1 Introduction
The Diphyllobothriidea (Platyhelminthes: Eucestoda) is a rather small (50 valid species) group of the most basal ‘true’ bothriate tapeworms (Eucestoda) [1,2]. It is unique among cestodes in its wide spectrum of definitive hosts, which includes all major groups of tetrapods, i.e. amphibians, reptiles, birds and mammals, including humans. However, the knowledge of the biology, life cycles, host associations, ultrastructure and phylogenetic relationships of diphyllobothriideans is fragmentary, with most data available only on a very few species of medical (Diphyllobothrium, Diplogonoporus, Spirometra) and veterinary (Ligula, Schistocephalus) importance.
Kuchta et al. [3] erected the order Diphyllobothriidea, which previously was part of the Pseudophyllidea, and listed three families within this new order, namely Diphyllobothriidae Lühe, 1910 with species parasitizing birds and mammals, Cephalochlamydidae Yamaguti, 1959 from amphibians, and Scyphocephalidae Freze, 1974 from reptiles. Preliminary molecular data [4] inferred from sequences of complete small subunit and partial (D1–D3 region) large subunit nuclear rDNA of a rather limited dataset of taxa (eight species) indicated that the family Cephalochlamydidae represented by Cephalochlamys namaquensis (Cohn, 1906) from clawed frog [Xenopus laevis (Daidin)] is the most basal taxon, whereas Duthiersia fimbriata (Diesing, 1854) from the monitor Varanus exanthematicus (Bosc), both species occurring in Africa, is a sister taxon to the remaining diphyllobothriideans from birds and mammals with cosmopolitan distribution.
Prior to this study, no data on vitellogenesis, which is one of the key steps in the formation of female gametes in invertebrates (flatworms) with polylecithal eggs (Neoophora) [5,6], were available. Just recently, Yoneva et al. [7] studied the vitellogenesis of the broad fish tapeworm, Diphyllobothrium latum (Linnaeus, 1758), the most frequent causative agent of human diphyllobothriosis [8].
Among the basal bothriate eucestodes, vitellogenesis was studied in several species of the Caryophyllidea, but with most data limited to the parasites of cyprinids in the Palearctic Region (e.g. [9–11]), in three species of the relictual order Spathebothriidea [12,13] from freshwater and marine teleosts, in one species of the order Diphyllidea [14] and several species of the marine order Trypanorhyncha, parasites of elasmobranchs (e.g., [15]). Therefore, the ultrastructural data on vitellogenesis are available for all but one (Haplobothriidea) orders considered representing the most basal bothriate eucestode lineages. This made it possible to compare vitellogenesis in basal cestodes (see [7]) but the existing dataset is insufficient because the taxonomic diversity of most orders at the familial level has not been by far covered and representative taxa with different life cycle strategies from unrelated vertebrate hosts have never been studied.
Therefore, the aim of the present study was to examine the process of vitellogenesis of diphyllobothriidean cestodes across all three families representing different life cycle strategies (one vs. two intermediate hosts, aquatic vs. terrestrial) and different definitive host groups (amphibians, reptiles, birds and mammals), with an attempt to highlight the essential characters of vitellocytes during their formation and to relate these characters to distinct life strategies of the selected species studied.
2 Materials and methods
Adult specimens of C. namaquensis (Cephalochlamydidae) were collected by V. Matey from the intestine of the naturally infected African clawed frog X. laevis caught off Irvine ponds, Orange Country, California, USA and fixed in Karnovsky's fixative in the 0.1 M phosphate buffer (pH 7.2).
Adult tapeworms of Duthiersia expansa Perrier, 1873 (Scyphocephalidae) were collected by R. Kuchta and T. Scholz from water monitor V. salvator (Laurenti) in the West Mountains, Thang Hoa Province, Vietnam and fixed in cold (4 °C) 1.5% glutaraldehyde and 1.5% paraformaldehyde solutions in 0.1 M Hepes (pH 7.4).
Mature worms of Schistocephalus solidus (Müller, 1779) (Diphyllobothriidae) were obtained by in vitro cultivation system and fixed in cold (4 °C) 2.5% glutaraldehyde in phosphate-buffered saline (0.1 M) at pH 7.4; these specimens were provided by M. Kalbe.
After post-fixation, the material was dehydrated in a graded series of acetone, infiltrated and finally embedded in Spurr's and Epon epoxy resins. Resin blocks were sectioned via a diamond knife on a Leica Ultracut UCT ultramicrotome. Ultrathin sections (60–90 nm) were collected on copper grids and stained routinely with uranyl acetate and lead citrate according to Reynolds [16]. The samples were viewed using a JEOL 1010 Transmission Electron Microscope operating at 80 kV (Laboratory of Electron Microscopy, Institute of Parasitology, České Budějovice).
For detection of glycogen, gold grids containing ultrathin sections were treated first with 10% periodic acid for 30 min, and then processed with thiosemicarbazide for 24 h and 1% silver proteinate for 30 min [17]. The voucher specimens of the studied species are deposited in the collection of the Institute of Parasitology, Biology Centre of the Czech Academy of Sciences (IPCAS Nos. C-429, C-630 and C-674, respectively).
3 Results
The vitellarium of all three diphyllobothriideans (C. namaquensis, D. expansa and S. solidus) consists of numerous vitelline follicles distributed in the cortical parenchyma. Each vitelline follicle is composed of vitelline cells at various stages of maturity as well as of interstitial cells (Fig. 1A, D, G). The latter contains nuclei with scattered clumps of heterochromatin, mitochondria and electron-dense vesicles (Fig. 1B, F, I). Cytoplasmic projections of interstitial cells penetrate between vitellocytes. Occasionally, intercellular junctions occur between the interstitial and vitelline cells (Fig. 1C). The vitelline follicles are separated from the parenchyma by a basal lamina (Fig. 1A, E, H). Four stages in the development of the vitellocytes can be recognized: immature, early maturation stage, advanced maturation stage, and mature vitelline cells (Fig. 1A, D, G).
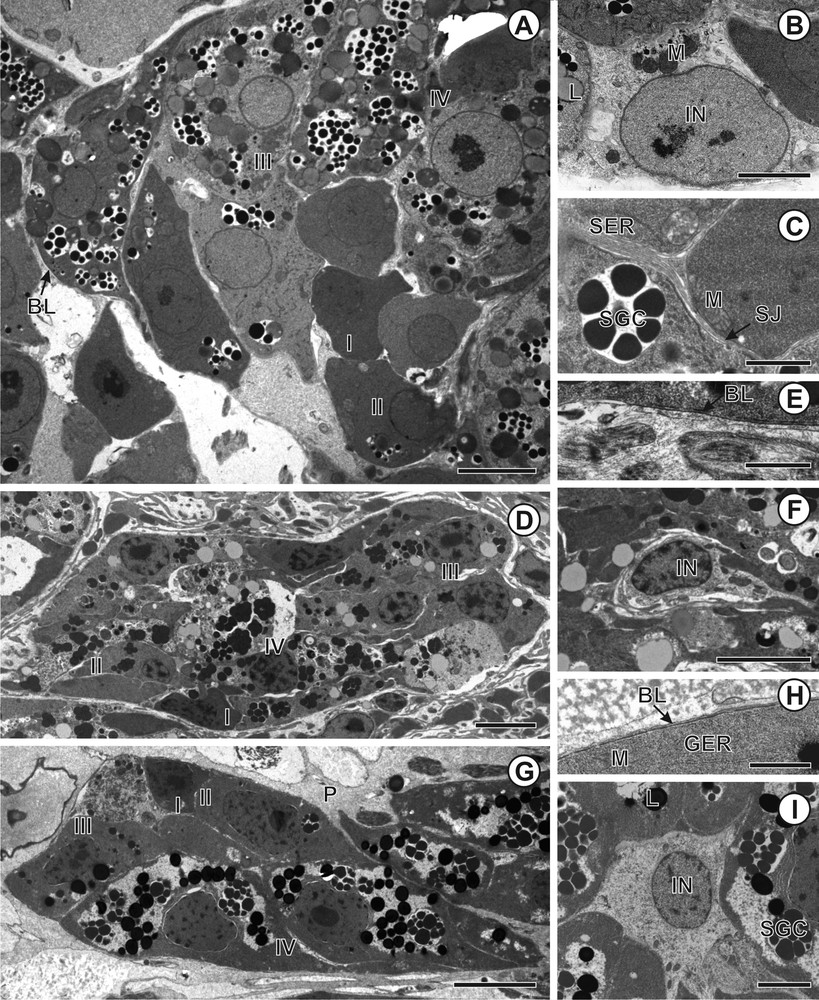
A–I. Transmission electron micrographs of vitelline follicles of Cephalochlamys namaquensis, Duthiersia expansa and Schistocephalus solidus. A. Vitelline follicle of C. namaquensis with vitellocytes at various stages of development. B. Nucleus of the interstitial cell of C. namaquensis. C. Septate junction between interstitial and vitelline cells in C. namaquensis. D. Vitelline follicle of D. expansa containing vitellocytes at various stages of maturation. E. Detail of basal lamina of D. expansa. F. Interstitial cell of D. expansa. G. Vitelline follicle of S. solidus containing vitellocytes at various stages of development. H. Detail of basal lamina, which surrounded externally vitelline follicle of S. solidus. I. Interstitial nucleus of S. solidus. Abbreviations to all figures: BL, basal lamina; Chr, chromatin; GC, Golgi complex; GER, granular endoplasmic reticulum; Gl, glycogen; IN, interstitial nucleus; L, lipid droplets; LB, lamellar body; M, mitochondria; N, nucleus; Nu, nucleolus; P, parenchyma; SER, smooth endoplasmic reticulum; SG, shell globules; SGC, shell globule clusters; SJ, septate junction; I, immature vitellocyte; II, early stage of vitellocyte maturation; III, advanced stage of vitellocyte maturation; IV, mature vitellocyte. Scale bars: A = 4 μm; B = 3 μm; C, H = 1 μm; D = 10 μm; E = 0.8 μm; F, G, I = 0.1 μm.
3.1 Immature vitellocytes
Immature vitellocytes (Figs. 1A, D, G, 2A, 3A, 4A) of all studied species are localized at the peripheral region of the vitelline follicle (Fig. 1A, D, G). They possess a large nucleus with irregular clumps of heterochromatin dispersed in the nucleoplasm and a thin layer of perinuclear cytoplasm (Figs. 2A, 3A, 4A). The nucleus of immature vitellocytes in C. namaquensis and S. solidus possesses distinct nucleolus (Figs. 2A, 4A). Free ribosomes and a few large mitochondria are scattered in the cytoplasm. Granular endoplasmic reticulum (GER) is visible at this stage in the cytoplasm of immature vitellocytes of C. namaquensis and D. expansa (Figs. 2A, 3A).

A–G. Transmission electron micrographs showing vitellogenesis in Cephalochlamys namaquensis. A. Immature vitellocyte. B. Early stage of maturation showing appearance of the first shell globules inside the Golgi vesicles. C. Advanced stage of maturation with shell globules, shell globule clusters and lipid droplets. D. Mature vitellocyte with loosely packed shell globules within the vitelline clusters. E. Golgi complex participates in formation of shell globule clusters. F. Mature vitellocyte with shell globule clusters and lipid droplets. G. Glycogen granules revealed by PA-TSC-SP cytochemical technique. Scale bars: A = 3 μm; B, C, D, F = 2 μm; E = 1 μm; G = 0.5 μm.
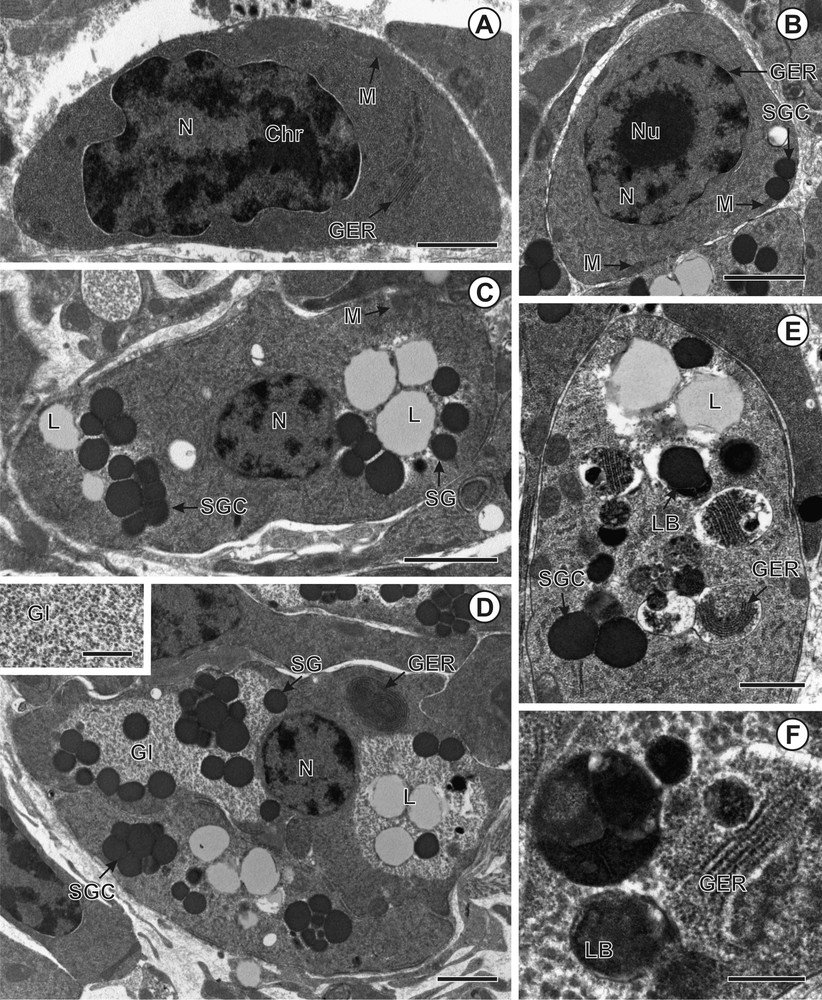
A–F. Transmission electron micrographs showing vitellogenesis in Duthiersia expansa. A. Immature vitellocyte containing nucleus, mitochondria and granular endoplasmic reticulum. B. Early stage of vitellocyte maturation with shell globule cluster composed of two shell globules. C. Advanced stage of vitellocyte maturation with shell globules, shell globule clusters and lipid droplets. D. Mature vitellocyte showing glycogen granules associated with vitelline clusters and lipid droplets. Inset: Glycogen granules revealed by PA-TSC-SP cytochemical technique. E. Mature vitellocyte with lamellar bodies and granular endoplasmic reticulum (GER). F. Details of lamellar bodies. Scale bars: A, D = 10 μm; B, F = 0.2 μm; C = 0.1 μm; E = 1 μm; Inset = 0.5 μm.

A–H. Transmission electron micrographs showing vitellogenesis in Schistocephalus solidus. A. Immature vitellocyte with high nucleo-cytoplasmic ratio. B. Early maturing vitellocyte showing nascent shell globules within the Golgi vesicles. C. Early stage of vitellocyte maturation showing the appearance of the first shell globule clusters. D. Advanced stage of vitellocyte maturation and mature vitellocyte with shell globule clusters, lipid droplets and glycogen granules. E. Mature vitellocyte. F. Glycogen granules revealed by PA-TSC-SP cytochemical technique. G and H. Details of lamellar bodies. Scale bars: A, B, F = 0.1 μm; C = 0.3 μm; D = 4 μm; E = 3 μm; G = 0.5 μm; H = 0.6 μm.
3.2 Early stage of differentiation
During this stage of development (Figs. 2B, 3B, 4B), the volume of perinuclear cytoplasm increases. The vitellocytes are characterized by a period of high secretory activity. Mitochondria, free ribosomes, developing granular endoplasmic reticulum (GER) and Golgi complexes are involved in the synthesis and packaging of vitelline material. The nucleus exhibits several irregular clumps of heterochromatin and nucleolus. The increased volume of perinuclear cytoplasm contains a few profiles of GER confined to the nuclear membrane and several mitochondria, mainly in the periphery of the cells (Figs. 2B, 3B, 4B). Preceding the formation of shell globule clusters, several individual shell globules inside the Golgi-derived vesicles are observed in the vitelline cytoplasm (Figs. 2B, 4B). Subsequently, the vesicles increase in size and aggregate, forming shell globule clusters composed of globules of various sizes. The early stage of vitellocyte differentiation is completed with the appearance of the first lipid droplet in vitelline cytoplasm (Fig. 2B).
3.3 Advanced stage of maturation
Advanced maturing vitellocytes (Figs. 2C, E, 3C, 4C, D) are elongated cells with the centrally situated nucleus and heterochromatin clumps usually adjacent to the membrane of the nucleus. During the advanced stage of maturation, protein synthesis still continues resulting in the formation of numerous shell globule clusters. Vitelline clusters of S. solidus (ca. 2.5 μm in diameter) and C. namaquensis (ca. 2 μm in diameter) comprise between 5 and 7 loosely packed globules (ca. 0.9 μm in diameter in S. solidus and 0.6 μm in C. namaquensis) embedded in electron-lucent matrix (Figs. 2C, 4D). In contrast, the clusters of D. expansa are bigger (ca. 5 μm in diameter), composed of ∼ 5 tightly packed electron-dense globules. Individual shell globules within the clusters of D. expansa are relatively homogeneous in shape and size (ca. 2 μm) and there is no outer limiting membrane surrounding the clusters (Fig. 3C). In addition, large electron-lucent lipid droplets are abundant in the cytoplasm of D. expansa and C. namaquensis (Figs. 3C, 4C, E). The cytoplasm of S. solidus contains glycogen granules situated around electron-dense lipid droplets (Fig. 4F).
3.4 Mature vitellocytes
The perinuclear cytoplasm of mature vitellocytes (Figs. 2D, F, G, 3D–F, 4D–H, 5A–D) is almost entirely occupied by four types of vitelline inclusions: shell globule clusters, lipid droplets, glycogen granules, and lamellar bodies. Concentric rows of granular endoplasmic reticulum (GER) are also found close to the cell periphery (Figs. 3D, 4E). The glycogen granules are scattered among lipid droplets and vitelline clusters (Figs. 2G, 3D, 4D). The test of Thiéry was used for revealing the presence of glycogen granules (Fig. 2G, 3D, Inset, Fig. 4F). Mature vitellocytes of D. expansa and S. solidus contain numerous lamellar bodies in the cytoplasm (Figs. 3E, F, 4E, G, H), but these cell structures are missing in C. namaquensis (Figs. 2D, F, 5A). Concentric rows of granular endoplasmic reticulum (GER) occur in the vicinity of the lamellar bodies (Figs. 3E, 4E), which may indicate that the appearance of these structures coincides with the breakdown of granular endoplasmic reticulum. For comparative purposes, a generalized scheme of mature vitellocytes of the three species is provided in Fig. 5(A–D). Table 1 summarizes general characters of the mature vitellocytes in the Diphyllobothriidea.
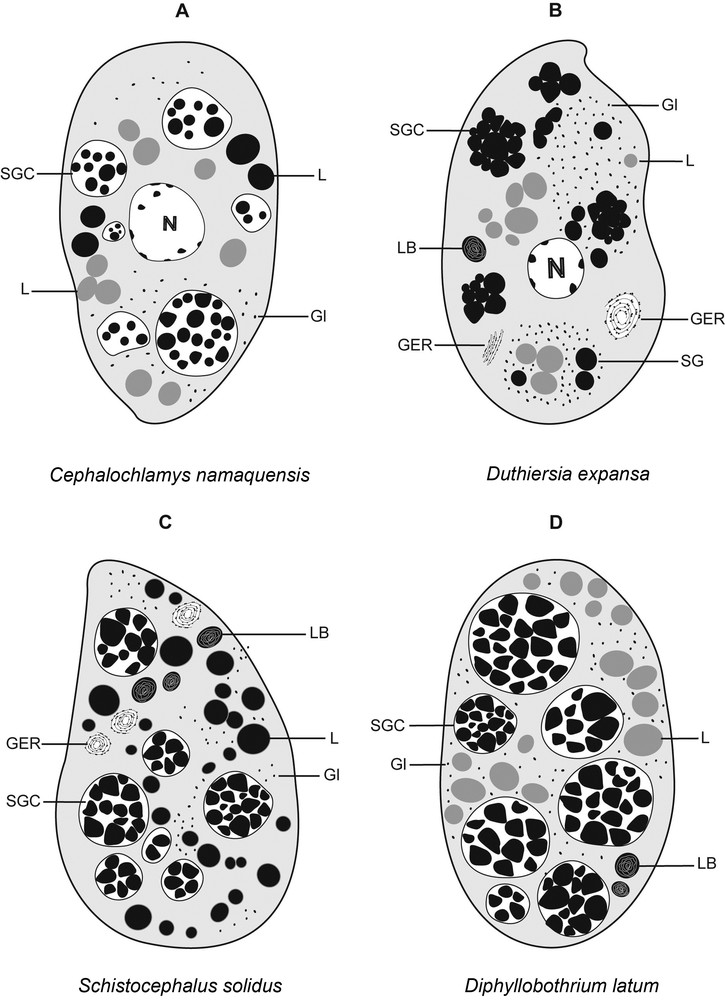
A–D. Diagrammatic representation of mature vitellocytes in four diphyllobothriidean cestodes (A) Cephalochlamys namaquensis, (B) Duthiersia expansa, (C) Schistocephalus solidus and (D) Diphyllobothrium latum.
Comparison of general characters of mature vitellocytes in the Diphyllobothriidea.
SG | SGC | L | Gl | LB | References | |
Order Diphyllobothriidea | ||||||
Family Cephalochlamydidae | ||||||
Cephalochlamys namaquensis | Up to 35 0.6 μm |
Loosely packed Membrane bound 2 μm |
S U |
Cytoplasmic | – | Present study |
Family Scyphocephalidae | ||||||
Duthiersia expansa | Up to 10 2 μm |
Tightly packed Non-membrane bound 5 μm |
S | Cytoplasmic | + | Present study |
Family Diphyllobothriidae | ||||||
Diphyllobothrium latum | Up to 35 0.4–0.9 μm |
Loosely packed Membrane bound 3 μm |
S | Cytoplasmic | + | [7] |
Schistocephalus solidus | Up to 10 0.9 μm |
Loosely packed Membrane bound 2.5 μm |
U | Cytoplasmic | + | Present study |
4 Discussion
Vitellocytes are important cells of the female reproductive system of the neoophoran Platyhelminthes in relation to the process of egg-shell formation and nourishment of the embryo [6]. General pattern of vitellogenesis of the diphyllobothriidean cestodes [7,present study] proceeds in a way commonly found in representatives of other lower bothriate eucestodes, i.e. Caryophyllidea, Spathebothriidea, Diphyllidea, and Trypanorhyncha [9–15,18–23].
Remarkable variations were detected among cytoplasmic inclusions of vitellocytes of the four diphyllobothriidean taxa studied until now, i.e. Diphyllobothrium latum from a mammalian host and three species studied in the present study, with regard to (1) the structure of shell globule clusters (loosely packed shell globules in C. namaquensis, D. latum and S. solidus vs. tightly packed globules in D. expansa); (2) the presence of (i) electron-lucent saturated lipid droplets in D. latum and D. expansa, (ii) electron-dense unsaturated lipid droplets in S. solidus, and (iii) both types of lipid droplets in C. namaquensis; (3) the presence of lamellar bodies in D. latum, D. expansa, and S. solidus vs. their absence in C. namaquensis.
Yoneva et al. [7] compared the arrangement of shell globule clusters, chemical nature of lipid droplets, glycogen granules and lamellar bodies in cestodes belonging to different orders. Therefore, the degree of variations of vitelline inclusions in the members of all three families of the same order is discussed in detail below.
4.1 Shell globules and shell globule clusters
Of particular interest are the morphological differences of vitelline clusters among the four diphyllobothriidean species studied to date [7,present study]. Vitelline clusters among them differ from each other by the degree of packaging shell globules, the number of shell globules in each cluster, and their size. Shell globule clusters in vitellocytes of S. solidus show a basic ultrastructural organization similar to that reported in D. latum [see 7], both cestodes belonging to the same family and parasitizing homeiothermic hosts. However, the clusters between the two species differ by the number of individual shell globules. The number of globules in clusters of D. latum is almost three times greater than in S. solidus. Surprisingly, C. namaquensis and D. latum share some common features, even though they are biologically and phylogenetically very distant [3,4]. Both exhibit vitelline clusters of approximately the same number of globules and possess individual globules of about the same diameter. Comparing the clusters of D. expansa with the three other species, we found similarities with S. solidus in the number of shell globules in each cluster, although they present different arrangement of shell globules in clusters (Fig. 5). These two taxa may represent phylogenetically closely related groups (R. Kuchta, unpublished data).
4.2 Lipids
The presence of lipid droplets in C. namaquensis, D. expansa, S. solidus and D. latum [7,present study] is consistent with the previous observation in other cestode groups, including the most basal eucestodes. These droplets probably play an important role in supplying nutritive reserves for the developing embryo [6]. In the Diphyllobothriidea, the type and amount of lipids varied across the examined species/families. S. solidus possesses osmiophilic and electron-dense lipid droplets containing a high level of unsaturated fatty acids, whereas lipid droplets in D. expansa are osmiophobic and electron-lucent, thus representing saturated fatty acids. It is interesting to note that the same type of lipids represent the most abundant inclusion in mature vitellocytes of the diphyllobothriid D. latum, but are less numerous in D. expansa than in D. latum (see [7]). In the presumably most basal taxon studied, C. namaquensis, vitelline cells differ in several ultrastructural aspects from those of the other diphyllobothriideans (Fig. 5). One of the characteristic features of mature vitellocytes of C. namaquensis is the presence of both types of lipids; the same character was observed in one of the three spathebothriidean cestodes studied by Bruňanská et al. [12], Cyathocephalus truncatus, a parasite of freshwater teleosts in the Holarctic Region.
4.3 Glycogen
Glycogen is the most common vitelline component in the Neodermata as an important source of energy for the future embryo [6]. The glycogen granules usually appear in the final stage of vitellogenesis and occupy almost entirely the cytoplasm of mature vitellocytes. All members of the Diphyllobothriidea contain numerous glycogen granules, usually accumulated around the lipid droplets and shell globule clusters.
4.4 Lamellar bodies
Another type of subcellular structures worth discussing, lamellar bodies, were found in mature vitellocytes of three of the four diphyllobothriidean cestodes studied. They appear during the final stage of vitellocyte maturation usually close to concentric rows of granular endoplasmic reticulum (GER) and vitelline clusters. Lamellar bodies are present in mature vitellocytes of S. solidus, D. expansa and D. latum, but they have not been observed in C. namaquensis, i.e. the most basal taxon of the order. Structures with lamellar arrangement are particularly widespread in caryophyllideans and spathebothriideans, i.e. the most basal eucestodes [7,10–13,19,20,24,present study] as well as in some trematodes and monogeneans [25–32].
The formation of lamellar bodies is associated either with the breakdown of granular endoplasmic reticulum [24,29], with the transformation of shell globule clusters during the process of egg formation [13], or with both processes [20]. Based on the present data and on those by Yoneva et al. [7], lamellar bodies in diphyllobothriideans seem to originate from both the granular endoplasmic reticulum and/or by transformation of vitelline clusters. Two distinct types of lamellar bodies were detected in the Caryophyllidea. The first type, referred to as regular lamellar bodies, shows distinct concentric features, and the second type, irregular lamellar bodies, appears to have a non-concentric configuration, as has been observed in D. latum, D. expansa, and S. solidus [7,present study]. The same type is commonly found in spathebothriideans [12,13]. In contrast, caryophyllideans appear to have both types of these lamellar structures, with the irregular type being more common than the regular one [10,11,19,20,24]. However, the function of these bodies in parasitic flatworms including cestodes is unknown.
4.5 Possible evolutionary implications – Diphyllobothriidea
Some previous studies on vitellogenesis in free-living and parasitic platyhelminths indicated that the ultrastructure of some vitelline cytoplasmic inclusions might differ, even between genera [10,13,33]. In the present study, the process of formation of vitelline cells in representative species of all diphyllobothriidean could be compared and related to their presumed phylogenetic position within the order Diphyllobothriidea. Based on molecular data published by Brabec et al. [4], the most plesiomorphic features would be expected to exist in C. namaquensis, whereas the diphyllobothriids S. solidus and D. latum should rather possess more derived characters; the reptilian parasite D. fimbriata should exhibit intermediate features.
As described above, this assumption is partly supported by the absence of lamellar bodies in C. namaquensis (present in the three more derived taxa) and by the presence of two types of lipid droplets in the former species (in contrast, the derived taxa of the Scyphocephalidae and Diphyllobothriidae possess only one of these types of droplets). Variations in the quantity of lipids in cestodes are supposed to be generally host-related [34]. Thus, such variations in diphyllobothriideans could be related to three different host groups, as described for bothriocephalideans [34]. In addition, the degree of variations in the vitelline inclusions of members of all three families of the order likely depends on their specific life cycles, which include different definitive hosts such as birds and mammals (Diphyllobothriidae), amphibians (Cephalochlamydidae), and reptiles (Scyphocephalidae), and the number of intermediate hosts (one vs. two) or type of egg development (presence or absence of an operculum). Even though data on vitellogenesis of representatives maturing in all major vertebrate groups are now available, it is obvious that more species should be studied, such as representatives of diphyllobothriids from marine hosts and other taxa from reptiles, before a more complex view of the possible evolution of vitellogenesis is presented.
4.6 Possible evolutionary implications – basal Eucestoda
The relationships of the Diphyllobothriidea with other basal groups of the Eucestoda are unclear and analyses inferred from different molecular markers provided different topologies of the phylogenetical tree of the Eucestoda. A close relationship with the Spathebothriidea and all cestode orders from elasmobranchs and remaining acetabulate groups was indicated by the sequences of mitochondrial genomes [1]. A most recent phylogenetic study [2] even placed the Diphyllobothriidea as a sister taxon of the Litobothriidea and all acetabulate (“tetrafossate”) orders, closely related to the Trypanorhyncha.
The ultrastructure of vitellocytes of the spathebothriideans, parasites of freshwater and marine fish that possess operculate eggs, but differ from each other in the presence/absence of polar filaments [35], resemble those in the diphyllobothriideans, which could indicate relatedness of both orders, thus supporting the topology of the cestode tree presented by Waeschenbach et al. [1]. Interestingly, the eggs of the diphyllobothriids (including D. latum, S. solidus and D. expansa) are hard-shelled, operculate and mostly unembryonated when laid. The only exceptions are eggs of the cephalochlamydids that have a membranous shell, are unoperculate, but are embryonated when laid, and whose coracidium is liberated by rupture of the egg membrane [36].
The scarcity of ultrastructural data throughout all major cestode groups at least to the family level limits a more detailed comparison of vitellogenesis in the Cestoda. It is thus impossible to map the ultrastructural characters on the phylogenetic tree of major groups of tapeworms, the phylogenetic relationship of which are not yet well resolved [1,2]. The future ultrastructural research thus should be much more selective, as far as the choice of the species to be studied: instead of incidental selection of taxa based just on their availability, more effort should be given to a more complete taxonomic coverage, i.e. the search for representatives of as many families or even genera as possible, with the focus on phylogenetically important groups, such as the most basal cestodes (“Cestodaria”, i.e. Gyrocotylidea and Amphilinidea), Haplobothriidea, and Litobothriidea.
Acknowledgements
The authors thank Martin Kalbe from Max Planck Institute (Germany), Céline Levron (France), and Victoria Matey from the Center for Inland Waters and Department of Biology (USA), who kindly provided samples of Schistocephalus solidus and Cephalochlamys namaquensis, respectively, Tran Thin Binh, Institute of Ecology and Biological Resources, Vietnamese Academy of Sciences, Hanoi, Vietnam, for help during the collecting trip to Vietnam during October, 2010, and the staff of the Laboratory of Electron Microscopy (head Jana Nebesářová), Institute of Parasitology, České Budějovice, for technical assistance. This work was funded by the Grant Agency of the Czech Republic (project No. P506/12/1632), the Institute of Parasitology (RVO: 60077344), and the Grant Agency of the Slovak Republic VEGA (project No. 2/0047/11).