1 Introduction
The babirusa (Genus Babyrousa) is a pig endemic to the Indonesian island of Sulawesi and a number of smaller islands in its vicinity [1]. The unusual anatomy of the maxillary canine teeth in the adult male, which rise through the skin beside the nose and curl over the face towards the forehead, has made its skull (Fig. 1) an object of curiosity for centuries [2–4]. The corresponding teeth in the female are either small or absent [5]. Recent studies have demonstrated how the maxillary canine teeth of the young male babirusa grow, from an intra-oral, latero-ventral orientation to a curved dorsally-pointing position having punctured the epidermal cover at the side of the nasal bones [5]. Although there have been a number of descriptions of the maxillary teeth in individual adult babirusa [6–10], the few population analyses of maxillary canine tooth morphology in adult male babirusa have been largely restricted to questions of taxonomy [11,12]. There has been no systematic analysis of the continuous growth of the anatomically rootless, maxillary canine tooth of the adult babirusa. In the current paper two main hypotheses were investigated (a) whether there is any relationship between the growth of the subcutaneous and the supracutaneous portions of the tooth and (b) are there changes in the subcutaneous angular growth pattern that would cause the tooth tip to avoid penetrating the cranium?

Series of right lateral views of the skulls (A = AAM0399; B = AAM0215; C = AAM0064; D = AAM0212; E = AAM0289) of the Buru babirusa (Babyrousa babyrussa) illustrating the caudal direction of growth in length of the maxillary canine teeth (Scale = 50 mm).
2 Materials and Methods
A total of 149 adult male babirusa skulls from twenty-two international museum and private collections were studied (Supplementary Table 1). Of these, one series of 49 skulls represented babirusa from Buru Island. A second series of 92 + 8 skulls, represented Sulawesi + the Togian Islands’ babirusa. None of these skulls came from zoo-bred animals.
The lengths of the subcutaneous and the supracutaneous portions of the left maxillary canine tooth were measured along the line of greatest curvature in respectively each of 22 and 19 intact maxillary canine teeth from Sulawesi babirusa skulls. The length of the subcutaneous portion of the tooth indicated the ‘depth of alveolar support’.
Measurements were made of the curvature observed in subcutaneous portion of 22 and in the supracutaneous portion of 25 left maxillary canine teeth from Sulawesi. The angle subtending a 20-mm arc on the lesser curvature of the maxillary tooth was measured at the open apex of the tooth (subcutaneous) and 5 mm distal to its exit from the alveolus (supracutaneous) and overlying epidermis. At each location the angle (a) subtended by the 20-mm arc, and the radius to that curvature (R) were measured (Supplementary Fig. 1).
Measurements were made of the external lateral length of each left maxillary alveolus, from the alveolar rim to its apex, in 41 of the Sulawesi babirusa.
For the statistical analysis of variation in the size of the left maxillary tooth measurements at different stages of animal growth of Sulawesi babirusa (the lengths of the intra-alveolar support, the angle of curvature within the alveolus, the angle of curvature of the supracutaneous portion) one-way analyses of variance (ANOVA) were carried followed by post hoc pairwise Tukey comparisons between groups (Tukey). The association between the depth of the alveolar support, and the supracutaneous length of the left maxillary teeth was assessed with a Pearson's product moment correlation coefficient. All statistical analyses were carried out in R [13], with P < 0.05 taken to indicated statistical significance. Figs. 6 and 9 were drawn using the wesanderson package [14].

A. The supracutaneous length of the left maxillary tooth of Sulawesi babirusa. B. The depth of the alveolar support of the left maxillary tooth. C. the relationship between these two measurements at different stages (A–E) of animal growth in size. B. Overall P–overall one-way ANOVA result, with statistically significant post hoc pairwise Tukey comparisons between groups indicated by horizontal bars and * P < 0.05, ** P < 0.01, *** P < 0.001. C. Pearson's product moment correlation coefficient (ρ) and associated P-value also included.

A. The angle of curvature of the left maxillary tooth within the alveolus. B. The angle of curvature of the supracutaneous portion of the left maxillary tooth, at different stages (A–E) of animal growth in size. Overall P–overall one-way ANOVA result, with statistically significant post hoc pairwise Tukey comparisons between groups indicated by horizontal bars and * P < 0.05, ** P < 0.01, *** P < 0.001.
3 Results
3.1 Maxillary canine teeth
The growth of the maxillary canine teeth of the Buru babirusa is illustrated in Figs. 1 and 2. Prior to any measurements being made, this continuous process was characterised as five stages according to the supracutaneous length and the position of the tip of the tooth over the bones of the skull: A (1 skull), B (8 skulls), C (14 skulls), D (11 skulls) and E (15 skulls). Following penetration of the skin of the nose, the supracutaneous portion of the teeth, which had a circular cross section near the tip, grew in a curve-linear fashion dorso-caudally (Fig. 1A). Their growth from the alveoli was also orientated such that the tips grew towards the midline of the cranium (Fig. 2A). As growth proceeded over the nasal bones the approximately 45-degree angle of orientation towards the midline was reduced to about 28-degrees (Fig. 2A–C). In the later stages of their growth, the tips of the maxillary teeth were carried towards and over the frontal bone and the angle of orientation with respect to the midline was reduced to about 14 degrees (Figs. 1D and E; 2D and E; 3). The teeth also became irregularly oval (rostro-caudally) in cross section. In eight of the skulls, one or both the maxillary canine teeth in category ‘E’ was/were broken. None of the teeth in the other four categories were broken.

Series of dorsal views of the skulls (A = AAM0399; B = AAM0215; C = AAM0064; D = AAM0212; E = AAM0289) of the Buru babirusa (Babyrousa babyrussa) illustrating the medio-caudal direction of growth in length of the maxillary canine teeth (Scale = 50 mm).

Frontal view of the skull (AAM0212) of a Buru babirusa (Babyrousa babyrussa) illustrating the convergent nature of normal maxillary tooth growth (Scale = 50 mm).
The corresponding pattern of growth of the supracutaneous portion of the maxillary teeth in the Sulawesi and Togian babirusa is illustrated in Fig. 4. The teeth of these animals were initially round in cross section, and thereafter irregularly oval (rostro-caudally), as their growth extended them towards and over the frontal bone. Their continuous growth was also characterised as five stages according to the position of the tip of the tooth over the bones of the skull (Fig. 4): A (7 skulls), B (9 skulls), C (14 skulls), D (26 skulls) and E (42 skulls). Following penetration of the skin of the nose, the teeth elongated dorso-caudally in a fairly tight curve-linear fashion (Fig. 4A and B). Thereafter they grew more dorsally over the nasal and frontal bones (Fig. 4C–E).

Series of five right lateral views of the skulls (A = AAM0710; B = AAM0543; C = AAM0325; D = AAM0664; E = AAM0338) of the Sulawesi babirusa (Babyrousa celebensis) illustrating the caudal direction of growth in length of the maxillary canine teeth until over the frontal and parietal bones (Scale = 50 mm). The tip of the right tooth (E) is sufficiently worn to expose the axial pulp cavity.
In 38 of the Sulawesi babirusa skulls, one or both of the maxillary canine teeth were broken, namely in categories D (9) and E (29). None of the teeth in the other three categories was broken.
The increase in supracutaneous length with animal growth stage is illustrated in Fig. 6A, with mean supracutaneous lengths increasing from 81.3 mm (43.8–118.9 mm) in growth stages ‘A’ to 215.3 mm (177.8–252.9 mm) in ‘E’. The subcutaneous (alveolar depth) support provided to the maxillary canine tooth also increased in size with animal growth stage (ANOVA P = 0.003, Fig. 6B). The mean extent of the alveolar support increased from 37.3 mm (95% confidence intervals: 29.9–44.4 mm) and 41.7 mm (34.2–49.1 mm) in growth stages ‘B’ and ‘C’, respectively, to 54.3 mm (49.4–59.2 mm) in growth stage ‘E’ (Tukey P < 0.037). Supracutaneous and subcutaneous lengths were statistically significantly positivity correlated (ρ = 0.684, P = 0.003), with increasing alveolar support as the erupted portion of the tooth lengthened (Figs. 5 and 6C).

Medial view of Sulawesi babirusa left maxillary canine teeth of young (B = AAM0368) and older (D = AAM0367) adult animals illustrating the relative intra-alveolar and extra-alveolar lengths (Scale = 20 mm).
The apical end of the maxillary tooth was open and very thin-walled (Figs. 5 and 7A). The tooth wall thickened as it progressed up the alveolus and the pulp cavity narrowed. The lumen of the tooth was filled with a cone of well vascularised dental pulp (Fig. 7B–D). The pulp cavity extended within the length of the tooth almost to its tip (Fig. 8A).
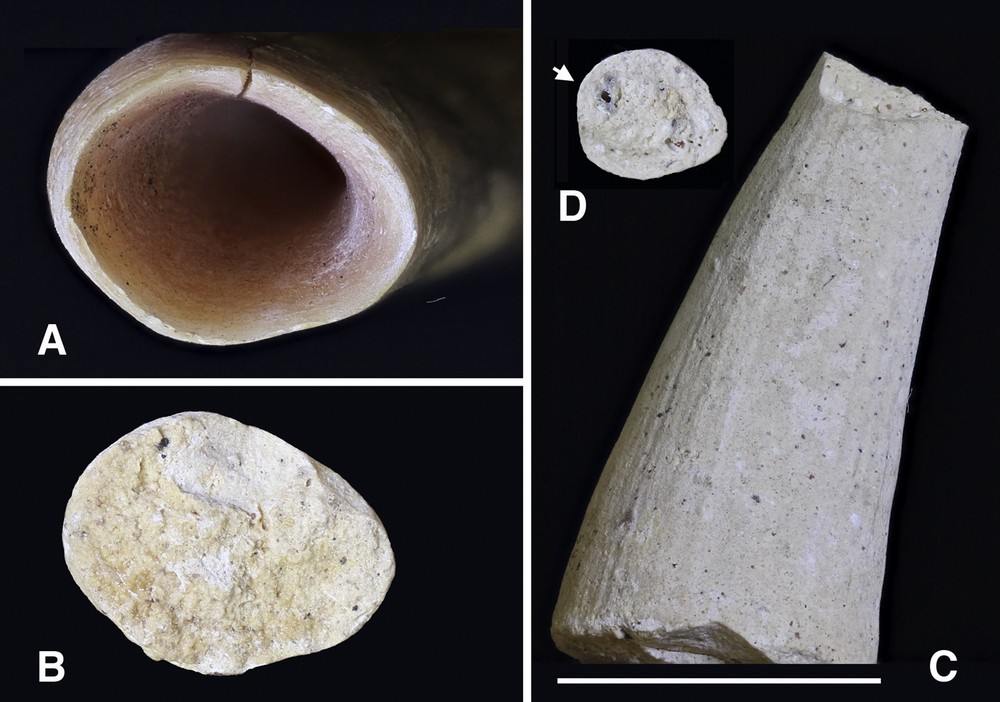
A. The open apex of the left maxillary tooth of a Sulawesi babirusa (AAM0111). B. The well vascularised apical surface of the dehydrated pulp ‘cone’ extracted from the maxillary tooth. C. The well vascularised outer surface of the curved ‘cone’ of dehydrated pulp extracted from the apical end of the maxillary tooth. D. The narrow rostral (‘tip’) surface of the ‘cone’ of dehydrated pulp illustrating the larger () and smaller blood vessels (Scale = 10 mm).

A. The right maxillary canine tooth of a Sulawesi babirusa (AAM0353) demonstrating that the pulp cavity, exposed by face rubbing behaviour, extends towards the tip of the tooth. B. Dorsal view of the maxillary alveoli of a Sulawesi babirusa (AAM0102) illustrating on the left (L) side; a broken and slightly shrunken part of the dentin layer had been retained in the alveolus; its pulp core had been removed revealing the pulp-covered apical floor of the alveolus. On the right (R) side of the nose are shown the internal structure of the wall of the alveolus and the pulp-covered apical floor (Scale = 10 mm).
3.2 Curvature of the maxillary canine teeth
The angle of curvature of the tooth (a) within the alveolus statistically significantly decreased with animal growth stage (Figs. 5 and 9A, ANOVA P < 0.001), with mean angles in growth stage ‘B’ of 19.8° (17.3–22.3°) being greater (Tukey P < 0.001) than growth stages ‘C’ (10.7° (8.2–12.2°)), ‘D’ (10.9° (9.5–12.4°)) and ‘E’ (7.4° (5.7–9.0°)). Angle of curvature in growth stage ‘E’ was also smaller compared to Group ‘D’ (Tukey P = 0.014). The corresponding supracutaneous angle of curvature also statistically significantly reduced with animal growth stage (Figs. 5 and 9B, ANOVA P < 0.001), with mean angles in growth stages ‘A’ (36.8° (33.6–40.1°)) and ‘B’ (30.7° (27.4–33.9°)) greater than those of growth stages ‘C’ (18.8° (15.6–22.1°)), ‘D’ (16.0° (14.1–17.9°)) and ‘E’ (10.7° (8.6–12.8°)) (Tukey P < 0.001). In addition, growth stage ‘E’ had a smaller corresponding supracutaneous angle of curvature compared to growth stages ‘C’ and ‘D’ (Tukey P < 0.007).
The growth of the tooth from the alveolus was orientated such that it grew towards the median plane of the cranium at an angle of about 45 degrees (Fig. 2). As the animals grew older this angle diminished in size (to about 14 degrees) and the orientation of the teeth tended to become more nearly parallel to the median plane.
3.3 Maxillary canine tooth alveolus
The external structure of the maxillary alveolus is illustrated in Figs. 1, 4 and 8, and the internal structure is illustrated in Figs. 8B and 10. In all skulls the exit from the alveolus was oval in shape. The orientation of the long axis of the exit was most often parallel to the median plane of the skull, but in nine instances it was at an angle of between 5 and 45 degrees convergent to the median plane. The external lengths of the maxillary alveoli of Sulawesi babirusa divided into 2 clusters (ANOVA P < 0.001, Fig. 4): growth stages ‘A’ (34.7 mm (27.5–41.8 mm)) and ‘B’ (34.5 mm (25.8–43.2 mm)) formed one cluster (Tukey P = 0.999) and the longer lengths in growth stages ‘C’ (45.7 mm (38.5–52.8 mm)) ‘D’ (45.3 mm (40.6–50.0 mm)), and ‘E’ (44.8 mm (42.3–47.2 mm)) forming the second cluster (Tukey P = 0.999). A flattened, bony bracket was arranged approximately vertically on the caudal external surface of the alveolus thickening that part of the alveolar wall (Fig. 8B). A thinner, flattened, bony bracket was also present rostrally, again thickening that part of the alveolar wall. Elsewhere the alveolar wall was somewhat thinner. A flat, intra-alveolar lamina dura covered the spongy bone of the alveolus (Fig. 8B). The apical region of the alveolus comprised a round, thin, flat perforated area of bone (Figs. 8B and 10).
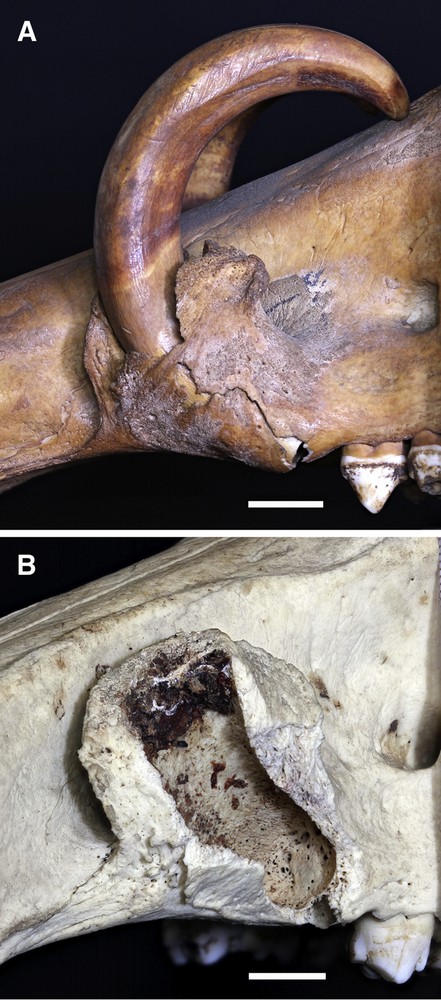
Maxillary alveolar structure. A. The external structure of the wall of the left alveolus of a young Sulawesi babirusa (AAM0091). Note the broken, thin, lateral wall of the alveolus and the exposed position of the apex of this tooth. B. The lumen of the alveolus of a slightly older Sulawesi babirusa (AAM0095). Note that the bony floor in the apical region of the alveolus is perforated with many openings for blood vessels. The alveolar wall comprises spongy bone with a thin covering sheet of lamina dura (Scale = 10 mm). The alveolus of the long-retained deciduous canine tooth is present ventral to the apical region of the permanent tooth.
4 Discussion
This study has demonstrated that the growth of the maxillary canine tooth of the adult male babirusa is a complex process. The evidence shows that normal growth of this tooth requires mechanisms to coordinate the changes in the intrinsic curvature of the tooth such that the caudally curled maxillary canine tooth grows up and over the nasal and frontal bones of the skull (Figs. 1 and 4). Mechanisms are also required to progressively adjust the horizontal angle of the direction of that growth with respect to the median plane of the head such that the tips of the maxillary canine teeth remain approximately adjacent to one another as the teeth elongate over the face of the babirusa (Fig. 3). In addition, remodelling of the bony supporting structure of the alveolus would appear to be an essential component facilitating the normal pattern of tooth growth.
The maxillary canine teeth of the male babirusa have been classified as ‘dentine hypsodont, euhypsodont, free growth teeth’ [15]. Rootless or continuously growing teeth are described as euhypsodont, although they have also been described as ‘hypselodont’ [15]. The category ‘dentine hypsodonty’ comprises elongated teeth with a surface that is dominated by dentine. In babirusa, both the upper and lower canines are reportedly free of enamel, and are therefore essentially dentine teeth [15]. Comparable dentine hypsodonty can be found in the maxillary canines of the warthog (Phacochoerus africanus), the narwhal (Monodon monoceros) and the walrus (Odobenus rosmarus), the incisors of the elephant (Elephas maximus and Loxodonta africana), and the molars of the sloth (Bradypus tridactylus) [15–17]. However, the tips of the tusks of juvenile walruses and young elephants are covered with a layer of hard enamel, which is worn away before adulthood [16,18]. Previous studies on the pattern of early development of the maxillary canine tooth of the babirusa concentrated on the subcutaneous rotation of the bony alveolus to bring the growing tooth unto a position that enabled its pointed tip to penetrate the overlying epidermal layer beside the nose [5]. Histological studies of the maxillary canine teeth of the juvenile babirusa remain to be carried out to see if enamel is present when the teeth puncture their way through the layers of skin beside the nose [5]. The freshly-erupted permanent maxillary canine of the Eurasian wild pig shows an unworn enamel cap [15].
The anatomy of the maxillary teeth of the babirusa is similar to those of the other pigs in that they all have ‘free growth’ from open roots. However, the babirusa is the only pig in which these teeth grow through the epidermis and rostro-caudally over the front of the face; the others extend laterally from the oral cavity and to varying degrees curl latero-dorsally and partially caudally towards and over the nose [19–24]. Growth in length of the babirusa canines, is therefore not compromised by abrasive contact with the mandibular canines, as is seen in these pig genera: Hylochoerus, Porcula, Potamochoerus, and Sus. The growth of the maxillary canine teeth in the adult male warthog do not rub on the lower canines, and become longer and more widely flared than those of the female after about 20 months of age [20,25]. Data on the growth in length of the maxillary canine of the Eurasian wild pig (Sus scrofa) has been compiled by Briederman [19].
The tips of the maxillary teeth of babirusa from Buru Island did not extend as far caudally over the skull as those from Sulawesi and the Togian Islands (Figs. 1, 2 and 4). This was not due to a sampling error of the wild population, as the wear of the molar teeth in groups ‘E’ of both groups of skulls was equivalent (Macdonald, unpublished). Deninger [11] pointed out that the maxillary teeth of the Buru skulls were thinner than those from Sulawesi, and concluded that the difference was genetically based. The length of the subcutaneous portion of the maxillary teeth from Sulawesi and the Togian Islands increased as the corresponding supracutaneous length increased (Fig. 6).
The cone-shaped pulp cavity, arising from the open root of the babirusa's maxillary canine tooth, was largely retained within the intra-alveolar portion of the tooth (Fig. 7). A comparable cone-shaped pulp cavity is present, also largely within the alveolus of the elephant tusk [26,27]. A narrow, centrally located and vascularised column of pulp then runs parallel to the longitudinal axis up much of the length of the babirusa tooth, and this could be seen in worn and broken teeth (Figs. 4E and 8A) [28]. In the current study a larger proportion of the Sulawesi and Togian skulls had broken upper canine teeth than the skulls from Buru. This would appear to reflect the lengths of the Sulawesi and Togian maxillary teeth and their greater susceptibility to significant wear of their lateral surfaces when their faces were rubbed against trees and other objects during marking behaviour [28–30].
Progressive changes in the angle of curvature of the tooth resulted in the tip of the tooth being lifted clear of the nasal and frontal bones (Figs. 1 and 4). These changes in curvature could be seen developing on the tooth within the alveolus (Fig. 9A). There was also a reduction in the angular relationship of the tooth with the median plane of the skull as the tooth lengthened (Fig. 2). In some skulls the tips of the two maxillary canines were in contact (Fig. 3) and in others the region of direct contact between the teeth was indicated by patches of wear on the distal-medial side of each tooth [28]. It is likely that the lateral pressure exerted by the teeth upon one another was transmitted back to the alveolus and influenced the construction of new tooth material. It is perhaps also conceivable that the ‘ploughing’ behaviour expressed by adult males, when they appear to be depositing oral or eye-gland secretions in soft mud or sand [28,30], could likewise induce cellular reaction within the alveolus and subsequent maxillary tooth growth orientation. Rotation, during its formation within the alveolus, may account for the spiral of the open rooted babirusa maxillary canine. The physical and cellular mechanisms involved remain to be investigated. The comparable triggers generating the anti-clockwise spiral of canine tooth of the Narwhal, a distant Cetartiodactyla relative of the babirusa, also remain to be explained [31,32].
Examination of the construction and remodelling of the maxillary canine alveolus during the juvenile growth of the babirusa skull revealed that it rotated its bony structure and encapsulated tooth through approximately 90 degrees, from pointing rostrally to pointing dorsally [5]. The valley formed between the alveolus and the nose allowed for the passage of the tendons of the Musculi labii superioris and M. depressor labii superioris to the snout [33,34]. The alveolus is therefore attached to the skull by means of approximately the ventral 50% of its length (Figs. 3 and 10). Blood and nerve supplies to the tooth in Sus arise from branches of the infra-orbital artery and nerve respectively, themselves branches of the maxillary artery and nerve [35]. The adult modifications to the anatomical structure of the babirusa alveolus result in a relative straightening of the sides of the alveolar lumen (Figs. 8B and 10). Although the internal dimensions of the alveolus were not examined in detail, the interior length of the alveolus could be interpreted from the subcutaneous portion of the maxillary tooth (Figs. 5 and 6). Its increase corresponded to the measured increase in the external length of the bony alveolus. However, this increase in tooth-root length was small relative to the increase in length of the subtended supracutaneous part of the tooth (Fig. 6B).
The cone-shaped deposit of pulp from the open apical portion of the babirusa's canine tooth (Fig. 7) implied that the tooth dentine was being laid down incrementally as the tooth grew in length from the open apex. In the rabbit, a row of columnar odontoblasts lines the periphery of the pulp bordering the dentine and these become low cuboidal cells as the cone of pulp tapers [36]. After cytoplasmic maturation, odontoblasts move in a centripetal direction and deposit ivory along their pathway [18,37,38]. As the pulp chamber of the babirusa tooth narrowed the primary dentine layers increased in thickness. Comparable development can be seen in the rabbit and elephant [27,36]. Currently, however, there is no detailed histology for the babirusa. The only microscopic examination of the babirusa tooth was carried out by Kollmann [39] on a maxillary canine tooth, probably from an animal from Buru Island, and a molar tooth from a different male. The cross-sectional image of the cement layer of the former appeared to show parallel dentinal tubules (Fig. 11). Neither the illustration nor the accompanying text gave details of these structures. Transverse profiles of Eurasian wild pig and warthog canines demonstrated that their dentinal tubules were not exactly transverse to the long axis; they lay at an angle pointing from the forming face towards the tip, so that transverse profiles cut them into short lengths [16]. Dentine growth bands in these pigs were narrow, and reflected the way in which the matrix gave the curled dentinal tubules (that create growth bands) the freedom to cluster into skeins, with curls and skeins being more clearly seen in the warthog, than in the Eurasian wild pig [16]. The overlying cement layer of the babirusa canine tooth also showed narrow bands (Fig. 11), which may have reflected comparable banding in the dentine layers of the tooth. Kollmann [39] found that Sharpeys fibres lay between the cementocytes, and extended to the tooth surface. Sharpey's fibres are the terminal ends of principal fibres (of the periodontal ligament) that insert into the cementum of the tooth and into the periosteum of the alveolar bone [40].

A cross-sectional image of the cement layer of the maxillary canine tooth of a male babirusa (published by Kollmann, 1873). Abundant cementocytes are shown in the banded cement layer (C). Sharpeys fibres are indicated between the cementocytes, and these extend to the tooth surface (S). The narrow, illustrated amount of the dentine layer (D) appears to contain parallel dentinal tubules.
No attempt was made to define the chronological age of the animals from these data. In addition, unknown regional differences in the diet and substrate induced wear of molar and premolar teeth precluded the identification of corresponding age-related characters [28,30]. Although analysis of suture fusion might have offered a different age-related character, there was no clearly visible pattern; perhaps the scent-marking ploughing behaviour of the male babirusa [30] contributed towards this apparent absence of clear cranial suture-fusion patterns.
What then is the purpose of the maxillary canine tooth of the babirusa? The suggestion that it is decoration and acts to differentiate between males has not been supported by studies carried out in the wild [41] or within zoological or safari park environments [42–44]. No evidence has yet been recognized of female babirusa preference of one male over another based on maxillary tooth morphology. Unlike in some other suids, the maxillary tooth of the babirusa, like its nose, is not used as an implement for digging, and has been deemed to be relatively fragile and susceptible to breakage [1,28,42]. This observation is reflected in the results of the present study of skulls from Sulawesi. It is also not used as a weapon against predators nor is it employed as such in agonistic encounters with other babirusa [41,43]. Indeed, the role of the curved maxillary teeth of the subservient (losing) male in protecting the throat of the superior male is ironic, but appears to be their only recognised function so far [43].
Acknowledgements
We gratefully acknowledge the kind hospitality and support of Friederike Johansson, Göran Nilson and Bianca Ziehmer during these studies. We would also like to thank the curators and staff of the following museums for access to the babirusa skeletal material that form part of their collections: Zoologisk Museum, København, Denmark; University Museum of Zoology, Cambridge, England; Natural History Museum, London, England; Oxford University Museum of Natural History, Oxford, England; Museum für Naturkunde, Berlin, Germany; Senckenberg Naturhistorische Sammlungen Dresden, Germany; Naturmuseum Senckenberg, Frankfurt Am Main, Germany; Zoologische Staatssammlung München, Germany; Private H.M. Collection, Bogor, Indonesia; Museum Zoologicum Bogoriense, Cibinong, Indonesia; Museum Wallacea, Universitas Haluoleo, Kendari, Indonesia; Universitas Tadulako, Palu, Indonesia; Zoological Museum Amsterdam, The Netherlands; Naturalis Biodiversity Center, Leiden, The Netherlands; National Museum of Scotland, Edinburgh, Scotland; Göteborgs naturhistoriska museum, Göteborg, Sweden; Naturhistoriska Riksmuseet, Stockholm, Sweden; Naturhistorisches Museum Basel, Switzerland; The Field Museum, Chicago, USA; American Museum of Natural History, New York, USA; National Museum of Natural History, Washington, USA. We would like to thank Aline Brodin, Edinburgh University Library, for translations into French. We are grateful to the University of Edinburgh and the Balloch Trust for financial support.