1 Introduction
The development of hemoglobin (Hb) based oxygen carriers (HBOC) as a blood substitute remains a challenge. However, specific problems are encountered with HBOCs due to the use of acellular Hb: the oxygen affinity, the dimerization and the irreversible auto-oxidation of the Hb [1]. In the past years, the regulation of oxygen affinity has been very well investigated and different studies have shown that it is possible to modulate the oxygen affinity of Hb [2–5]. In parallel, different molecules have been developed to prevent tetramer dissociation and renal toxicity either by chemical modification [6] or by protein engineering technology [1] but clinical trials with stabilized tetrameric Hb have shown an arterial hypertension and peripheral vasoconstriction [7]. This vasoconstriction is essentially attributed to scavenging of nitric oxide (NO) by Hb after extravasation through or close contact with the endothelium [8,9]. The increase of the molecular size of the carrier and its oxygen affinity has been proposed to reduce the undesirable vasoactive properties. Different approaches have been developed to increase the size of HBOC, such as surface modification of different Hbs by poly (ethylene) glycol (PEG) conjugation [10] or by glutaraldehyde polymerization [11]. Generally, these different Hb derivatives are a heterogeneous mixture of different polymers with small components, and may overcome the extravasation and vasoactive effects of acellular Hb. A PEG-Hb conjugate (MP4) has been developed with a molecular weight of 90 kDa and exhibiting a high oxygen affinity [12]. For the first generations of HBOC, the objective was to decrease the oxygen affinity in order to deliver quickly the oxygen to the tissue but the recent study with the MP4 molecule has shown that this macromolecule reduces precapillary oxygen release leading to a more efficient oxygen transport and may help limit the vasoconstriction of the arterioles as a regulatory mechanism for excess oxygen delivery [13,14]. In order to obtain a HBOC with appropriate size and correct oxyphoric capacity, we have developed the recombinant HbβG83C (rHbβG83C) which forms a stable octameric rHb of molecular mass 129 kDa (Fig. 1) with a slightly increased oxygen affinity relative to a Hb A solution [15]. We have previously reported that this octameric rHbβG83C is resistant toward potential disulfide reducing agents present in fresh human plasma and does not interact rapidly with haptoglobin, a glycoprotein that binds Hb (αβ) dimers as part of the elimination of Hb from blood circulation [15–18]. In contrast to other molecules produced by recombinant technology [19], rHbβG83 C exhibits a homogeneous size and does not dissociate into small molecular species at low concentration [15].
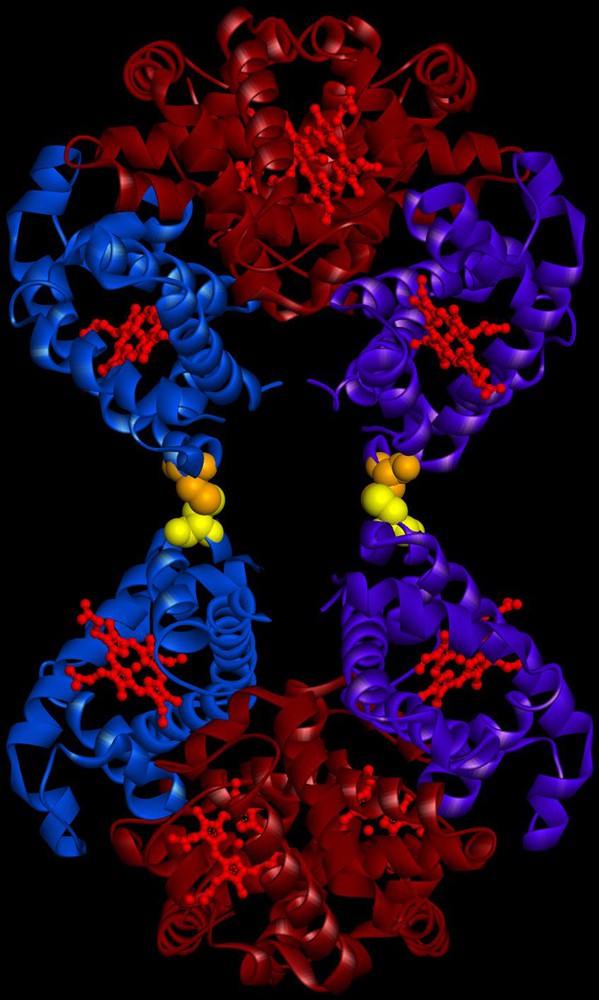
(Color online.) Schema of the octameric rHbβG83C, a dimer of tetramers joined by disulfide bonds at the β83 cysteines. The β chains in one tetramer are represented in blue and purple, respectively. The α chains and the heme molecules are represented in burgundy and in red respectively. The cysteines, introduced at position β83 are shown in yellow on one tetramer and orange on the other. This molecule was obtained using Accelrys software (Biovia, San Diego, CA, USA) with the crystallographic structure of tetrameric Hb A.
In this work, we explore the potential side effects of this octameric molecule on endothelial cells. It has been shown that certain HBOCs in contact with endothelial cells lead to oxidative stress, NO scavenging and inflammatory effects [20–22]. The inflammation induces the expression of molecules such as Intermolecular-Adhesion Molecule 1 (ICAM-1) and E-selectin [21,23]. The presence of oxidized Hb and the products of heme degradation as well as reactive oxygen species induce an oxidative stress response of endothelial cells by the expression of heme oxygenase 1 (HO-1) [23]. One of the major consequences of increased vascular reactive oxygen species production is a reduction of endothelial NO bioavailability [24]. This reduction of NO bioavailability is involved in the mechanism of vasoconstriction observed in vivo after the administration of HBOC [25]. Both NO synthesis and endothelial nitric oxide synthase (eNOS) transcriptional regulation are of interest as an increase in eNOS transcription could run counter to NO depletion in the circulation [21].
The aim of the present study was to evaluate the potential impacts of purified octameric rHbβG83C solution on Human Umbilical Vein Endothelial Cells (HUVEC) by assessing Hb effects on the markers of vasoactivity and inflammation and oxidative stress. The results were compared with those obtained with two reference HBOCs, Dex-BTC-Hb, a human Hb conjugated to dextran benzene tetracarboxylate (Dex-BTC) macromolecules [26] described with a decreased oxygen affinity and an increased vascular half-life [27], and Oxyglobin a glutaraldehyde-polymerized bovine Hb (Biopure Corporation, USA), as well as with those obtained with the control Voluven, a plasma expander solution.
2 Materials and methods
2.1 Preparation of HBOCs
The rHbβG83C was produced in BLi5 strains of Escherichia coli containing the mutated pHE7 vector [17] and solubilized and purified as previously described [15,16]. The pure octameric rHb were then obtained after size exclusion chromatography on column Superose 12 HR16/50 (GE Healthcare Lifesciences, Uppsala, Sweden) equilibrated with Ringer Buffer (Sigma-Aldrich, Saint-Louis, USA). Endotoxin Removal from octameric rHbβG83C solution was performed with Polymyxin B agarose columns (Detoxi-Gel Endotoxin Removing, Pierce, Rockford, USA) according to the manufacturer's instructions. Briefly, columns were washed with five column volumes of 1% sodium deoxycholate, followed by five column volumes of pyrogen-free water and five column volumes of Ringer Buffer. The octameric rHbβG83C was applied to a Detoxi-Gel column, incubated during 30 min at 4 °C, and then eluted with Ringer Buffer. Samples were concentrated by ultrafiltration (Amicon Ultra, Millipore, Billerica, USA). This procedure was repeated twice. Then, endotoxin content was determined by the endotoxin detection system based on Limulus Amebocyte Lysate test (LAL) (Lonza, Basel, Switzerland). The LAL assay was performed in duplicate using commercial LAL reagent. The assay was performed in pyrogen-free test tubes which 0.1 mL of octameric solution (concentration range from 1 μg/mL to 200 μg/mL of protein) and 0.1 mL of LAL reagent were added. After 1 h incubation at 37 °C, the test tubes were examined by 180° inversion for the presence of a stable solid clot. A clotted incubation mixture was considered to be a positive result. Endotoxin standard (E. coli strain O55: B5, 0.125 EU/mL) and pyrogen-free LAL reagent water, both provided by the manufacturer, were used as a control. A labeled LAL reagent sensitivity of 0.125 EU/mL was confirmed by serial dilutions prior to use. Two modified Hb solutions were used as reference: Dex-BTC-Hb obtained from Pasteur Mérieux Serums et Vaccins, France; Oxyglobin a glutaraldehyde-polymerized bovine Hb (Biopure Corporation, Cambridge, Massachusetts, USA), provided by Dr. Alayash (Center for Biologics Evaluation, Food and Drug Administration, Bethesda, MD, USA). All Hb solutions were diluted at 16 g/L in culture medium. This value corresponds to the plasmatic Hb concentration after a 1/5 volemic compensation of the total blood volume with a 80 g/L HBOC solution. This Hb concentration in the plasma simulates levels observed in clinical situations of hemodilution, such as cardiopulmonary bypass or resuscitation from hypovolemic shock. The control was Voluven 6% (w/v) (Fresenius Kabi, France) diluted at 1/5 in culture medium. Voluven is a 130 kDa hydroxyethyl starch (HES) exhibiting a physiological oncotic pressure close to HBOC studied. All solutions were incubated 16 h in contact with HUVEC at 37 °C, 5% CO2.
2.2 Cell culture and seeding
HUVEC were isolated from three different donors for each experiment according to the method of Jaffe et al. [28]. HUVEC were cultured at 37 °C, 5% CO2 in 25 cm2 tissue-culture-treated flask in complete medium. This medium consisted of an equal mixture of M199 and RPMI 1640, supplemented with 20% (v/v) pooled human serum, 2 mmol/L L-glutamine, 20 mmol/L HEPES, 100 IU/mL penicillin, 100 mg/mL streptomycin and 2.5 mg/mL Fungizon. HUVEC, at the first passage, were seeded in 24-wells plates at 25,000 cells/cm2 until 80% of confluence.
2.3 Measurement of Hb forms
The percentage of OxyHb, HbCO and MetHb, before and after experiments, were quantified spectrophotometrically between 450 and 700 nm at room temperature. Microsoft Excel solver was used to minimize the least squares differences between the experimental spectrum and a mixture of pure oxyHb, HbCO and metHb spectra. An analysis of the residuals indicated that no significant concentrations of other Hb derivatives were present to interfere with the quantification.
2.4 Cellular viability
In order to measure the mitochondrial activity of cells, Alamar Blue redox assay (Serotec Ltd., Kidlington, England) was used. After 16 h of incubation with rHbβG83C, the control HBOCs or Voluven, the medium was removed and the cells were washed with RPMI 1640 without phenol red (Sigma-Aldrich, Saint-Louis, USA). Then, Alamar Blue reagent dissolved at 10% (v/v) in RPMI 1640 without phenol red was added to each well. After 4 h incubation at 37 °C, the solution was removed and the color change, due to mitochondrial activity, was measured by monitoring the absorbance with a spectrophotometer (Beckman DU 640, Fullerton, CA, USA) at 570 and 630 nm. The optical density difference ΔOD = OD570 nm-OD630 nm was calculated in order to estimate the metabolic activity of the cells. Wells without cells were used as a reference. The results were normalized with respect to counted cells. The quantification of apoptosis/necrosis versus cell viability is based on the labeling and quantification of externalized phosphatidylserines (PS) by annexin V coupled with Alexa 488 and the nucleus with propidium iodide (PI) (Vybrant apoptosis kit #2, Invitrogen Life Technologies, Carlsbad, New Mexico, USA). PS has the ability to translocate on the external membrane layer of cell during early apoptosis. The Vybrant apoptosis kit has been developed for non-adherent cells, so manufacturer instructions have been adapted to our adherent cells. Indeed, several concentrations of trypsin (from 0.025 mg/mL to 0.25 mg/mL) were tested. We chose the concentration of 0.05 mg/mL inducing the less annexin V false positive cells with the shorter incubation time (3 min) (data not shown). After incubation with the different test solutions, cells were washed three times with phosphate buffered saline (PBS) and incubated with 2.5 μL of annexin V-Alexa 488 and 1 μL PI for 15 min at room temperature in the dark. Then cells were washed with PBS and harvested with 200 μL of 0.05 mg/mL trypsin-EDTA (Invitrogen Life Technologies) during 3 min at 37 °C. Cells were resuspended in fresh medium containing human serum (to inhibit trypsin) and centrifuged at 300 g during 5 min. The pellet was finally resuspended with 300 μL of annexin V buffer. Fluorescent intensities were measured with a Cytomics FC500 flow cytometer (Beckman Coulter).
2.5 Quantification of genes expression variation
Total RNA from HUVEC incubated with HBOCs, Voluven or culture medium were extracted using a technique, which by combining two widely used methods: the TRIzol reagent (Invitrogen Life Technologies) technique and the RNeasy minikit (Qiagen, Venlo, The Netherlands). Briefly, cells were washed with cold sterile PBS and incubated 5 min with 1 mL of TRIzol reagent allowing cell lysis. Cell lysate was then mixed with 200 μL chloroform and centrifuged at 12,000 g for 15 min. The upper phase containing RNA was transferred onto the column contained in the RNeasy mini kit. This technique provided a sufficient quantity of highly pure RNA. One μg total RNA was reverse transcribed into cDNA in a total volume of 20 μL using iScript cDNA Synthesis kit (Bio-Rad Life Science, Hercules, CA, USA) according to the manufacturer instructions.
Real-time quantitative PCR (qPCR) was performed on cDNA using the SYBR Green qPCR kit (Bio-Rad Life Science) in the iCycler real-time PCR detection system (Bio-Rad Life Science) according to the manufacturer instructions. Briefly, 5 μL of cDNA were mixed with 12.5 μL of 2X SYBR Green Mix and 300 nM of each primer in a final volume of 25 μL. RPS29 was used as an internal control to normalize gene interest amplification results. Samples were firstly subjected 3 min to 95 °C, then 25 cycles of 60 °C for 1 min and 95 °C for 10 s and finally, one cycle at 95 °C for 1 min and one cycle at 55 °C for 1 min. Gene expression analysis was performed using the gene expression analysis for iCycler iQ Real-Time PCR Detection System 1.0 (Bio-Rad Life Science).
2.6 Statistical analysis
Results were expressed as the mean ± standard errors of the mean (SEM) for each triplicate experiment (n = 3). One-factor ANOVA with PLSD Fisher correction (Statview IVs, Abacus Concepts, Inc., Berkeley, CA, USA) was used to analyze the data. P values ≤ 0.05 were considered as significant.
3 Results
3.1 Quantification of Hb forms
Because of their packaged manufacturing, the three different HBOCs were stored under different conditions i.e. Dex-BTC-Hb and Oxyglobin were formulated as oxyHb (90 to 80%, respectively) whereas rHbβG83C was a 92% HbCO form. Furthermore, Dex-BTC-Hb and rHbβG83C contain low levels of metHb (0–1%) whereas Oxyglobin levels of metHb were near to 20%. After 16 h of incubation in contact with HUVEC under air with 5% CO2 at 37 °C, size exclusion chromatography showed that rHbβG83C still octameric (data not shown) and, as expected, all HBOCs were significantly oxidized, rHbβG83C and Dex-BTC-Hb had the higher rates of oxidation into metHb compared to Oxyglobin. However, the final percentage of metHb with the rHbβG83C (69%) is close to that of Oxyglobin (62%), while Dex-BTC-Hb (41%) had a lower level of metHb as previously reported [26]. Furthermore, only 5.5% of the HbCO form still present in rHbβG83C at the end of the incubation.
3.2 Incidence of rHbβG83C on cellular viability
The mitochondrial activity of HUVEC in the presence of Voluven was the control considered for 100% activity (Fig. 2A). As expected, the incubation of HUVEC with 20% (v/v) of Oxyglobin or Dex-BTC-Hb for 16 h did not alter the reducing environment in the cells (Fig. 2A). The presence of rHbβG83 C has no incidence (87 ± 5%) compared to Voluven or both HBOC references.

Mitochondrial activity (A) and viability of human umbilical vein endothelial cells (B) after a 16 h incubation at 37 °C and 5% CO2 with Voluven (control), and different hemoglobin-based oxygen carriers human Hb conjugated to dextran benzene tetracarboxylate macromolecules, Oxyglobin, rHbβG83C. Mitochondrial activity was measured with Alamar Blue test (Serotec). Apoptosis was highlighted by the quantification of PS externalization labelled by annexin V, and necrosis by labelled nucleus with PI (Vybrant apoptosis kit # 2, Invitrogen Life Technologies). Cells were counted vs. fluorescence emission with a flow cytometer (Cytomics FC500, Beckman Coulter).
To go deeper in the cytocompatibility evaluation, apoptotic and necrotic cell amount was evaluated. The early apoptosis is characterized by the externalisation of the PS initially included in the inner leaflet membrane of the cells. Externalized PS was labelled with annexin V coupled with Alexa 488. Necrosis was highlighted by the incorporation of PI in the nucleus due to plasmic membrane permeabilization.
The viability of HUVEC incubated with Dex-BTC-Hb, Oxyglobin or rHbβG83C is 81%, 89% and 87%, respectively (Fig. 2B). The ratio of apoptotic cells is between 10% for Oxyglobin and 17% for Dex-BTC-Hb. Finally, the ratio of necrotic cells is between 0.3% for Oxyglobin and 2% for Dex-BTC-Hb. The difference observed for rHbβG83C is not statically significant.
3.3 Transcription of E-selectin and ICAM-1 genes in HUVEC after contact with rHbβG83C
As expected and after 16 h incubation, the E-selectin mRNA relative expression level was the lowest for HUVEC incubated with culture medium alone (Fig. 3A). Indeed, E-selectin expression is inducible and has a very weak basal expression. In the presence of Voluven, the transcription of E-selectin increased 2.9 fold, certainly due to the presence of the macromolecule [29]. After a 16 h incubation with Dex-BTC-Hb or Oxyglobin, HUVEC showed an induction of E-selectin transcription (around 1.4 and 0.9 fold, respectively) compared to HUVEC incubated with Voluven (Fig. 3A). Whereas, in the presence of rHbβG83C, the transcription level of E-selectin is close to that of the basal level represented by culture medium and weaker of 45% than observed with the other tested solutions.
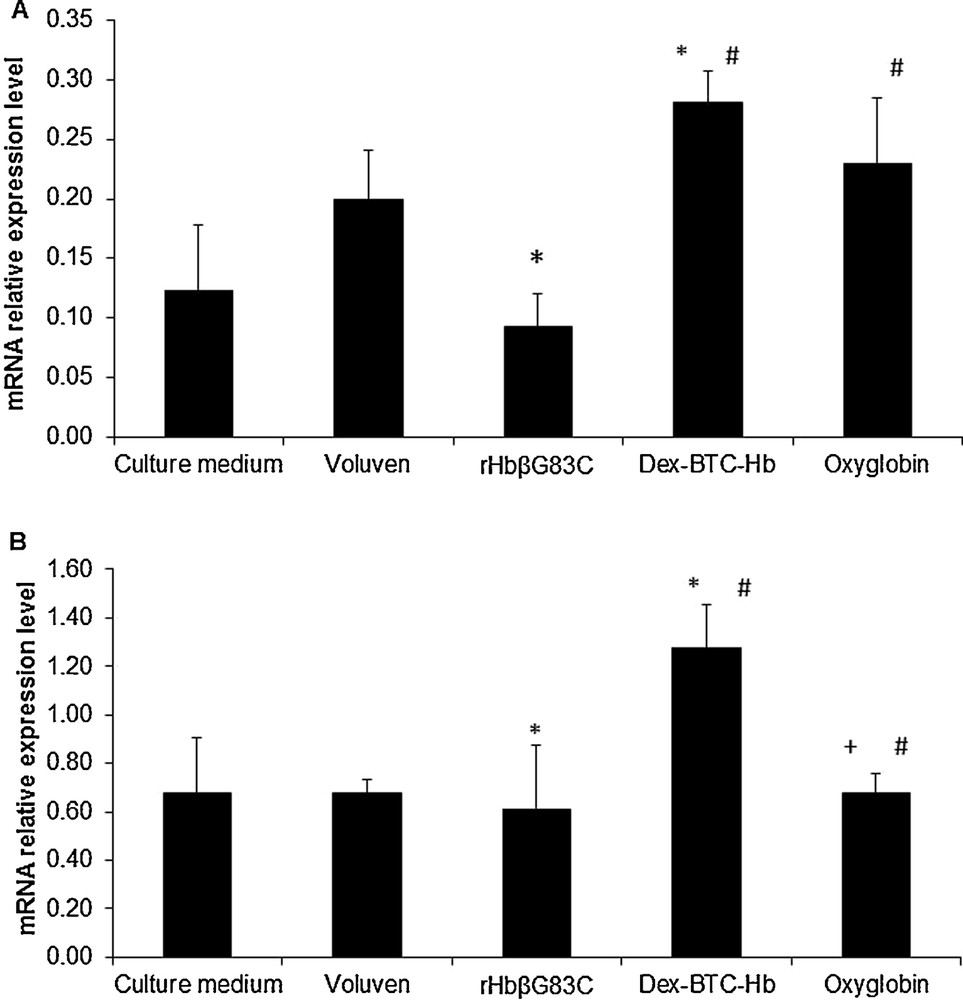
E-selectin (A) and ICAM-1 (B) mRNAs relative expression level in human umbilical vein endothelial cells after a 16 h incubation at 37 °C, 5% CO2. The cells were incubated with culture medium (Negative control), Voluven (volume expander, control), human Hb conjugated to dextran benzene tetracarboxylate macromolecules (Dex-BTC-Hb) and Oxyglobin (hemoglobin-based oxygen carriers references), and rHbβG83C. Results are expressed as mean ± standard errors of the mean. #P ≤ 0.05 vs. rHbβG83C, *P ≤ 0.05 vs. for Voluven, +P ≤ 0.05 vs. Dex-BTC-Hb vs. Voluven, +P ≤ 0.05 vs. Dex-BTC-Hb.
After 16 h incubation, the basal mRNA relative expression level of ICAM-1 was represented by culture medium condition (Fig. 3B). The transcription of ICAM-1 in HUVEC in the presence of Voluven or rHbβG83C led to a decrease by 35% or 50% respectively, compared to culture medium. As for E-selectin, the mRNA transcription level of ICAM-1 with rHbβG83C was 64% and 20% lower than observed with Dex-BTC-Hb and Oxyglobin, respectively.
Ultimately, rHbβG83C did not induce inflammation marker transcription compared to Dex-BTC-Hb or Oxyglobin. Furthermore, it had no impact on E-selectin transcription regarding the results obtained with culture medium.
3.4 Expression of HO-1 in HUVEC after contact with rHbβG83C
Culture medium represented the negative control without macromolecule and Voluven the macromolecule control without Hb. As expected, after 16 h at 37 °C in contact with HUVEC, the mRNA relative expression level of HO-1 is slightly increased by 2.9 fold (Fig. 4) by all three HBOCs solutions. The presence of Dex-BTC-Hb or Oxyglobin strongly induced (around 22 fold) the mRNA relative expression level of HO-1 compared to the basal level of HUVEC incubated with culture medium alone. The rHbβG83C induced 4.7 and 1.6 fold the transcription of HO-1 compared with HUVEC incubated with culture medium alone or Voluven, respectively. Thus, the induction of HO-1 transcription by rHbβG83C is 80% weaker than in the presence of Dex-BTC-Hb or Oxyglobin.
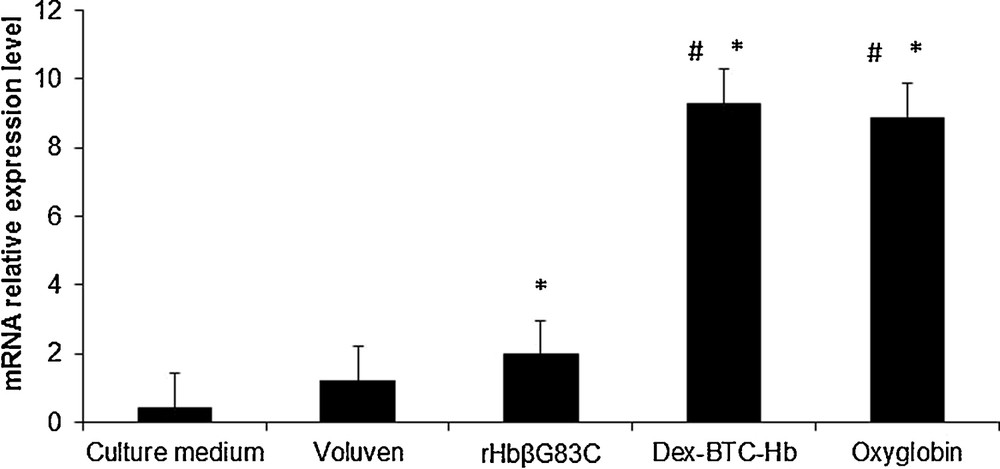
Heme oxygenase-1 mRNA relative expression level in human umbilical vein endothelial cells cultivated 16 h at 37 °C, 5% CO2 with culture medium (Negative control), Voluven (volume expander, control), human Hb conjugated to dextran benzene tetracarboxylate macromolecules and Oxyglobin (references hemoglobin-based oxygen carriers), and rHbβG83C. Results are expressed as mean ± standard errors of the mean. #P ≤ 0.05 vs. rHbβG83C, *P ≤ 0.05 vs. Voluven.
3.5 Repression of eNOS in HUVEC after contact with rHbβG83C
HUVEC incubated 16 h with culture medium represented the basal expression of the eNOS (Fig. 5). With Voluven, the transcription of eNOS was slightly increased by 1.4 fold. The incubation of HUVEC with Dex-BTC-Hb or Oxyglobin induced a marked transcription of eNOS around 1.7 fold compared to the incubation of HUVEC with culture medium (Fig. 5). In contrast, rHbβG83C induced a huge repression (around 11 fold) of eNOS compared to its basal level.

Endothelial nitric oxide synthase mRNA relative expression level in human umbilical vein endothelial cells cultivated 16 h at 37 °C, 5% CO2 with culture medium (Negative control), Voluven (volume expander, control), human Hb conjugated to dextran benzene tetracarboxylate macromolecules and Oxyglobin (references hemoglobin-based oxygen carriers), and rHbβG83C. Results are expressed as mean ± standard errors of the mean. #P ≤ 0.05 vs. rHbβG83C, *P ≤ 0.05 vs. Voluven.
4 Discussion
We have previously reported the functional and biochemical characteristics of rHbβG83C, a stable octameric recombinant Hb (129 kDa) (Fig. 1) as a HBOC candidate [15,17]. In this in vitro study, we evaluated the impact of this new oxygen carrier on endothelial cells compared to two other largely investigated HBOCs i.e. Dex-BTC-Hb (200 kDa) and Oxyglobin (100 kDa). These two HBOCs were chosen to investigate whether the modification of the Hb molecule, chemical or genetic, can influence the biological response of endothelial cells.
The two first parameters investigated were the mitochondrial activity and the viability of cells after incubation with the three HBOCs and Voluven, a volume expander (Fig. 2). Despite rHbβG83C and Oxyglobin presenting high levels of metHb after incubation with cells, no difference in the mitochondrial activity of cells nor viability (80% of viable cells, 15% of apoptotic cells and 5% of necrosis) was observed. These results indicate that, even at a high concentration, the metHb is not lethal for HUVEC and did not affect its mitochondrial activity. Regarding the auto-oxidation rate of Hb compared with their stored condition, there was no difference between Hb stored under CO form (rHbβG83C) or O2 form (Dex-BTC-Hb and Oxyglobin). This can be explained by the dilution of rHbβG83C in a medium oxygenated under 20% of O2. It can be postulated that after few minutes, all CO is replaced by O2. However, concerning metHb concentration, there were no antioxidant molecules in our culture medium so we cannot speculate here on the auto-oxidation of these HBOCs in the blood. However, in physiological conditions, even in acute hemodilution, the blood exhibited a capacity to reduce extracellular metHb within a day in vitro and in vivo [30,31]. Indeed, metHb is known to induce the expression of inflammatory mediators like E-selectin and ICAM-1 two inflammatory membrane proteins implicated respectively in the recruitment and the firm adhesion of leukocytes during their diapedesis through the endothelium [32]. However, despite rHbβG83C and Oxyglobin presented the highest levels of metHb after incubation with HUVEC, they did not induce a higher transcription of ICAM-1 and E-selectin compared to Voluven and Dex-BTC-Hb (Fig. 3). This suggested that the induction of inflammatory markers expression can be related to the modification of the macromolecule instead of its oxidation status. Indeed, the presence of Voluven, which does not contain oxidized Hb, induced an increase of E-selectin mRNA transcription compared to culture medium (3.1 fold). This was probably the result of the stress induced by the presence of an exogenous macromolecule. The behavior of E-selectin transcription seemed to be dependent on Hb modification for the different HBOCs solutions. Indeed, the three different HBOCs induced a different profile for E-selectin transcription. Dex-BTC-Hb and Oxyglobin induced an increase of mRNA relative expression level compared to HUVEC incubated with culture medium alone (around 3-4 fold). In contrast, the transcription of E-selectin in the presence of rHbβG83C was equivalent to culture medium, which is lower than Voluven. Furthermore, rHbβG83C seemed to be the HBOC solution with less impact on E-selectin transcription in HUVEC compared to Dex-BTC-Hb or Oxyglobin. rHbβG83C obtained by protein engineering has less impact on E-selectin transcription than chemically modified Hbs (Dex-BTC-Hb or Oxyglobin). So, we could speculate on the potential low impact of rHb βG83C on inflammation in terms of leukocyte recruitment. As we demonstrated in a previous work [23], the transcription level of E-selectin is differentially modulated depending on the type of HBOCs. E-selectin should be integrated in the systematic screening of HBOC impact on endothelial cells. ICAM-1 is the marker of inflammation the most studied concerning HBOC evaluation. In this study, we showed that HUVEC incubated with rHbβG83C have the lowest transcription level for ICAM-1 compared with the two other HBOCs solutions and Voluven. Indeed, the quantity of ICAM-1 mRNA in HUVEC incubated with Oxyglobin was equivalent to that incubated with Voluven, whereas Dex-BTC-Hb induced a huge induction of the transcription of ICAM-1. As for E-selectin, ICAM-1 transcription was lowered by the incubation with rHbβG83C compared with Voluven incubated HUVEC. For HUVEC, the presence of rHbβG83C in the culture medium for 16 h seemed to have no impact on inflammatory markers transcription compared to culture medium.
High concentrations of metHb are also known to induce the expression of the transcription of the gene coding for its detoxifier enzyme the heme oxygenase-1 (HO-1) [33]. So, considering the level of auto-oxidation of rHbβG83C and Oxyglobin, we could speculate that they will induce a high transcription of HO-1. Despite the finding that HO-1 transcription level in HUVEC was increased by the presence of HBOC compared to Voluven, Dex-BTC-Hb and Oxyglobin induced a higher increase of HO-1 transcription than rHbβG83C. Although Oxyglobin and rHbβG83C contained the same final percentage of metHb, a great difference in HO-1 transcription level was observed. As demonstrated in a previous study, the HO-1 transcription level is not only correlated with the percentage of metHb present in HBOC solutions but also with entities like the heme modified by oxidation correlated with oxidative reactions (auto-oxidation) [23].
The transcription of eNOS can be used as a marker of vasoactive effects of HBOCs on endothelial cells. Indeed, HBOCs are thought to induce vasoconstriction by reducing NO availability for smooth muscle cells’ relaxation. In our culture conditions, we supposed that a great increase of eNOS transcription could be a response to compensate for NO depletion. The mRNA relative expression level of eNOS was increased by the presence of Oxyglobin solution in the culture medium compared to Voluven. In contrast, the rHbβG83C seemed to repress the transcription of eNOS while the eNOS is constitutively expressed in EC. This result can be explained by a destabilization of the mRNA of eNOS or an inhibition of eNOS transcription. However, the destabilization of mRNA by Hb proteins had not been shown anywhere else. It has been highlighted that hydrogen peroxide (H2O2) was able to inhibit eNOS promoter activity leading to a diminution of eNOS transcription [34]. We have shown that the rate of rHbβG83C auto-oxidation after 16 h incubation with HUVEC at 37 °C was the highest (205 times) compared with Oxyglobin (69 times). So, we could speculate that the production of H2O2 will be higher in the presence of rHbβG83C. eNOS transcription repression will reduce the production of NO[35,36] by endothelial cells possibly inducing a vasoconstriction that can be potentialized also by the limited transcription of HO-1 producing CO, another vasodilator [37].
In parallel to our work, another recombinant octameric Hb has been obtained by introducing analogous substitution on the α-chains at αAsn78(EF7). This rHb αN78C also forms a stable octameric structure with a similar oxygen affinity as that of Hb A [18]. The mutation αN78C has been also associated with the αL29F or with αL29W mutation leading to an increase or decrease in oxygen affinity, respectively [38]. The group of Ho has shown that the administration of an octameric Hb (rHb αN78C, rHb αN78C/L29F or rHb αN78C/L29W) as resuscitation solution in mice after traumatic brain injury combined with hemorrhagic shock allows restoration of systemic hemodynamics (normalization of mean arterial blood pressure) and normalization of markers of global tissue metabolism, which are much better than lactated Ranger's solution [38]. The rHb αN78C/L29F with high oxygen affinity confers acute neuroprotection assessed at 24 h after injury [38].
5 Conclusion
In summary, rHbβG83C has no effect on cell viability with less impact on HO-1 transcription compared to the two other HBOCs solutions tested (Dex-BTC-Hb, Oxyglobin). Furthermore, the octameric rHbβG83C produced by protein engineering decreased the inflammatory effect of HBOC on endothelial cells (E-selectin and ICAM-1). Consequently, rHbβG83C, a genetically engineered octameric Hb, without any further chemical modification, may appear as an interesting molecule for HBOC development leading to little impact at the level of endothelial cell inflammatory response.
Disclosure of interest
The authors declare that they have no conflicts of interest concerning this article.
Acknowledgements
This work was supported by the Institut national de la santé et de la recherche médicale (INSERM), the University Paris XI, the EU Framework VI EuroBloodSubstitutes project and the région Lorraine.
We thank Dr M. Marden for a critical analysis of the manuscript. We thank Dr O. Barzu for providing the BLi5 strains of Escherichia coli BL21 (DE3) strain containing plasmid pDIAI7 vector (Institut Pasteur, Paris, France), Dr A. Alayash for providing the Oxyglobin and Dr P. Lacolley (Unité mixte UHP-INSERM U684, Faculté de médecine, Vandoeuvre-lès-Nancy, France) for the access to the qPCR system.