[De l’échelle du laboratoire aux paysages planétaires, quelles sont les empreintes laissées par des écoulements de surface sur des substrats solides en ablation soumis à des changements de phase ?]
Les processus en ablation, tels que les changements de phase, physiques (sublimation/fusion) ou chimiques (dissolution), sont étudiés dans de nombreuses applications techniques en physique. Pour les interfaces solide/fluide, l’interaction entre un changement de phase et un écoulement peut conduire à la formation de motifs topographiques. Dans ce cas, la mécanique des fluides associée à de tels changements de phase joue un rôle primordial dans l’évolution des paysages terrestres et planétaires, observés par les sondes en orbite autour des planètes et des lunes. Sur Terre, la glace de mer, les glaciers et les plateaux karstiques s’étendent sur des mètres ou des kilomètres. L’échelle de ces paysages contraste avec l’échelle des mécanismes physiques qui régissent leur dynamique évolutive. C’est en effet la taille typique des couches limites atmosphériques ou des films d’eau de fonte/soluté qui limite le transfert de chaleur/concentration à l’interface de changement de phase/dissolution, et donc le taux d’ablation du solide. Dans de nombreuses situations, ces couches sont contrôlées par l’écoulement du fluide, de type convection naturelle ou forcée. Dans le premier cas, la flottabilité de la fonte/dissolution elle-même, entraîne une stratification en densité causée par des gradients de température/concentration, qui peut être stable ou instable. Dans le second cas, l’écoulement forcé par des vents ou des pentes peut être considéré comme un écoulement de hauteur infinie ou finie, tels que les écoulements à surface libre. Dans tous les cas, le flux de masse modifie la topographie, qui en retour impacte les écoulements de couches limites (fines ou épaisses) et donc le taux d’ablation de manière rétroactive. Dans la nature, la rétroaction positive entre la géométrie et le transfert de masse entraîne la formation spontanée de formes caractéristiques à différentes échelles. Ces formes ne sont pas seulement des curiosités géologiques, comme le sont par exemple les « Zen stones », ces pierres sur un piédestal de glace ou encore les cônes de poussière, mais des marqueurs des processus hydrodynamiques en jeu. De nombreux paysages sont façonnés selon des motifs réguliers et répétés, qu’ils soient pointus, en forme de cupules, à ligne de crêtes parallèles entre elles ou encore en marches d’escaliers. Dans ce papier de synthèse, nous recensons les études expérimentales dédiées à l’étude des motifs générés par différents modes de transport des flux sur des substrats solides soumis à des changements de phase physiques ou chimiques. Nous souhaitons ainsi mettre en avant le rôle du mode de transport des flux dans la diversité des motifs observés sur des matériaux analogues. Comprendre la diversité de ces motifs est essentiel pour évaluer les conditions environnementales dans lesquelles ils se forment, notamment sur des planètes comme Mars ou Pluton sur lesquelles les changements de phase jouent un rôle géomorphologique majeur.
Physical or chemical phase changes in ablation, such as sublimation, melting or dissolution, are studied in physics for their many engineering applications. At solid/fluid interfaces, the interaction between a phase change and a flow can lead to pattern formation. In this case, the fluid mechanics associated with such phase changes play a key role in the evolution of terrestrial and planetary landscapes, observed by probes orbiting planets and moons. On Earth, sea ice, glaciers and karst plateaus extend over meters or kilometers. The scale of these landscapes contrasts with the scale of the physical mechanisms that govern their evolutionary dynamics. Indeed, it is the typical size of atmospheric boundary layers or meltwater/vapor/solute films that constrain the heat/concentration transfer at the phase change/dissolution interface, and hence the rate of solid ablation. In many situations, these layers are controlled by fluid flow, either natural or forced convection. In the former case, the flow may be buoyancy driven by the melting/dissolution/sublimation itself, resulting in density stratification caused by, for example, temperature/concentration gradients. This stratification may be stable or unstable. In the second case, the flow forced by winds or slopes can be considered as a flow of an infinite height or of a finite height, such as shallow water flow. In all cases, the mass flux modifies topography, which in turn affects the boundary layer flows and thus the ablation rate in a retroactive way. In nature, the positive feedback between geometry and mass transfer drives the spontaneous formation of characteristic patterns at different scales. These patterns are not just geological curiosities, such as Zen stones or dirt cones but markers of the hydrodynamic processes at work. Many landscapes are shaped by regular, repeated patterns, whether sharp-edged, scalloped, parallel-crested, or stepped. By experimentally investigating different modes of flow transport on solid substrates undergoing physical or chemical phase change, this review aims to highlight the role of the flow transport mode in the diversity of patterns observed on analogous materials. Understanding the diversity of these patterns is key to assessing the environmental conditions under which they form on planets such as Mars or Pluto, where phase changes play a very important geomorphological role.
Révisé le :
Accepté le :
Première publication :
Mots-clés : Sublimation, Fusion, Dissolution, Motifs solides naturels, Analogues expérimentaux, Formation de motifs, Classification
Sabrina Carpy 1 ; Michael Berhanu 2 ; Martin Chaigne 2 ; Sylvain Courrech du Pont 2
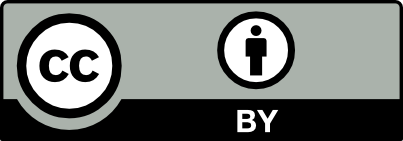
@article{CRPHYS_2024__25_S3_A1_0, author = {Sabrina Carpy and Michael Berhanu and Martin Chaigne and Sylvain Courrech~du Pont}, title = {Fingerprints of surface flows on solid substrates ablated by phase change: from laboratory experiments to planetary landscapes}, journal = {Comptes Rendus. Physique}, publisher = {Acad\'emie des sciences, Paris}, year = {2024}, doi = {10.5802/crphys.230}, language = {en}, note = {Online first}, }
TY - JOUR AU - Sabrina Carpy AU - Michael Berhanu AU - Martin Chaigne AU - Sylvain Courrech du Pont TI - Fingerprints of surface flows on solid substrates ablated by phase change: from laboratory experiments to planetary landscapes JO - Comptes Rendus. Physique PY - 2024 PB - Académie des sciences, Paris N1 - Online first DO - 10.5802/crphys.230 LA - en ID - CRPHYS_2024__25_S3_A1_0 ER -
%0 Journal Article %A Sabrina Carpy %A Michael Berhanu %A Martin Chaigne %A Sylvain Courrech du Pont %T Fingerprints of surface flows on solid substrates ablated by phase change: from laboratory experiments to planetary landscapes %J Comptes Rendus. Physique %D 2024 %I Académie des sciences, Paris %Z Online first %R 10.5802/crphys.230 %G en %F CRPHYS_2024__25_S3_A1_0
Sabrina Carpy; Michael Berhanu; Martin Chaigne; Sylvain Courrech du Pont. Fingerprints of surface flows on solid substrates ablated by phase change: from laboratory experiments to planetary landscapes. Comptes Rendus. Physique, Online first (2024), pp. 1-48. doi : 10.5802/crphys.230.
[1] Wind-tunnel modelling of dispersion from a scalar area source in urban-like roughness, Bound.-Layer Meteorol., Volume 126 (2008) no. 23, pp. 103-124 | DOI
[2] Development of naphthalene PLIF for visualizing ablation products from a space capsule heat shield, 52nd Aerospace Sciences Meeting, American Institute of Aeronautics and Astronautics, Reston, 2014 AIAA 2014-1152. Online at https://arc.aiaa.org/doi/10.2514/6.2014-1152 (accessed January 31, 2025) | DOI
[3] Analysis of the TATER nosetip boundary layer transition and ablation experiment, 14th Aerospace Sciences Meeting, American Institute of Aeronautics and Astronautics, Reston, 1976 AIAA 1976-167. Online at https://arc.aiaa.org/doi/abs/10.2514/6.1976-167 (accessed January 31, 2025) | DOI
[4] Laminar-turbulent transition on reentry capsules and planetary probes, J. Spacecr. Rockets, Volume 43 (2006) no. 6, pp. 1153-1173 | DOI
[5] On the formation of regmaglypts on meteorites, Fluid Dyn. Res., Volume 1 (1987) no. 3-4, pp. 191-199 | DOI
[6] Size of scallops and ripples formed by flowing water, Nature, Volume 277 (1979), pp. 281-283 | DOI
[7] The Spiral troughs of Mars as cyclic steps, J. Geophys. Res.: Planets, Volume 118 (2013) no. 9, pp. 1835-1857 | DOI
[8] Penitentes as the origin of the bladed terrain of Tartarus Dorsa on Pluto, Nature, Volume 541 (2017) no. 7636, pp. 188-190 | DOI
[9] Ice sublimation as a geomorphic process: A planetary perspective, Geomorphology, Volume 126 (2011) no. 1-2, pp. 1-17 | DOI
[10] Cryosphere during the twentieth century, Geophys. Monogr. Ser., Volume 150 (2004), pp. 239-257 | DOI
[11] Scaling laws for ablation waves formed by ice sublimation and rock dissolution: applications to the Earth, Mars and Pluto, Front. Astron. Space Sci., Volume 10 (2023), 1176158 | DOI
[12] Karst Hydrogeology and Geomorphology, John Wiley & Sons, Springer, Netherlands, 2007 | DOI
[13] Incidence of saline deposits in geologic time, Saline Deposits: A Symposium based on Papers from the International Conference on Saline Deposits, Houston, Texas, 1962 (R. B. Mattox; W. T. Holser; H. Ode; W. L. McIntire; N. M. Short; R. E. Taylor; D. C. Van Siclen, eds.), Geological Society of America, New York, 1968, pp. 43-57 | DOI
[14] Fluid dynamic instabilities: Theory and application to pattern forming in complex media, Philos. Trans. R. Soc. A: Math. Phys. Eng. Sci., Volume 375 (2017) no. 2093, pp. 1-44 | DOI
[15] A simulation study of the development of Rillenkarren, Earth Surf. Process., Volume 5 (1980) no. 1, pp. 25-36 | DOI
[16] Laboratory modeling of karst phenomena and their rock relief on plaster, subsoil karren, rain flutes karren and caves, Acta Carsologica, Volume 45 (2016) no. 2, pp. 187-204 | DOI
[17] Streamwise dissolution patterns created by a flowing water film, Phys. Rev. Lett., Volume 125 (2020) no. 19, 194502 | DOI
[18] Bed forms due to mass transfer in turbulent flows: a kaleidoscope of phenomena, J. Fluid Mech., Volume 49 (1971) no. 1, pp. 49-63 | DOI
[19] Analysis of scallop patterns by simulation under controlled conditions, J. Geol., Volume 80 (1972) no. 1, pp. 121-122 | DOI
[20] Experimental and theoretical studies of dissolution roughnesses, J. Fluid Mech., Volume 65 (1974) no. 4, pp. 735-751 | DOI
[21] Buoyancy driven dissolution of inclined blocks: Erosion rate and pattern formation, Phys. Rev. Fluids, Volume 5 (2020), 053802 | DOI
[22] Dissolution instability and roughening transition, J. Fluid Mech., Volume 832 (2017) no. R2, pp. 1-14 | DOI
[23] Ice ripple formation at large Reynolds numbers, J. Fluid Mech., Volume 694 (2012), pp. 225-251 | DOI
[24] Cyclic steps on ice, J. Geophys. Res. F: Earth Surf., Volume 121 (2016) no. 5, pp. 1023-1048 | DOI
[25] The hydrodynamic genesis of linear karren patterns, J. Fluid Mech., Volume 913 (2021), A34 | DOI
[26] Boundary-layer approach to the linear karren instability, J. Fluid Mech., Volume 977 (2023), R3 | DOI
[27] Stability of dissolving or depositing surfaces, AIChE J., Volume 25 (1979) no. 4, pp. 697-701 | DOI
[28] Steady states and linear stability analysis of precipitation pattern formation at geothermal hot springs, Phys. Rev. E, Volume 76 (2007) no. 4, pp. 1-11 | DOI
[29] Hydrodynamic-driven stability analysis of morphological patterns on stalactites and implications for cave paleoflow reconstructions, Phys. Rev. Lett., Volume 108 (2012) no. 23, pp. 1-5 | DOI
[30] Ripples on the underside of river ice covers, J. Hydraul Eng., Volume 98 (1972), pp. 1603-1624
[31] Turbulent heat transfer to wavy boundaries, Proceedings of the Heat Transfer and Fluid Mechanics Institute, 1972, pp. 200-213
[32] et al. Sublimation waves: Geomorphic markers of interactions between icy planetary surfaces and winds, Earth-Sci. Rev., Volume 211 (2020), 103350 | DOI
[33] Sedimentary Structures, Their Character and Physical Basis (J. R. L. Allen, ed.), Developments in Sedimentology, 30, Elsevier, Amsterdam, Oxford, New York, 1982, 592 pages
[34] On the formation of snow penitentes, J. Glaciol., Volume 3 (1958) no. 24, pp. 304-311 | DOI
[35] Seasonal evolution of penitente glaciochemistry at Tapado Glacier, Northern Chile, Hydrol. Process., Volume 30 (2016) no. 2, pp. 176-186 | DOI
[36] Ablation at high altitudes and under high solar incidence, Am. J. Sci., Volume 239 (1941) no. 5, pp. 379-382 | DOI
[37] et al. Bladed Terrain on Pluto: Possible origins and evolution, Icarus, Volume 300 (2018), pp. 129-144 | DOI
[38] Formation of metre-scale bladed roughness on Europa’s surface by ablation of ice, Nat. Geosci., Volume 11 (2018) no. 12, pp. 901-904 | DOI
[39] Penitente formation is unlikely on Europa, Nat. Geosci., Volume 13 (2020) no. 1, pp. 17-19 | DOI
[40] Reply to: Penitente formation is unlikely on Europa, Nat. Geosci., Volume 13 (2020) no. 1, pp. 20-21 | DOI
[41] The origin of penitents, J. Glaciol., Volume 2 (1954) no. 15, pp. 331-338 | DOI
[42] Theory of structure formation in snowfields motivated by penitentes, suncups, and dirt cones, Phys. Rev. E, Volume 63 (2001) no. 5, 056129 | DOI
[43] Micro-morphology of the snow surface at the Quelccaya ice cap, Peru, J. Glaciol., Volume 27 (1981) no. 97, pp. 423-428 | DOI
[44] Ablation of snow-fields at high altitudes by radiant solar heat, Trans. Am. Geophys. Union, Volume 15 (1934), 380 | DOI
[45] Theory of structure formation in snowfields motivated by penitentes, suncups, and dirt cones, Phys. Rev. E, Volume 63 (2001) no. 5, pp. 1-12 | DOI
[46] Physical processes causing the formation of penitentes, Phys. Rev. E, Volume 92 (2015) no. 3, 033015 | DOI
[47] Flow dynamics and shape of rinnenkarren systems, Geomorphology, Volume 198 (2013), pp. 115-127 | DOI
[48] Ultra-sharp pinnacles sculpted by natural convective dissolution, Proc. Natl. Acad. Sci. USA, Volume 117 (2020) no. 38, pp. 23339-23344 | DOI
[49] Formation d’ondes topographiques par transfert de masse et diffusion dans un écoulement turbulent : analyse d’exemples naturels terrestres et planétaires et modélisations, PhD thesis, Nantes Université (2020)
[50] On the glaciological, meteorological, and climatological significance of Antarctic Blue Ice areas, Rev. Geophys., Volume 37 (1999) no. 3, pp. 337-359 | DOI
[51] Observed configuration and computed roughness of the underside of rive ice, St. Croix River, Wisconsin, US Geol. Survey Prof. Pap., Volume 550 (1966), p. B192-B198 | DOI
[52] The tsingy karrenfields of Madagascar, Karst Rock Features: Karren Sculpturing (A. Ginés; M. Knez; T. Slabe; W. Dreybrodt, eds.), Volume 9, Karst Research Institute, Založba ZRC, 2009, pp. 411-422
[53] The pinnacle karrenfields of Mulu, Karst Rock Features. Karren Sculpturing (A. Ginés; M. Knez; T. Slabe; W. Dreybrodt, eds.), Volume 9, Karst Research Institute, Založba ZRC, 2009, pp. 423-432
[54] Lithologic and morphological properties and rock relief of the Lunan stone forests, Karst Rock Features. Karren Sculpturing (A. Ginés; M. Knez; T. Slabe; W. Dreybrodt, eds.), Karst Research Institute, Založba ZRC, 2002, pp. 259-266
[55] Karrenfield Landscapes and Karren Landforms, Karst Rock Features, Karren Sculpturing (A. Ginés; M. Knez; T. Slabe; W. Dreybrodt, eds.), Volume 9, Karst Research Institute, Založba ZRC, 2009, pp. 13-24
[56] Salt karst, Treatise on Geomorphology, Volume 6, Academic Press, San Diego, CA, 2013, pp. 407-424 | DOI
[57] Mode of formation of “ablation hollows” controlled by dirt content of snow, J. Glaciol., Volume 33 (1987) no. 114, pp. 135-139 | DOI
[58] Mars global surveyor Mars orbiter camera: Interplanetary cruise through primary mission, J. Geophys. Res., Volume 106 (2001) no. E10, pp. 23429-23570 | DOI
[59] Surface textures of Mars’ north polar layered deposits: A framework for interpretation and future exploration, Mars J., Volume 2 (2006), pp. 21-45 | DOI
[60] et al. Crater population and resurfacing of the Martian north polar layered deposits, J. Geophys. Res. E: Planets, Volume 115 (2010) no. 8, pp. 1-11 | DOI
[61] Martian polar and circum-polar sulfate-bearing deposits: Sublimation tills derived from the North Polar Cap, Icarus, Volume 209 (2010) no. 2, pp. 434-451 | DOI
[62] et al. Dunes on Pluto, Science, Volume 360 (2018) no. 6392, pp. 992-997 | DOI
[63] Morphology of ice surfaces in the Dobšiná Ice Cave, Proceedings of the 2nd International Workshop on Ice Caves (S. Turri; J. Zelinka, eds.), Slovak Caves Administration, Liptovský Mikulá, 2006, pp. 15-23 Online at http://www.ssj.sk/en/user_files/IWIC_2_15.pdf (accessed January 31, 2025)
[64] Ice Caves (A. Persoiu; S.-E. Lauritzen, eds.), Elsevier Inc., Amsterdam, Oxford, Cambridge, 2018, 752 pages
[65] The mass and energy balance of ice within the Eisriesenwelt cave, Austria, Cryosphere, Volume 5 (2011) no. 1, pp. 245-257 | DOI
[66] The interplay between air temperature and ice mass balance changes in Scărişoara Ice Cave, Romania, Acta Carsologica, Volume 40 (2011) no. 3, pp. 445-456 | DOI
[67] Scallops and flutes, Trans. Cave Res. Group of Great Britain, Volume 7 (1966) no. 2, pp. 121-160
[68] Ice in Coulthard Cave, Alberta, Canad. J. Earth Sci., Volume 11 (1974) no. 4, pp. 510-518 | DOI
[69] Geological pattern formation by growth and dissolution in aqueous systems, Proc. R. Soc. A: Math. Phys. Eng. Sci., Volume 466 (2010) no. 2115, pp. 659-694 | DOI
[70] The scalloping phenomenon and its significance in flow-assisted corrosion, Proceedings of the Twenty Sixth Annual CNS-CNA Student Conference, Canadian Nuclear Society, Toronto, 2001, pp. 1-10 Online at https://www.nuceng.ca/univcomm/studconf2001/S16.PDF (accessed January 31, 2025)
[71] Transverse erosional marks of mud and rock: their physical basis and geological significance, Sediment. Geol., Volume 5 (1971) no. 3-4, pp. 167-385 | DOI
[72] Stability of dissolution flutes under turbulent flow, J. Cave Karst Studies, Volume 73 (2011) no. 3, pp. 181-186 | DOI
[73] Bedforms in salt deposits of the Dead sea brines, J. Sediment. Res., Volume 57 (1987) no. 4, pp. 723-735 | DOI
[74] Detailed observations of the rippled surface of Antarctic blue-ice areas, J. Glaciol., Volume 47 (2001) no. 158, pp. 387-396 | DOI
[75] The heat and mass balance of snow dunes on the central Antarctic plateau, J. Glaciol., Volume 8 (1969) no. 53, pp. 277-284 | DOI
[76] The KARREN and KARREN formation of bare slopes, Earth-Sci. Rev., Volume 188 (2019), pp. 272-290 | DOI
[77] Rillenkarren, Karst Rock Features, Karren Sculpturing, Karst Research Institute, Zalozba ZRC, 2009, pp. 185-210
[78] et al. Ruissellement et dissolution dun bassin versant lapiazé des karsts de Patagonie, île Tarlton, archipel de Madre de Dios (Chili), Collection EDYTEM. Cahiers de géographie, Volume 19 (2017) no. 1, pp. 137-146 (Included in a thematic issue: Monitoring en milieux naturels. Retours d’expériences en terrains difficiles) | DOI
[79] The formation of sediment ripples, dunes, and antidunes, Annu. Rev. Fluid Mech., Volume 1 (1969), pp. 147-168 | DOI
[80] Incisional cyclic steps of permanent form in mixed bedrock-alluvial rivers, J. Geophys. Res.: Earth Surf., Volume 122 (2017) no. 1, pp. 130-152 | DOI
[81] Identification and mapping of rock weathering surface forms and features, Zeitschrift für Geomorphologie Supplementband, Volume 120 (2000), pp. 5-22
[82] The secret life of ice sails, J. Glaciol., Volume 63 (2017) no. 242, pp. 1049-1062 | DOI
[83] The formation of ice sails, Geophys. Astrophys. Fluid Dyn., Volume 111 (2017) no. 6, pp. 411-428 | DOI
[84] Onset of glacier tables, Phys. Rev. Lett., Volume 127 (2021) no. 10, 108501 | DOI
[85] Formation of glacier tables caused by differential ice melting: field observation and modelling, Cryosphere, Volume 16 (2022) no. 6, pp. 2617-2628 | DOI
[86] How dirt cones form on glaciers: Field observation, laboratory experiments, and modeling, Phys. Rev. E, Volume 107 (2023) no. 3, 034905 | DOI
[87] Ice melting under a thin layer of moraine, and the existence of ice cores in moraine ridges, Geografiska Annaler, Volume 41 (1959) no. 4, pp. 228-230 | DOI
[88] Ablation on debris covered glaciers: an example from the Rakhiot Glacier, Punjab, Himalaya, Snow Glacier Hydrol., Volume 218 (1993), pp. 289-296
[89] Glacial melt under a porous debris layer, J. Glaciol., Volume 61 (2015) no. 229, pp. 825-836 | DOI
[90] Solidification of fluids, Perspectives in Fluid Dynamics - A Collective Introduction to Current Research (G. K. Batchelor; H. K. Moffatt; M. G. Worster, eds.), Cambridge University Press, Cambridge, 2000, pp. 393-446 Online at http://www.itg.cam.ac.uk/people/grae/44.pdf (accessed January 31, 2025)
[91] Kinetics of Water–Rock Interaction (S. L. Brantley; J. D. Kubicki; A. F. White, eds.), Springer, New York, 2008 | DOI
[92] Mathematical Modeling of Melting and Freezing Processes, Hemisphere Publishing Corporation, Taylor & Francis, Washington DC, 1993 | DOI
[93] Thermodynamics and An Introduction to Thermostatistics, John Wiley & Sons, New York, Chichester, Brisbane, Toronto, Singapore, 1985
[94] The effects of curvature on the dissolution kinetics of spherical precipitates, Metal Sci. J., Volume 4 (1970) no. 1, pp. 222-225 | DOI
[95] Holographic interferometry study of the dissolution and diffusion of gypsum in water, Geochim. Cosmochim. Acta, Volume 71 (2007) no. 8, pp. 1913-1920 | DOI
[96] The evaporation coefficient of water: a review, Int. J. Heat Mass Transfer, Volume 40 (1997) no. 12, pp. 2963-2973 | DOI
[97] Modélisation physique du procédé de découpe de métaux par laser, PhD thesis (2003) https://api.semanticscholar.org/CorpusID:169339509
[98] Temperature dependence of the sublimation rate of water ice: Influence of impurities, Icarus, Volume 233 (2014), pp. 101-105 | DOI
[99] The classical field theories, Principles of Clasical Mechanics and Field Theory (S. Flügge, ed.) (Encyclopedia of Physics), Springer, New York, 1960 | DOI
[100] Hydrodynamic and Hydromagnetic Stability, Clarendon Press, Oxford, 1961
[101] The Handbook of Chemistry and Physics (D. R. Lide, ed.), CRC Press, Boca Raton, FL, 2004 | DOI
[102] Modeling binary alloy solidification by a random projection method, Numer. Methods Partial Differ. Equ., Volume 35 (2019) no. 2, pp. 733-760 | DOI
[103] Experimental studies of halite dissolution kinetics, 1 The effect of saturation state and the presence of trace metals, Chem. Geol., Volume 137 (1997) no. 8, pp. 201-219 | DOI
[104] Melting and dissolving, J. Fluid Mech., Volume 239 (1992), pp. 429-448 | DOI
[105] A synthesis of thermo-dynamic ablation at ice–ocean interfaces from theory, observations and models, Ocean Model., Volume 154 (2020), 101692 | DOI
[106] Laboratory experiments on ice melting: a need for understanding dynamics at the ice–water interface, J. Marine Sci. Eng., Volume 10 (2022) no. 8, 1008 | DOI
[107] Icebergs melting, Annu. Rev. Fluid Mech., Volume 55 (2023), pp. 377-402 | DOI
[108] Shaping of melting and dissolving solids under natural convection, J. Fluid Mech., Volume 900 (2020), A35 | DOI
[109] Solutal convection induced by dissolution, Phys. Rev. Fluids, Volume 4 (2019), 103801 | DOI
[110] The relationship between intrinsic dissolution rates and solubilities in the water–ethanol binary solvent system, Int. J. Pharm., Volume 18 (1984) no. 1-2, pp. 149-156 | DOI
[111] Emergence of tip singularities in dissolution patterns, Proc. Natl. Acad. Sci. USA, Volume 120 (2023) no. 48, e2309379120 | DOI
[112] Turbulent solutal convection and surface patterning in solid dissolution, Phys. Rev. E, Volume 54 (1996) no. 1, 486 | DOI
[113] Reactive flows in porous media: challenges in theoretical and numerical methods, Annu. Rev. Chem. Biomol. Eng., Volume 12 (2021) no. 1, pp. 543-571 | DOI
[114] Time-dependent shapes of a dissolving mineral grain: Comparisons of simulations with microfluidic experiments, Chem. Geol., Volume 540 (2020), 119459 | DOI
[115] Calcite dissolution under turbulent flow conditions: a remaining conundrum, Acta Carsologica, Volume 43 (2014) no. 1, pp. 195-202 | DOI
[116] Experimental study of the effect of wind on the stability of water ice on Mars, Icarus, Volume 196 (2008) no. 2, pp. 477-487 | DOI
[117] Classification of ablation mode during impact of hot liquid jet on a solid, Int. J. Heat Mass Transfer, Volume 181 (2021), 121883 | DOI
[118] Pattern selection in single-component systems coupling Bénard convection and solidification, J. Fluid Mech., Volume 144 (1984), pp. 133-151 | DOI
[119] Natural convection in phase change material: experimental study, Int. J. Heat Mass Transfer, Volume 183 (2022), 122047 | DOI
[120] Analog experiments on melting and contamination at the roof and walls of magma chambers, J. Volcanol. Geotherm. Res., Volume 129 (2004) no. 1, pp. 173-197 | DOI
[121] Melting and solidification of a pure metal on a vertical wall, J. Heat Transfer, Volume 108 (1986) no. 1, pp. 174-181 | DOI
[122] Modelling karst geomorphology on different time scales, Geomorphology, Volume 106 (2009), pp. 62-77 | DOI
[123] Thin-film induced morphological instabilities over calcite surfaces, Proc. R. Soc. A, Volume 471 (2015) no. 2176, 20150031 | DOI
[124] Measurement of the pure dissolution rate constant of a mineral in water, Geochim. Cosmochim. Acta, Volume 72 (2008) no. 23, pp. 5634-5640 | DOI
[125] Do dissolving objects converge to a universal shape?, Langmuir, Volume 31 (2014) no. 14, pp. 41145-41150 | DOI
[126] Shape dynamics and scaling laws for a body dissolving in fluid flow, J. Fluid Mech., Volume 765 (2015), pp. 1-10 | DOI
[127] Calcite dissolution kinetics in the system CaCO–HO–CO at high undersaturation, Geochim. Cosmochim. Acta, Volume 71 (2007) no. 6, pp. 1398-1410 | DOI
[128] Diffusion of sucrose in supersaturated solutions, J. Am. Chem. Soc., Volume 72 (1950) no. 7, pp. 3261-3267 | DOI
[129] Diffusion in supersaturated solutions. II. Glucose solutions, J. Am. Chem. Soc., Volume 75 (1953) no. 16, pp. 3900-3904 | DOI
[130] The naphthalene sublimation technique, Exp. Therm. Fluid Sci., Volume 4 (1991) no. 5, pp. 510-523 | DOI
[131] The maximum rate of sublimation of solids, AIChE J., Volume 8 (1962) no. 5, pp. 590-593 | DOI
[132] The Merck Index: An Encyclopedia of Chemicals, Drugs, and Biologicals, Royal Society of Chemistry, Great Britain, 2013
[133] Erosion patterns on dissolving and melting bodies (2015 Gallery of Fluid motion), Phys. Rev. Fluids, Volume 1 (2016), 050508 | DOI
[134] Self-sculpting of a dissolvable body due to gravitational convection, Phys. Rev. Fluids, Volume 3 (2018), 043801 | DOI
[135] Anomalous convective flows carve pinnacles and scallops in melting ice, Phys. Rev. Lett., Volume 128 (2022) no. 4, 044502 | DOI
[136] Mass transfer in laminar–boundary-layer flows with finite interfacial velocities, AIChE J., Volume 6 (1960) no. 3, pp. 410-414 | DOI
[137] The convective Stefan problem: Shaping under natural convection, J. Fluid Mech., Volume 915 (2021), A86 | DOI
[138] Sur la vitesse de dissolution des sels dans leurs solutions aqueuses, J. Phys. Théor. Appl., Volume 4 (1905) no. 1, pp. 17-26 | DOI
[139] Recherches sur la vitesse de dissolution des sels dans leurs solutions aqueuses, J. Chim. Phys. Phys.-Chim. Biol., Volume 2 (1904), pp. 245-306 | DOI
[140] Hydrodynamic approach to dissolution rate, Int. J. Clin. Pharm., Volume 3 (1981), pp. 129-144 | DOI
[141] The surface morphology of crystals melting under solutions of different densities, J. Cryst. Growth, Volume 92 (1988) no. 3-4, pp. 364-370 | DOI
[142] Melting driven by vigorous compositional convection, J. Fluid Mech., Volume 280 (1994), pp. 255-285 | DOI
[143] Onset of convection in a layer of water formed by melting ice from below, Phys. Fluids, Volume 11 (1968) no. 6, pp. 1263-1270 | DOI
[144] Turbulent convection and surface patterning in solid dissolution, Phys. Lett. A, Volume 206 (1995), pp. 49-53 | DOI
[145] Dynamics of surface patterning in salt-crystal dissolution, Phys. Rev. E, Volume 53 (1996) no. 6, pp. 5572-5575 | DOI
[146] Genesis and shape of natural solution cavities within salt deposits, Water Resour. Res., Volume 44 (2008) no. 11, W11409 | DOI
[147] Alcove formation in dissolving cliffs driven by density inversion instability, Phys. Fluids, Volume 34 (2022) no. 5, 054118 | DOI
[148] Surface dissolution and the development of scallops, Chem. Eng. Commun., Volume 192 (2005) no. 1, pp. 125-136 | DOI
[149] Time evolution of surface roughness in pipes due to mass transfer under different Reynolds numbers, Int. J. Heat Mass Transfer, Volume 103 (2016), pp. 661-671 | DOI
[150] Boundary-Layer Theory, McGraw Hill, New York, 1979
[151] Stability of surfaces that are dissolving or being formed by convective diffusion, Annu. Rev. Fluid Mech., Volume 13 (1981) no. 1, pp. 231-252 | DOI
[152] Forced-convection heat transfer from irregular melting wavy boundaries, J. Heat Transfer, Volume 101 (1979), pp. 598-602 | DOI
[153] Wave formation and heat transfer at an ice–water interface in the presence of a turbulent flow, J. Fluid Mech., Volume 99 (1980), pp. 619-640 | DOI
[154] Ice scallops: a laboratory investigation of the ice–water interface, J. Fluid Mech., Volume 873 (2019), pp. 942-976 | DOI
[155] Transport-controlled dissolution in an evolving fracture: The extended Purday solution and fracture flow tests, Water Resour. Res., Volume 57 (2021) no. 3, e2020WR029166 | DOI
[156] Numerical and experimental investigation of buoyancy-driven dissolution in vertical fracture, J. Geophys. Res.: Solid Earth, Volume 118 (2013) no. 5, pp. 2038-2048 | DOI
[157] On the role of gravity in dissolving horizontal fractures, J. Geophys. Res.: Solid Earth, Volume 128 (2023) no. 3, e2022JB025214 | DOI
[158] Dissolution regimes of a horizontal channel in a gravity field, Phys. Rev. Fluids, Volume 8 (2023) no. 12, 123902 | DOI
[159] Supraglacial streams and rivers, Annu. Rev. Earth Planet. Sci., Volume 47 (2019), pp. 421-452 | DOI
[160] Supraglacial channel inception: Modeling and processes, Water Resour. Res., Volume 51 (2015) no. 9, pp. 7044-7063 | DOI
[161] Controlled irradiative formation of penitentes, Phys. Rev. Lett., Volume 96 (2006) no. 9, 098502 | DOI
[162] et al. Ice structures, patterns, and processes: A view across the icefields, Rev. Modern Phys., Volume 84 (2012) no. 2, pp. 885-944 | DOI
[163] Intra-surface radiative transfer limits the geographic extent of snow penitents on horizontal snowfields, J. Glaciol., Volume 60 (2014) no. 219, pp. 147-154 | DOI
[164] Stability of a planar interface during solidification of a dilute binary alloy, J. Appl. Phys., Volume 35 (1964) no. 2, pp. 444-451 | DOI
[165] A quantitative assessment of dirt-cone dynamics, J. Glaciol., Volume 11 (1972) no. 63, pp. 431-446 | DOI
[166] Sublimation-driven morphogenesis of Zen stones on ice surfaces, Proc. Natl. Acad. Sci. USA, Volume 118 (2021) no. 40, e2109107118 | DOI
[167] Introductory notes on the changing geometry of cave structures, Cave Stud., Volume 11 (1959), pp. 69-90
[168] Pattern forming instabilities, Hydrodynamics and Nonlinear Instabilities (C. Godrèche; P. Manneville, eds.), Cambridge University Press, Cambridge, 2005
[169] Rayleigh–Bénard convection with a melting boundary, J. Fluid Mech., Volume 858 (2019), pp. 437-473 | DOI
Cité par Sources :
Commentaires - Politique