1 Introduction
The Harderian gland (HG) is an orbital gland found in the majority of land vertebrates [1–4]. Although the function of this gland is still unclear, its size and widespread occurrence among vertebrates suggest that it has potentially important more roles.
One of the main characteristics of this gland is the extreme variety, not only of its morphology at the ultrastructural level, but also its biochemical properties being the secretory material specific for each species, genus, or order. This most likely reflects a versatility of functions related to different adaptations of the species considered.
The rat HG produces large amounts of lipids and porphyrins, which are secreted into the conjunctival sac. The lipids are represented by fatty acids with chain lengths from 12 to 22 carbon atoms and fatty alcohols derived from wax esters, and cholesterol [5–7]. The function of the lipid secretions is not well established; however, roles in the protection of the cornea and lubrication of the nictitating membrane have been suggested [5–7]. Moreover, other functions have been hypothesized, such as an involvement in thermoregulation [8,9], in a bactericidal effect, and as a solvent for bioactive substances as well as pheromones [6,10,11]. The production of porphyrins in the HG suggests that these molecules are phototransducers with an ability to absorb UV light [12,13]. Finally, due to the production of melatonin, an involvement of the HG in the retinal-pineal axis has been proposed [14–18].
Furthermore, the complexity of this gland is evident in its control by many exogenous (light and temperature) and endogenous factors (such as steroid hormones). Androgen and estrogen receptors occur in the HGs of rat and golden hamster [19,20]. The androgen receptor in the hamster HG binds 5α-dihydrotestosterone with a higher affinity than testosterone [19,21], suggesting that 5α-dihydrotestosterone has a greater effect on the secretory activity of the HG and on sexual dimorphism than testosterone [22,23]. Further, a correlation between estrogens and HG porphyrin content has been documented in rodents [24].
Although the steroid hormones are different, they are synthesized from a common precursor substrate, which is cholesterol. Steroidogenesis thus begins with the transport of cholesterol across the mitochondrial membrane and is mediated by the Steroidogenic Acute Regulatory (StAR) protein, considered to be the rate-limiting step in steroid hormone biosynthesis [25,26]. Subsequently, cholesterol is converted to pregnenolone by cytochrome P450 side chain cleavage (P450scc, the product of Cyp11a1 gene); pregnenolone diffuses into the cytosol and is converted in the smooth endoplasmic reticulum to progesterone and then androstenedione by 3β-hydroxysteroid dehydrogenase (3β-HSD, the product of Hsd3b1gene). Then, 17β-hydroxysteroid dehydrogenase (17β-HSD, the product of the Hsd17b3 gene) catalyzes the conversion of androstenedione to testosterone, which is the main final product of the steroidogenic process. Testosterone can thus be converted into either a more potent androgen, the 5α-dihydrotestosterone by 5α-reductase (5α-Red, the product of the Srd5a gene) or into 17β-estradiol by cytochrome P450 aromatase (P450 aro, the product of the Cyp19a1gene). Steroidogenic activity in the HG has not been documented to date, although studies have demonstrated the occurrence of P450scc and 5α-Red expressions in the hamster HG [27,28].
In this study, we analyze the expressions of the StAR protein and key steroidogenic enzymes in the HGs of male and female Rattus norvegicus. The objective of the present investigation was to ascertain (a) whether the rat HG is involved in steroid production starting with cholesterol, and (b) whether the pattern of gene and protein expressions displays sexual dimorphism.
2 Materials and methods
2.1 Animals
Male and female Wistar rats, Rattus norvegicus, weighing 300–350 g, were kept under regulated conditions of temperature (24 ± 2 °C) and light (12 h light/12 h dark cycles). The females were randomly selected during the reproductive cycle. They received commercial food pellets and water ad libitum. Rats were first anesthetized by an i.p. injection of chloral hydrate (40 mg/g body mass), and then decapitated. The HGs as well as testes and ovaries (positive controls) were dissected out and rapidly immersed in liquid nitrogen for biochemical and molecular determinations, as described below. Pieces of glands were quickly immersed in fixative for light or electron microscopy. The experimental protocols below described, as well as the housing conditions, was in accordance with the Italian guidelines (D.Lvo 116/92) and authorized by the local Animal Care Committee (ASL 44, Prot. Vet. 22/95). All efforts were made to reduce animal suffering and the number of animals.
2.2 RNA isolation and RT-PCR
Total RNA was extracted from the rat HG and gonads (100 mg from each animal), using TRIzol standard protocol (Invitrogen Life Technologies) and then treated for 30 min at 37 °C with DNase I (10 U/sample) (Amersham Bioscience) to eliminate any contaminations of genomic DNA. Total RNA purity and integrity were determined by spectrophotometry at 260/280 nm and electrophoresis on a denaturizing formaldehyde agarose gel [29]. One microgram of total RNA was reverse-transcribed using the SuperScriptTM First-Strand Synthesis System kit (Invitrogen Life Technologies). Specific primer sets were designed for RT-PCR using Primer 3 (http://frodo.wi.mit.edu/primer3). The sequences of the primers used were reported in Table 1.
Primers for RT-PCR.
Gene | Forward primer (5′ to 3′) | Reverse primer (5′ to 3′) | GeneBank Accession No. |
Star | agctccaaatgccactacct | tggccttttacagaggagca | AF044081.1 |
Cypllal | accccatctctgtgaccttg | tcgacccatggcatagctag | J05156.1 |
Hsd3bl | tttgtgggccagaggatcat | gtcaccttggcctttgtctg | NM_00100719.3 |
Hsdl7b3 | cacatggaatcaaggcggag | ctatacagaggccagggacg | NM_054007 |
Srd5al | cacctttgtcttggccttcc | tcaggaaacagggatgggtc | NM_017070.3 |
Srd5a2 | tcacaagagggaggcctttc | tctgcgagtaaaccaggta | NM_022711.4 |
Cypl9al | gctcccaggagtgtagcttg | ccacaccacacacagagtcc | NM_017085.2 |
β-Actin | agccatgtacgta gccatcc | ccctcatagatgggcacag | NM 031144.3 |
As internal control, the same cDNAs were amplified using β-actin oligonucleotide primers (Table 1). For the actual analysis, samples were heated for 3 min at 94 °C. Then 35 cycles were carried out, each consisting of 45 s at 94 °C, 45 s at 55–58 °C (55 °C for Hsd3b1; 56 °C for Cyp11a1; 57 °C for Star, Hsd17b1, Srd5a1 and Srd5a2; 58 °C for Cyp19a1), and 1 min at 72 °C. This was followed by a final 7-min extension at 72 °C. Finally, the PCR reaction products, separated on a 1.5% agarose gel containing ethidium bromide, were rapidly visualized. The amount of mRNA levels was quantified by ImageJ software.
2.3 Preparation of mitochondrial fraction and Western blot analysis
Mitochondria were isolated after homogenization of HGs, testes and ovaries (300 mg from each animal) in an isolation medium consisting of 220 mM mannitol, 70 mM sucrose, 20 mM Tris–HCl, 1 mM EDTA, 5 mM EGTA, and 5 mM MgCl2, pH 7.4, supplemented with the following protease inhibitors: 1 mM benzamidine, 4 mg/ml aprotinin, 1 mg/ml pepstatin, 2 mg/ml leupeptin, 5 mg/ml bestatin, 50 mg/ml N-tosyl-l-phenylalaninechloromethyl ketone, and 0.1 mM phenylmethylsulfonylfluoride. After homogenization, the samples were centrifuged at 700 g for 10 min. The supernatant was collected and transferred into new tubes for subsequent centrifugation at 10,000 g for 10 min. The obtained mitochondrial pellet was washed twice, then resuspended in a minimal volume of isolation medium, and kept on ice. Protein concentrations of mitochondrial fraction were estimated using a modified Bradford assay (Bio-Rad, Melville, NY, USA) [30–32]. The protein extracts were boiled in Laemmli sample buffer, separated by sodium dodecyl sulphate–polyacrylamide gel electrophoresis (SDS–PAGE) and transferred onto a nitrocellulose membrane. The complete transfer was assessed using prestained protein standards (Bio-Rad, Melville, NY). Membranes were first treated for 1 h with a blocking solution (5% non-fat powdered milk in 25 mMTris, pH 7.4; 200 mMNaCl; 0.5% TritonX-100, TBS/Tween) and then incubated overnight at 4 °C with polyclonal rabbit anti-StAR antibody (diluted 1:1000) (#EAP0193, Elabscience) or with polyclonal mouse anti-β-actin antibody (#sc-81178; Santa Cruz Biotechnology) (diluted 1:2000). After washing with TBS/Tween, the membranes were incubated with the horseradish-peroxidase-conjugated secondary antibody for 1 h at room temperature. The reactions were detected using an enhanced chemiluminescence (ECL) system (Amersham Bioscience, UK). Bands were quantified by ImageJ software.
2.4 Ultrastructure
For electron microscopy, pieces of HG (3 mm3) were promptly immersed and left for 3 h in Karnovsky's fixative in cachodylate buffer (pH 7.4), then postfixed for 2 h in cachodylate buffer containing 1% osmium tetroxide. The samples were dehydrated through a graded ethanol series, and finally embedded in Epon 812 [33,34]. Ultrathin sections stained with 4% uranyl acetate, followed by 1% lead citrate were examined using a Zeiss LEO-912 transmission electron microscope (Zeiss, Germany).
2.5 Immunohistochemistry
For localization of StAR, pieces of HGs were rapidly immersed in Bouin's fluid. Paraffin sections (5 μm thick) were incubated overnight with rabbit anti-StAR polyclonal antibody (diluted 1:50, in 0.1 m PBS at pH 7.4 with 0.1% BSA) (#EAP0193, Elabscience). After washing in PBS, the sections were incubated for 1 h with biotinylated goat antirabbit IgG (Dako) diluted 1:200, followed by incubation for 1 h with peroxidase-conjugated streptavidin (Vector) diluted 1:500. The antigens were visualized using 3,3′-diaminobenzidine tetrahydrochloride (Sigma–Aldrich Corp.) and 0.3% H2O2 Tris buffer (0.05 M, pH 7.6) [35]. For the negative controls, the primary antibody was omitted.
2.6 5α reductase and cytochrome P450 aromatase activities
5α-Red as well as P450 aro enzyme activities were measured by evaluating the in vitro conversion rate of testosterone to 5α-dihydrotestosterone and 17β-estradiol, respectively.
HG and testis (positive control) were homogenized 1:10 in Tris–HCl 0.05 M pH 7.4. After centrifugation at 3000 g for 10 min, 2 ml of supernatant were separately incubated with 2 μM or 18 μM of testosterone as substrate and incubate at 37 °C under shaking [36]. After 5 min, 1 ml of the assay mixture was taken from each sample and mixed with 9 ml of ethylic ether. Then, the samples were centrifuged at 13,000 rpm and the supernatants were dried in appropriate glass Petri dishes at 40–50 °C under shacking under air. The residues were mixed with 0.2 ml of 10 mg/ml bovine serum albumin (BSA) in PBS in order to dissolve the hormones from the Petri dish and then used for the determination of 5α-dihydrotestosterone (Abnova) and 17β-estradiol (Diametra) using the ELISA method [37–39]. The sensitivities were 6 pg/ml for 5α-dihydrotestosterone and 15 pg/ml for 17β-estradiol. The results are expressed as enzymatic units/mg tissue, one unit being defined as the amount of the enzyme that was capable of converting 1.0 nmol of testosterone to 5α-dihydrotestosterone or 17β-estradiol under the assay conditions above.
2.7 Statistical analysis
ANOVA followed by a Student–Newman–Keuls’ test was used to evaluate significant changes between groups. Differences were considered statistically significant at P < 0.05. All data were expressed as the mean ± S.D.
3 Results
3.1 Expression and immunolocalization of steroidogenic acute regulatory protein
Both mRNA and protein for StAR were expressed in the HG of both male and female rats, with significant higher levels in males than in females (Fig. 1A and B).
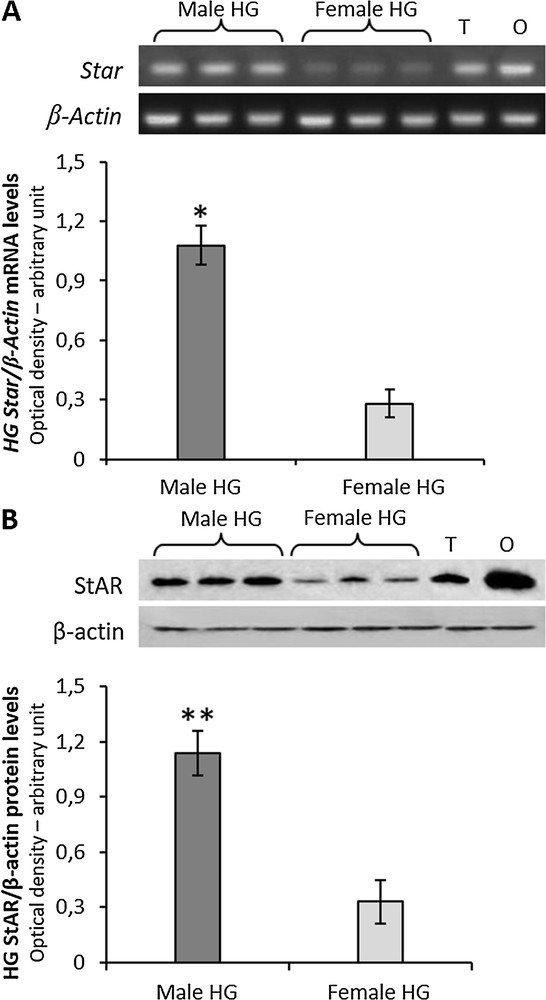
A, RT-PCR-analysis of Star in HG from three male and three female rats. The upper panel shows the mRNA levels normalized with respect to β-actin mRNA levels; lower panel, the levels of HG Star was quantified using the ImageJ program and normalized with respect to β-actin mRNA. T, testis; O, ovary: positive controls. B, the upper panel shows Western blot detection of the StAR protein in the HG from three male and three female rats. One specific band, corresponding to the size of 32 KDa was detected; lower panel, the levels of HG the StAR protein were quantified using the ImageJ program and normalized with respect to β-actin protein. T, testis; O, ovary: positive controls. Values shown represent the means ± S.D. of three animals. *P < 0.05; **P < 0.01. Masquer
A, RT-PCR-analysis of Star in HG from three male and three female rats. The upper panel shows the mRNA levels normalized with respect to β-actin mRNA levels; lower panel, the levels of HG Star was quantified using the ... Lire la suite
As reported by Chieffi Baccari et al. [40], two cell types are present in rat HG epithelium (Fig. 2A). Type-A cells showed the cytoplasm filled with large secretory vacuoles, whereas type-B cells contained numerous mitochondria and small unstained vacuoles (Fig. 2A). Immunohistochemical analysis revealed that the StAR protein was detected in the cytoplasm of both type A and type B cells, with a more intense immunopositivity in B cells (Fig. 2B).
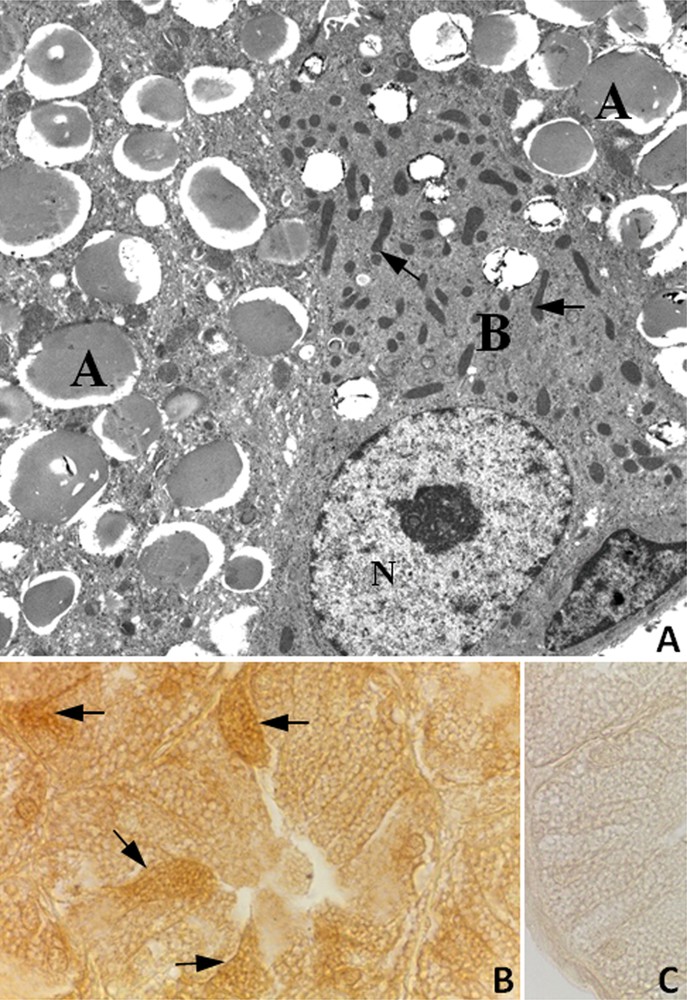
A, Electron micrograph of the HG from male rat. Large secretory vacuoles occupy the cytoplasm of type A cells (A); type B cell (B) displays numerous mitochondria (arrows) and small secretory vacuoles. N, nucleus. ×4600; B, Immunohistochemistry for the StAR protein in the HG from male rat. Type B cells showing a strong immunopositivity (arrows) were observed among A cells. X1000; C, StAR antibody-negative control section of HG.
3.2 Gene expression of steroidogenic enzymes
The mRNAs for Cyp11a1, Hsd3b1, Hsd17b3, Srd5a1, Srd5a2 and Cyp19a1 were detectable in the HG of both male and female rats (Fig. 3). Significant differences were observed between sexes in gene expression levels of Hsd17b3, Srd5a1 and Cyp19a1 (Fig. 3). Particularly, Hsd17b3 and Cyp19a1 mRNA levels were higher in males than females, whereas Srd5a1 mRNA levels were higher in females than in males (Fig. 3). No significant differences were observed in mRNA levels of Cyp11a, Hsd3b1and Srd5a2 between sexes.
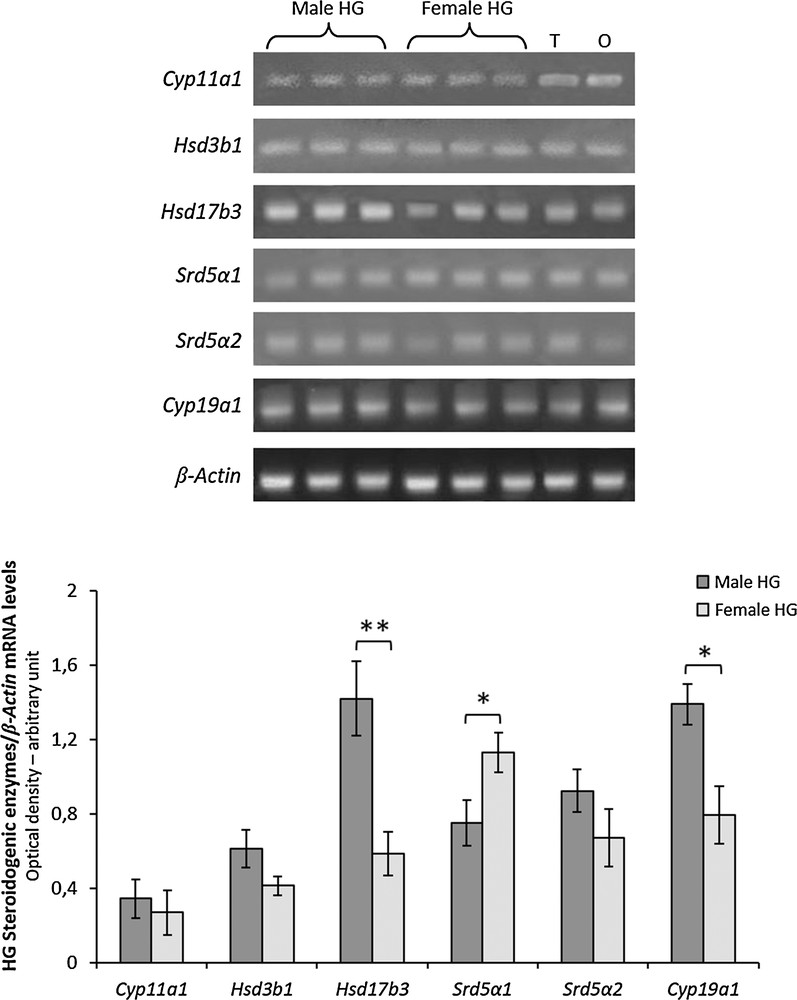
RT-PCR-analysis of Cyp11a1, Hsd3b1, Hsd17b3, Srd5a1, Srd5a2 and Cyp19a1 in HG from three male and three female rats. The upper panel shows the mRNA levels normalized with respect to β-actin mRNA levels; lower panel, the levels of HG mRNA steroidogenic enzymes were quantified using the ImageJ program and normalized with respect to β-actin mRNA. T, testis; O, ovary: positive controls. Values shown represent the means ± S.D. of three animals. *P < 0.05; **P < 0.01.
3.3 5α reductase and cytochrome P450 aromatase activities
Rat HG and testis (control tissue) homogenates were incubated with testosterone and 5α-Red or P450 aro enzyme activities were measured by evaluating the in vitro conversion rate of testosterone to 5α-dihydrotestosterone or 17β-estradiol, respectively.
The addition of testosterone to both male and female HG homogenates produced a dose-dependent increase of both 5α-Red and P450 aro activities (Table 2).
After addition of 2 μM testosterone, no significant differences in 5α-Red activity were observed between sexes, whereas after addition of 18 μM testosterone, a significant higher 5α-Red activity was observed in female than in male HG (Table 2).
After addition of both 2 μM and 18 μM of testosterone, the P450 aro activity was significantly higher in male than in female HG (Table 2).
Following the addition of 2 μM and 18 μM testosterone to testis homogenates 5α-Red activity was 3 ± 1 UE and 21 ± 5 UE, respectively; P450-aro activity was 2 ± 1 UE and 6 ± 2 UE, respectively.
4 Discussion
Numerous studies have clearly demonstrated that HG activity is influenced by steroid hormones and that androgens are responsible for sexual dimorphism in the hamster HG [2,41]. The occurrence of steroidogenic enzymes in HG tissues have not been documented to date, though studies strongly suggest a local synthesis of 5α-dihydrotestosterone due to the presence of 5α-Red activity [20,27]. The biosynthesis of steroid hormones begins upon mobilization of cholesterol from cellular stores to the mitochondrial inner membrane [42–44]. A large body of evidence indicates that the intramitochondrial transport of cholesterol is primarily mediated by the StAR protein, which is considered to be the rate-limiting step in steroid hormone biosynthesis [25,26,41,42,45,46]. In this regard, numerous studies have demonstrated a tight correlation between the synthesis of the StAR protein and the synthesis of steroids in a variety of classical (e.g., adrenal and gonadal) and non-classical (e.g., glial cells and skin) steroidogenic tissues [42,47–50]. The results of the present study demonstrate, for the first time, the expression of the StAR protein in the rat HG. We found a clear sexual dimorphism of StAR mRNA and protein expressions, which were more pronounced in males than in females. Furthermore, immunohistochemical investigations demonstrated that the StAR protein is more represented in B cells than A cells. It has been described that, in the rat HG, A and B cells represent different stages of a secretory cycle, with B cells representing the initial stage and A cells the end of the cycle activity [40,51]. B cells contain more numerous non-swollen mitochondria than A cells, and therefore are likely to display a more intense steroidogenic activity.
The results also demonstrated that the rat HG expresses Cyp11a1 (gene for P450scc), Hsd3b1 (gene for 3β-HSD) and Hsd17b3 (gene for 17β-HSD) and that in males, Hsd17b3 expression levels are far higher than in females. P450scc converts cholesterol to pregnenolone and 17β-HSD catalyzes the conversion of androstenedione to testosterone. In support of this view, it has been reported that both pregnenolone and testosterone elicit physiological effects on the HG [20]. Interestingly, Vilchis et al. [52] isolated the cDNA for P450scc in the HG of Syrian hamster.
Most steroidogenic enzymes present in the HG, such as StAR, P450scc, 3β-HSD and 17β-HSD are involved in de novo steroidogenesis, i.e. starting from the precursor cholesterol, in contrast to other enzymes such as 5α-Red1/2 and P450 aro, which are mainly responsible for the conversion of testosterone in 5α-dihydrotestosterone and 17β-estradiol, respectively [53]. Our results demonstrated that transcripts of 5α-Red1 were higher in the female than in the male rat, whereas those of P450 aro were higher in the male than in the female HG. It is noteworthy that Ramos et al. [28] isolated specific cDNAs for 5α-Red1, 5α-Red2 and 5α-Red3 in the hamster HG. As opposed to 1 and 2 isoforms, 5α-Red3 does not seem to drive the reduction of steroids. In a comparison among the three 5α-Red, the type 3 isozyme was found to have a low identity with type 1 (19%) and type 2 (17%) isozymes, whereas the homology between hamster type 1 and 2 reductases was nearly 40% [54]. However, they found that 5α-Red1 mRNA levels were higher than 5α-Red2, but no sexual dimorphism was shown.
Furthermore, our in vitro experiments demonstrated a higher 5α-Red activity in female as compared to the male HG and vice versa with a higher P450 aro activity in males than in females. This finding suggests a higher conversion rate of testosterone to 5α-dihydrotestosterone in females and a greater conversion of testosterone to 17β-estradiol in males. While there are no data related to P450 aro activity in the HG, Vilchis et al. [27] reported a higher 5α-Red activity in the female than in the male hamster HG. It is known that the HG of the hamster efficiently transforms testosterone to 5α-dihydrotestosterone, a necessary conversion to maintain the male glandular pattern. In fact, the activity of 5α-Red in the HG displays a marked androgen-regulated sexual dimorphism. No evidence of a sexually dimorphic Hsrd5a3 pattern was observed in the HG, suggesting that this expression is not influenced by androgens [54].
It is well known that, in some rodents, the HG is involved in chemical communication. In the gerbil HG exudates act as an attractant pheromone when spread throughout the pelage. The Harderian exudates in the male guide proceptive behavior in the female [10]. It has been speculated that Harderian spread allows the female to assess the reproductive competence of the male. The same findings have been reported by Payne [1] in the golden hamster, where homogenates of female HG lower aggressive male behavior toward other males whose faces are coated with these. We speculate that the sex steroid hormones locally synthesized in HG could be involved in the pheromone secretion and therefore regulate the sexual behavior in males and females.
In conclusion, the results of the present study indicate that Harderian gland can be classified as a steroidogenic tissue because it synthesizes cholesterol, expresses StAR and the steroidogenic enzymes involved in both androgen and estrogen synthesis as well as in the other steroidogenic tissues [46,47]. Finally, the dimorphic expression and activities of steroidogenic enzymes could suggest sex-specific hormonal effects into HG physiology.
Disclosure of interest
The authors declare that they have no competing interest.
Acknowledgements
This work is supported by University of Campania L. Vanvitelli.