mean month insulation is 211 h (National Institute of Meteorology of Tunisia).
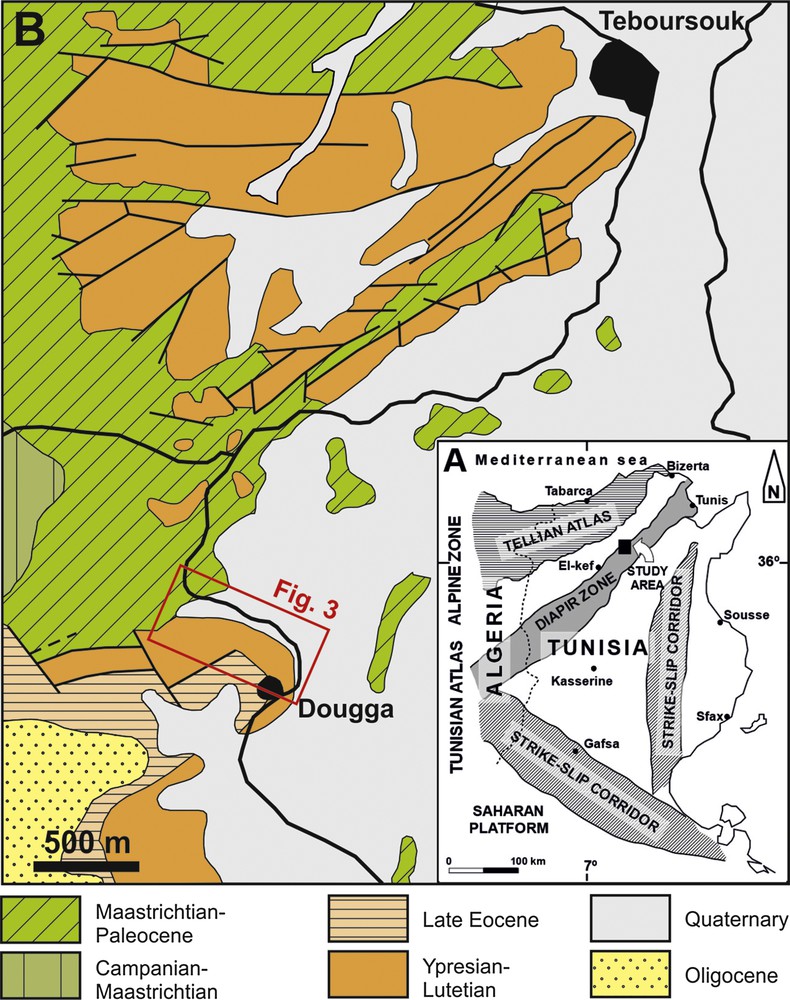
A. Location of Dougga in Diapir Zone of the north-central part of Tunisia. B. Simplified geological map of the Teboursouk region, showing the age of the rocks cropping out in the area of Dougga (based on Perthuisot, 1979).
This archaeological site covers an area of approximately 75 ha, although only one third of them have been excavated. The building rock was extracted from local quarries that are still located and perfectly recognized in the vicinity of the city (Fig. S2). The ruins of Dougga represent a complete city with all its components, testimony of more than 15 centuries of history (Khanoussi, 1989, 2003). They are an extraordinary illustration representing the synthesis between several cultures, including Numidian, Roman, Punic and Byzantine ones.
The city of Dougga possesses a remarkable group of public buildings dating back, for the most part, to the 2nd and 3rd centuries A.D. (Fig. 2). It conserves traces of the different periods of the Antique city with all its components: the monumental centre (capitol, forum, market, Rose of the winds square, etc.), entertainment buildings (theatre, circus), and public baths. Dougga is considered the best-preserved example of an Africo-Roman city in North Africa (Ennabli, 2000), and so was inscribed on the World Heritage List of UNESCO in 1997.
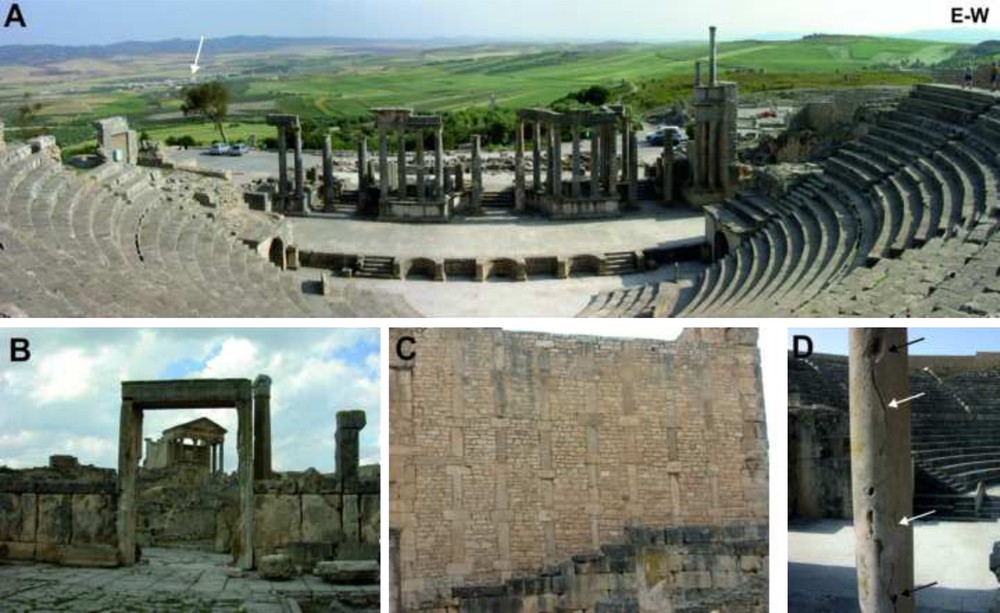
Field views of some of Dougga's buildings made with nummulitic limestones. A. Panoramic view of the amphitheatre with the valley and Nouvelle Dougga (arrow) in the background (S. II AD). B. View of the market's entrance with the Capitoline Temple (s. I AD) in the background. C. Wall made of centimetre- to decimetre-sized rock blocks. Note the meter-scale blocks used in the base of the wall. D. Detail of a column from the amphitheatre showing fractures (white arrows) and macrovugs (black arrows).
Despite the overall good state of preservation of Dougga compared to other Roman cities of Tunisia such as Carthage, some buildings have been partially destroyed, and numerous original rock elements are broken or disappeared (Fig. 2). Based on their work about the geomorphological hazard in Dougga, Lazzari and Lazzari (2012) recommended the reinforcement of the monumental structures to inhibit the movements of the soils and save the city from its progressive deterioration and from the risks caused by natural disasters. Moreover, the building rocks show some located weathering patterns such as fissures, desquamations, pitting, and fragmentation, typically affecting columns. Surface erosion and biological colonization are also frequent. In this regard, future conservation and restoration works will need information regarding the origin and characteristics of the building rock. Nevertheless, no works regarding the petrophysical properties and durability of this building stone have been carried out up to now and most, restoration works are realized without preliminary studies. Petrographic, physical, hydric, mechanical studies and ageing tests are the standard petrophysical analyses frequently used for this purpose (Borrelli and Urland, 1999; De Kock et al., 2015; Esbert and Marcos, 1983; Esbert et al., 2008; Gauri and Punuru, 1989; Lazzarini and Laurenzi Tabasso, 1986; López-Arce et al., 2008; Ordoñez et al., 1997; Robertson, 1982; Torraca, 1999; Villegas Sánchez and Vale Parapar, 1993; Winkler, 1975). Such analyses allow predicting possible interactions with other building materials such as mortars, to assess the effectiveness of conservation treatments and rock durability to different agents of decay that may affect the monument in the future.
The aims of this work are (i) to characterize the local building rock used in the construction of Dougga, and (ii) to assess its durability concerning several factors of weathering. To achieve these goals, the petrophysical properties of these materials are determined allowing the understanding of decay mechanisms of the building rock and so facilitate and improve its use in future restoration works.
2 Results
2.1 Petrography of the building rock
Macroscopic observations allow determining four main rock lithotypes of the Eocene nummulitic limestones used as building materials in the archaeological site of Dougga, labelled here as 2F, 2G, L1, and L3 (Fig. S3). Lithotype L1 is characterised by the presence of terrigenous grains that appear partially dissolved in the surface of the rock, likely increasing porosity compared to other lithotypes. Lithotype L3 is characterised by very coarse-sized nummulites that appear aligned with each other, providing to this rock a very distinctive aesthetics appearance compared to other lithotypes. Lithotypes 2F and 2G have very similar characteristics but differ in the grain size of the skeletal components, mostly nummulites, being 2F characterised by fine-sized specimens and 2G by coarser-sized specimens. Lithotypes L1, 2F and 2G have been preferentially used in the construction of the walls, while lithotype L3 was mainly used for columns and floors. The petrographic characteristics of each lithotype are reported in the following sections.
Lithotype L1
Nummulites are the main component of this lithotype, mostly between 2 and 3 mm in size although can reach up to 6 mm. The sorting is moderate to good, and no anisotropies were identified. Nummulites represent about 70% of the skeletal components together with minor discocyclinids, red algae, and echinoids fragments (spines and plates) (Fig. S3, A). Quartz and monocrystalline single calcite grains appear as accessory minerals. The intergranular pore space is totally cemented by a sparry to microsparry calcite cement, whereas syntaxial calcite overgrowths precipitated in optical continuity with echinoids fragments. Therefore, the rock is classified as a grainstone of nummulites according to Dunham (1962). This lithotype is characterised by intraparticule porosity that resulted from the dissolution of sedimentary rock fragments, mainly micritic limestone grains with sizes between 2 and 5 mm (Fig. S3, A). Partial cementation by sparry to microsparry calcite crystals nearly destroyed completely this kind of porosity. Nevertheless, the average pore size diameter ranges between 100 to 200 μm in the interparticle space.
Lithotype L3
This lithotype is also a grainstone whose main components are bioclasts, essentially displayed by large oriented nummulites (80–85%) reaching 30 mm in size, although the mode is between 4 and 5 mm (Fig. S3, B). Echinoids fragments are also observed. The inter- and intraparticle pore space is filled with sparry to microsparry calcite cement. Syntaxial overgrowths cement around echinoids fragments are also observed. The porosity is low although inter- and intraparticle porosity can be distinguished. Intraparticule porosity corresponds to the nummulite chambers, which are partially filled with sparry to microsparry calcite cement and range in size between 50 and 200 μm (Fig. 3B).
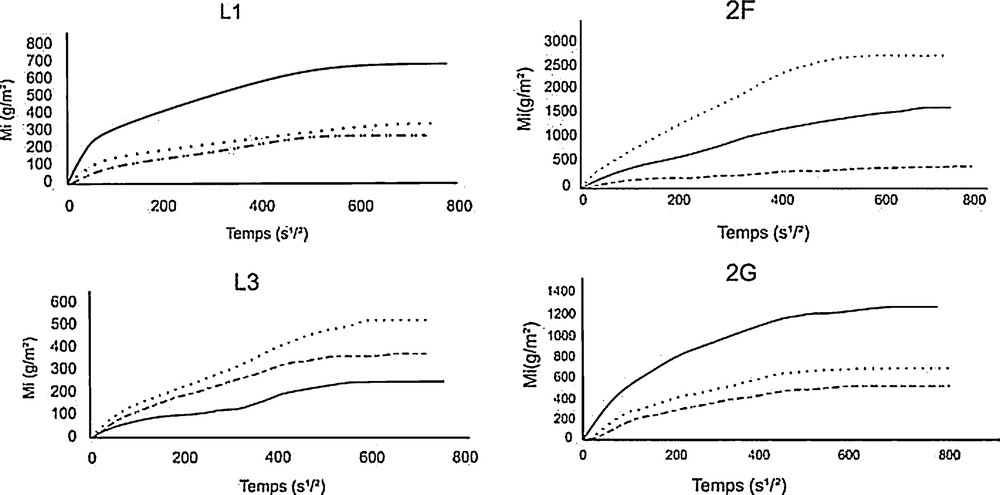
Water absorption curves of the four lithotypes, showing the variability in kinetic capillary absorption between the four lithotypes.
Lithotype 2F and 2G
Both lithotypes show grainstone fabrics, being the components dominated by echinoids, red algae and gastropods fragments, with grains between 0.1 and 0.4 mm in size. Nummulites and discocyclinids are also observed although at a much lower percentage compared to other lithotypes (< 10%) (Fig. S3, C). Cements are sparry calcite with syntaxial overgrowth growing around echinoids fragments. The porosity is essentially interparticle with average pore diameter between 100 and 300 μm. Lithotype 2G has the same petrographic characteristics than lithotype 2F but shows an increase in the content and size (2–4 mm) of discocyclinids and nummulites foraminifera (Fig. S3, D).
2.2 Petrography of the quarry rock
Two quarries are recognized in the surroundings of Dougga (Fig. S3). The most important one is located in the north of the city, next to the Temple of Saturn, and has an extraction front 700 m long and 50 m high (Fig. S4, A–B). This front represents the first third part of the logged stratigraphic succession and is labelled as Lower quarry (Figs. S2 and S5). Medium to large (decimetre to metre thick) stone blocks (ashlars) were extracted from the Lower quarry, where the thickness of the bedding allows such dimensions. This is noted by the shaped ashlars still preserved in the base of the front (Fig. S4, C) and the exploitation marks observed at the top of the extraction front (Fig. S4, D–F). The rock extracted from this quarry correspond to lithotypes L1, L3, 2F, and 2G as recognised in the monument (Table SM1). Specifically, lithotype L3, which is characterised by the occurrence of large size of nummulites aligned in the same direction and typically used in the construction of columns, was extracted from the uppermost beds (Figs. S4, E and S5).
A second quarry is located 750 m northwest of the city and has an extraction front 500 m in length and 25 m in height. This front represents the top of the logged stratigraphic succession and is labelled as Upper quarry (Fig. S2 and S5 and S7). These strata are generally thinner and thus provided small to medium (centimetre to decimetre thick) stone blocks. The rock types extracted from this quarry have equivalent petrographic characteristics, and thus correspond to lithotypes 2F and 2G as recognised in the monument (Table SM1).
2.3 Pore network characterisation
The total open porosity of the rock, determined by mercury intrusion porosimetry, shows very low values ranging from 2.02 to 4.04% (Table SM2). All lithotypes present a microporous structure with pore diameter < 5 μm (Fort et al., 2013; Gomez-Heras and Fort, 2007; Russell, 1927).
Lithotypes L1 and L3 have similar porosities, 2.52 and 2.03% respectively, but a different pore size distribution (Fig. S6). L1 has a bimodal distribution with the pore modes ranging between 0.005 and 0.01 μm and between 0.015 and 0.025 μm. By contrast, the L3 distribution is unimodal and ranges between 0.05 and 0.2 μm. Lithotypes 2F and 2G show different pore size distribution despite petrographic similarities. In the case of lithotype 2G, the mode is between 0.02 and 0.05 μm, while 2F displays a mode with larger pore diameter, between 0.05 and 0.25 μm (Fig. S6, Table SM2).
Results of MIP and OFM suggest that the pore network of these limestones, with pore diameter lower than 1 μm, in all cases is of fissure type (Fig. S7). Occasionally, these fissures can be connected with larger pores (vugs). However, the mercury porosimetry only measures the size of pore access, and thus the majority of the pores larger than the fissures are not represented in the distribution curves, but clearly identified in the thin sections by OFM. Macroporosity directly accessible to mercury has a very low percentage, which is in accordance with the MIP curves.
2.4 Hydric behaviour
Porosity values obtained by absorption of water under vacuum are very low, ranging from 2.03 to 4.10%. Lithotype L3 presents the lower porosity value (2.03%), while 2F presents the higher one (4.10%) (Table SM2). L1 and 2G present coefficients of 2.6 et 3.9%, respectively. In all the studied lithotypes, the porosity, calculated with the hydrostatic method, is very similar to that calculated by mercury intrusion porosimetry. The small difference between the values obtained with both analytical techniques is likely related to the occurrence of large pores that the mercury porosimetry technique cannot detect.
This is indicative of the good connectivity of the porous network of these rocks. The saturation coefficient (Ws) is very low in all lithotypes. Lithotype L3 shows the lowest saturation value with 0.77%, followed by lithotype L1, with 1.01%, and 2G with 1,50%. Lithotype 2F presents the highest value (1.62%). The saturation results are in concordance with porosity data. The capillary absorption coefficient is low and varies between 0.79 (L3) and 3.62 (g/m2·s0.5) (2F). Lithotypes L1 and 2G display coefficients of 2.8 and 2.86 (g/(m2·s0.5), respectively (Table SM2). The results depend on the porosity percentage of each lithotype and on its pore size distribution. We can note that the higher the porosity percentage, the higher the capillary coefficient. At very close porosity values (L3–L1), the higher the pore diameter, the lower the capillarity coefficient. The low capillarity coefficient of lithotype L3 can be explained also by a higher tortuosity of its pore network due to the higher percentage of intraparticle porosity corresponding to the nummulite's chambers (Table SM2) (Fig. 3).
Regarding the drying curves of lithotypes L1, L3, and 2F (Fig. 4), the high values of the critical water content (Wc) imply a low rate of evaporation, because almost 50% of the absorbed water under vacuum is evaporated from inside the rock in vapour form. For lithotype 2G, the drying rate is slightly higher, because Wc represents almost one third of the maximum absorbed water by the specimens (Table SM2). Therefore, only a third (almost 33%) of the absorbed water under vacuum is evaporated from inside the rock by diffusion, so the drying process is slightly faster.
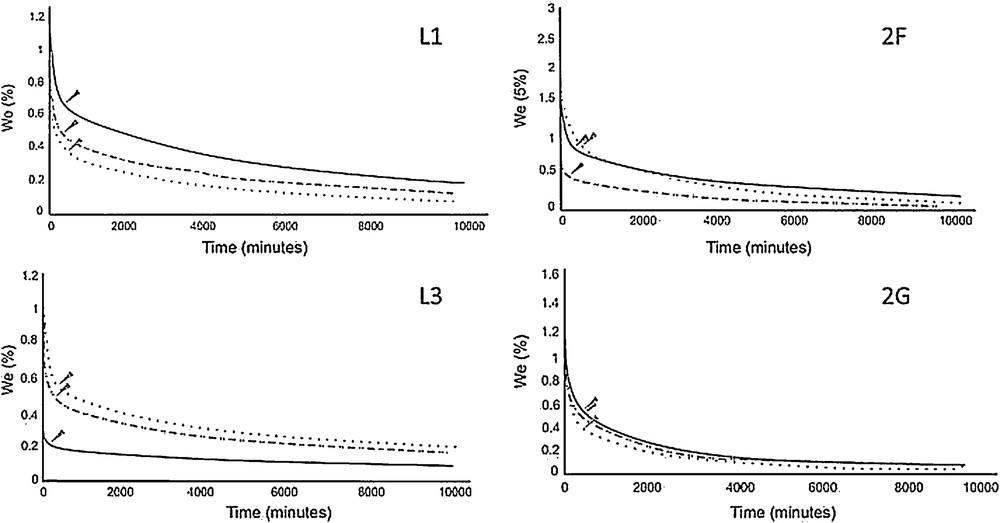
Water drying curves of the four lithotypes, showing the variability in the kinetics of the drying process. We represent the percentage of water remaining in the stone at each time interval. The arrows indicate the position of the critical water content (Wc).
All the studied lithotypes retain a slight amount of water, despite the microporous structure of the rocks. This effect supports the fissured rock pore network type. Particularly, lithotype L3 retains more water at the end of the drying test, which is interpreted to result from the presence of a higher percentage of intragranular porosity inside the large nummulite chambers (partially cemented chambers) compared to the other lithotypes. On the contrary, lithotype 2G retains a lesser amount of water compared to the other samples, which is due probably to the continuous pore size distribution, which gives rise to faster evaporation rates.
2.5 Mechanical behaviour
The values obtained by the compression test range from 60.11 to 91.4 MPa. Lithotype L1 presents the highest value (91.4 MPa), followed by lithotype L3 (71.63 MPa). Lithotypes 2G and 2F have similar values, 60.11 and 69.40, respectively. The obtained values indicate that the studied rocks have a very high mechanical strength. At similar mineralogy and fabric, it seems that the compression strength is related with the porosity volume. In fact, lithotypes with higher porosity (2F and 2G) show a higher compression strength. Values obtained by the abrasion test are low and very similar; this is can be due to the very close mineralogical composition of all lithotypes (Table SM3).
2.6 Durability
The visual inspection of the samples’ appearance before and after the ice crystallisation test shows that the tested rocks have not suffered any type of weathering (colour change, fissures, and exfoliation). However, the comparative values of the initial and final apparent volume show a slight decrease (Table SM4). This is indicative of a low-rate internal decay. Lithotype L3 is the sample affected at the least extent.
The results of the ageing test with SO2 show that all lithotypes have a high resistance against SO2 action. A slight weight loss has been registered (Table SM5) in samples exposed to both solutions (0.31 to 0.44% for solution A and 0.13 to 0.22 for solution B). However, no significant apparent weathering patterns such as oxidations, spots, swelling, and peels have been observed.
Based on the results from Table SM5, the higher the concentration of SO2 in the solution, the higher the damage of the samples. The difference found in the weight loss after 22 cycles among the lithotypes is not really significant. Some crystals of a soft white compound have showed up on the sample surface treated with both solutions, although in solution A there are more abundant than in the solution B, as it was expected due to the increased aggression with higher SO2 concentration. These white spots correspond to the formation of calcium sulphate by the following reaction (Amoroso and Fassina, 1983; Charola and Ware, 2002; Fassina et al., 1994; La Bastille, 1981; López-Arce et al., 2016; Mohnen, 1988): CaCO3 + H2SO4 → CaSO4 + H2O + CO2.
3 Discussion
The petrographic analysis of the building rocks used in Dougga allowed the characterization of four main lithotypes (L1, L3, 2F and 2G) that have been identified in the monument and in the stratigraphic log, and sampled from the historical quarries (Figs. S2, S4 and S5). These quarry rock samples, in turn, allowed establishing most important petrophysical characteristics of the rock used in the construction of Dougga.
The results show that the most common building rock used in the construction of Dougga corresponds to an Eocene nummulitic limestone extracted from local quarries located in the vicinity of the city (Figs. S2, S3 and S5). Most important pieces in terms of rock size (i.e. dimension stone), which were those used to make ashlars for walls, floors and columns, are preferentially made of washed nummulitic grainstones without terrigenous components (Figs. S5 and S7, Table SM1). Specifically, lithotypes L1, 2F and 2G were used in the construction of the base of the walls, while lithotype L3 was mainly used for the elaboration of columns and floors, maybe for esthetical purpose, since the large size of the nummulites and their density confer to the rock a very good appearance and undoubtedly because of the large thickness of the strata. Other limestone textures identified in the stratigraphic log and quarries as packstones and mudstones were rarely used to perform ashlars, but were preferentially used as rubble stone in the construction of some walls (Fig. 2C, and S5; Table SM1).
Petrographic data show that large ashlars corresponding to lithotypes L1, 2F and 2G were extracted from specific beds in the same quarry, most probably from the Lower quarry, as suggested by the size of the blocks, which is directly related to the thickness of the individual layers (Figs. S4 and S5). Analytical data show that very small differences in the petrographical features such as porosity and fabrics of the studied lithotypes have an impact on their physical and durability behaviours.
The total porosity for all lithotypes ranges between 2 and 4%, whereas a majority of pore size radii are < 0.1 μm. This kind of pore size distribution induces a very slow dynamic mobility of solutions inside the rock (Benavante, 2011; Buj and Gisbert, 2010; McGreevy, 1996; Pédro, 1983, 1993; Pédro and Delmas, 1983; Ruedrich and Siegesmund, 2007). The secondary porosity created by dissolution is mostly closed as suggested by the low values calculated by MIP and by the hydrostatic method. Moreover, the low connectivity of the pore system is confirmed by OFM observations
The studied rocks are characterised by a very low saturation rate and capillary absorption (Fig. 3). These low saturation values are related with the low pore size of the rock. Indeed, the water transport properties depend on the pore size distribution, as demonstrated by Winkler (1975). As it was expected, at almost similar porosity values L1 (2.6) and L3 (2.03), the smaller the pore diameter, the higher the capillary coefficient for the sample. Moreover, at similar pore size distribution, the more porous the rock, the higher the capillary coefficient (L3 and 2F).
The studied lithotypes display equally a high drying kinetics (Fig. 4). The highest drying rate of 2G can be explained by the type of pore size distribution (Fig. S6). Lithotype 2G displays more pore ranges than lithotypes 2F. Therefore, this more continuous pore size distribution facilitates fluid transfer, as demonstrated by Fitzner and Snethlage (1982).
All lithotypes show a very high resistance to compressive strength (Table SM3), which is interpreted as resulting from their high degree of cementation, their scare porosity, and their very small pore size (Table SM2). A mineralogy almost exclusively made of calcite, in turn, is the main reason for the very low resistance to abrasion observed in the studied samples (Table SM3). Several authors demonstrated the relationship between material characteristics such as degree of cementation, grain size distribution (Zoghlami and Gómez-Gras, 2004), rock fabric (Siegesmund, 1996), and porosity, on the one hand, and the mechanical properties of the material, on the other hand. Ruedrich and Siegesmund (2007) demonstrated that porosity controls the mechanical and elastic properties of the rock. In the current work, it seems that the variation of the compression resistance between lithotypes is tightly linked to pore volume and pore size distribution. In fact, the highest resistance of lithotype L1 can be explained by its low porosity and its smallest pore size compared with the other lithotypes.
Variations in the petrographic characteristics of the studied samples displayed by this study (Fig. S3; Table SM1) have a clear influence on their physical properties: (i) lithotypes presenting low porosity values and smallest pore size distribution (L1) have higher resistance to compressive strength (Tables SM2 and SM3); (ii) lithotypes presenting a continuous pore size distribution (2G) have a higher drying velocity and very low water retention compared to the other ones (Table SM2; Figs. 3 and 4); (iii) lithotypes presenting a high percentage of intraparticle porosity (L3) retain more water (Figs. 3 and 4).
In ice crystallization ageing tests, the difference in values between the initial and final apparent volume shows a slight decrease, probably related to a possible increase in porosity, which typically occurs in rocks after the ageing test (Fort et al., 2008; Momeni et al., 2015; Ruedrich and Siegesmund, 2007). Lithotype L3 is less affected thanks to its low porosity and its lowest degree of saturation (Ws) compared to the other lithotypes, which reduces ice crystallization in the pore network.
The high resistance to the ice crystallization ageing test observed in all samples (Table SM4) can be attributed particularly to the high percentage of pore radii < 0.1 μm. This pore radius (i.e. pore access) is thought to control the uptake and transport of fluids within a stone, as discussed by Ruedrich and Siegesmund (2007). The high mechanical strength and their hydric properties characterised by high drying rate and low retention play a key role in conferring such resistance to the rock against frost action. Ruedrich and Siegesmund (2007) studied the role of the water content in the decay process by ice crystallisation of sandstone rocks and demonstrate that at water saturation values < 20%, no decay occurs. In the current study, the water content at saturation (Ws) is about 0.77 to 1.62%, suggesting that, despite the amount of the microporosity, the effect of ice crystallisation on the rock is low to generate a disruptive stress because of the high mechanical resistance of the studied stones. Despite the visible high resistance to ice crystallization of these rocks, we cannot rule out the possibility of internal decay, as suggested by Freire-Lista et al. (2015) in their study of freeze-thaw fracturing in granites. The latter authors demonstrated that the ice crystallisation ageing test commonly resulted in an increase in inter- and intracrystalline microcracks, whose density and volume increases with the number of cycles. A similar finding was reported by Sousa et al. (2005), who detected intracrystalline cracks on feldspar crystals that formed part of ornamental granites located. Based on scanning electron microscopy analysis, Manchao et al. (2010) demonstrated that these microcracks often appeared in the cleavage direction.
The resistance of the rock to the SO2 ageing test (Table SM5) can be also attributed to its high content of micropores, as pointed out by Ross et al. (1989) and Olaru et al. (2010). In a comparative study of the ageing test by SO2 applied to carbonate rocks, the latter authors demonstrated that limestone are more sensible to alteration because of its higher porosity compared to marble, despite they have the same mineralogical and chemical composition. It is widely accepted that dissolved sulphate is carried in solution into the stone through the pore space (Ross et al., 1989). Indeed, in the present study, the ageing test simulates an atmosphere where the SO2 penetrates mixed with water vapour into the stone pore network due to the high relative humidity of the ageing environment, instead of penetrating directly as a pure gas phase. Thus, the low water saturation of the studied rocks likely reduced the dynamic mobility of solutions inside the pore network, conferring it high resistance against this SO2 ageing test, despite its carbonate nature.
The salt-crystallisation ageing test has not been performed in this study, but a high degree of resistance of the studied rocks to salt decay phenomena can be predicted from the low porosity and the type of the pore size distribution of these rocks, as previously reported (e.g., Buj and Gisbert, 2010; Everett, 1961; Honeyborne and Harris, 1958). The latter authors demonstrated that rocks with a high percentage of pore throats radii < 0.1 μm are less susceptible to salt decay than rocks with higher pore size. Consequently, pore radii < 0.1 μm are known to be less accessible to salt dissolved in water (Winkler, 1975) and so unchanged during the salt crystallization weathering test (Fitzner and Snethlage, 1982). As pointed out by Benavante (2011), stress caused by salt crystallization is considered for pore sizes from 0.1 to 10 μm. As a synthesis, we assume that the studied rocks are most probably resistant to salt decay because of their high mechanical resistance, their low porosity and saturation values and their very small pore size access. In fact, the columns of the Capitol of Dougga are very high monolithic pillars submitted to an overload (large weight supported by a small area). On the one hand, if we observe the exploitation level of these columns in the ancient quarries, we can note that the pillars are oriented against the bedding planes. This orientation favours the splitting of the rock in this direction (Siegesmund, 1996). On the other hand, petrographic characterization shows that in this lithotype (L3) nummulites are big sized and aligned with each other, creating a structural anisotropy that causes a decrease in the mechanical strength and so a split into several parts along these planes of weakness. Several authors noted the impact of anisotropies on the durability of building rocks, the relationship between anisotropy, and resistance to decay (Fort et al., 2008, 2011, Freire-Lista et al., 2015; Siegesmund, 1996). These authors demonstrated that when anisotropy increases, the resistance to decay decreases. Likewise, pre-existence microcracks, their orientation and distribution in the quarry may play a key role in a development of anisotropy and so splitting and fragmentation in columns as demonstrated by Freire-Lista and Fort (2017).
3.1 Regional comparisons
The nummulitic limestones used as building rock in the Roman city of Dougga present an acceptable state of preservation when compared with equivalent nummulitic limestones used in other historical constructions throughout the Mediterranean area. In particular, the nummulitic limestones used in Egyptian monuments such as the pyramids of Giza and other historical buildings are much altered, as extensively described in the literature (Aly et al., 2015; Elhefnawi, 1998; Liritzis et al., 2008). According to these authors, the alterability of the pyramid's limestone is essentially linked to the presence of salts coming from the air pollution and the rising of humidity by capillary from the water table which level reach the ground floor of the monuments. As discussed in the previous section, the geographic location of Dougga in the country side (i.e. far from polluted and intense traffic) and its geological setting favour that salts are rarely affecting buildings rocks.
In terms of rock quality, two main factors can be claimed to be the origin of the differences in the alterability in the nummulitic limestones between the Egyptian and Dougga case studies: (i) mineralogy and (ii) porosity. The nummulitic rocks of Egypt are composed of both limestones and dolostones, implying the presence of a range of carbonates minerals, like calcite (CaCO3), ankerite (Ca(Mg, Fe, Mn)(CO3)2), and dolomite CaMg(CO3)2, (Fitzner et al., 2002). According to these authors, quartz, opaque minerals and salt minerals are equally present. Contrary to Egypt nummulitic rock, the mineralogy of the nummulitic limestones of Dougga is more homogeneous, since they are mainly comprised of calcite, which may favour a higher degree of resistance to decay and so explain the better conservation state in the field.
According to Elhefnawi (1998) and Fitzner et al. (2002), the Egyptian nummulitic rocks are biomicrites or poorly washed biosparite according to Folk (1974), or wackestone to mudstone according to the classification of Dunham (1962). By contrast, Dougga's rocks are biosparite or well-washed grainstones, suggesting that their high degree of cementation by calcite results in higher resistance to decay than in the case rocks with a muddy matrix.
A most important difference between Dougga and Egypt case studies, however, lays in the porous network of the nummulite limestone (Table SM6). The Egyptian rock shows higher porosity (12–37%), with a majority of pores with a radius ranging between 0.1 and 10 μm, and reaching sometimes 100 μm, which is favourable to salt decay according to Benavante (2011). By contrast, Dougga's rock shows very low total porosity values (2.03–4.06%) (Table SM2) with pore radii ranging between 0.005 and 0.1 μm for all lithotypes (unfavourable to salt decay phenomena). Thus, the Dougga and Egyptian Eocene nummulitic limestone are mainly different by their fabric, amount of porosity, and pore size distribution. These small variations on the petrographic characteristics seem to have a great impact on their respective durability.
Nevertheless, salts in Dougga are almost missing on rocks. But even if salts are present in Dougga, the rock will not be affected because of its high mechanical strength (60 to 91 MPa), of its low porosity (≤ 4%), which implies a very low saturation rate, and of its pore size distribution (pore size < 0.1 μm) which prevents fluid circulation inside the rock.
Another documented case study of nummulitic limestones used as building rock in the Mediterranean area is the nummulitic stone commercially named “Piedra de Girona” has been used in the construction of important buildings like the Cathedral of Girona (Esbert et al., 1989). In this case, the mineralogical content of the Spanish and the Tunisian Eocene nummulitic limestones are very similar, but slightly differs regarding petrographic characteristics (fabric, porosity, and pore size distribution) (Table SM6). From a mineralogical point of view, the Girona limestone is made mostly of calcite (82.9%) but also presents minor proportions of feldspar (9.6%), quartz (5.1%), and dolomite (2.4%). Nevertheless, its mechanical resistance is very high (165 MPa), which improves its resistance to ice crystallization. Its decay processes are probably tightly linked to its fabric and its pore size distribution, which are specifically favourable to ice and salt crystallization decay (Table SM6).
4 Conclusions
Comparing petrographic characteristics from the lithotypes sampled on Dougga archaeological site and samples from historical quarries allow the identification of exploitation levels of the four recognized lithotypes.
All lithotypes identified by the petrographic study correspond to a grainstone of nummulites mainly comprised of calcite. The total porosity for all lithotypes ranges between 2 and 4%. They mainly differ by the size of their fossils, the percentage of the detritic fraction and their pore size distribution. These petrographic differences generate some little variation in the hydric and mechanical behaviour of the studied lithotypes.
In general, the studied rocks present a very good hydric behaviour characterized by very low water absorption rate, very fast drying, and almost no retention, with the exception of lithotype L3. Rocks are characterised also by a high mechanical resistance, as shown using compressive strength testing. Nevertheless, it displays a low resistance to abrasion, which explains in part the superficial erosion of the columns.
The current study shows that Dougga building rocks have a very high resistance against ice crystallization and acid attack. The low porosity of the limestone, its pore size distribution and its fabric can be considered as key factors for the durability of this rock. The present study highlights the high performance of Dougga's building stones in different climate conditions compared to similar rocks (age, type, and composition) used in the architectural heritage around the Mediterranean area.
The fissures observed in the columns of the Capitol cannot be the result of an aggressive environment as demonstrated in this study. On the contrary, these disjunctions are probably related to the mining of the rock and elaboration of the columns from specific beds, which can enhance cracking along weakness plans (bedding plans and fabric anisotropy due to the orientation of the nummulites) since the load above is very high.
This exhaustive petrophysical study of Dougga's building materials can help and guide professionals in the choice of methodology and techniques to carry out successful future conservation and restoration works on the monument. Indeed, based on the petrographic and petrophysical characteristics, the identification of the four lithotypes used in the construction of Dougga and their location in the ancient quarries strongly facilitate future restoration works. Petrographic differences between lithotypes must be considered, since they induce differential petrophysical properties. The choice of substitution materials such as rocks or restoration mortars and conservation products should be based on the results of the current work to avoid incompatibility problems that represent the principal factor of decay for the majority of the Tunisian monuments and historical buildings. Nevertheless, a specific research concerning the origin of the decay patterns of columns of the Capitol should be carried out. Indeed, it is interesting to know if the anisotropy generated by the orientation of the nummulites detected in this work is the unique origin of the fissures observed on the columns, or if there are other factors, such as the pre-existence of microcracks.
Acknowledgements
This research was funded by the “Agencia Española de Cooperación Internacional” (AECI, Spain) and the “Ministère de l’Enseignement supérieur et de la Recherche scientifique” (Tunis). Additional funding was provided by the Sedimentary Geology Research Group of the "Generalitat de Catalunya"GauriK.L. (2014SGR251). The authors would like to acknowledge the “Institut national du patrimoine of Tunis” (INP) and Mrs. Aicha Ben Abed for their support, and Dr. Juan Ramon Rosell for its collaboration in the performance of the mechanical and ice crystallization ageing tests at the “Departament de Construccions Arquitectòniques II” (“Escola Politècnica Superior d’Edificació de Barcelona”). Special thanks go to Dr. Enrique Romero of the “Universitat de Barcelona” for its collaboration in the monitorisation of mercury intrusion porosimetry analysis.