1 Introduction
Dendrimer chemistry has received a great attention in the last few years [1–7] and it is not the aim of this chapter to review the field. We intend to discuss some, in our opinion, representative examples taken by our own work, to illustrate as the use of suitable functionalization in the core, or at the periphery of a dendritic structure can lead to interesting photo-induced functions. In particular we divided the chapter in four main sections:
- • ruthenium polypyridyl-cored dendrimers;
- • light responsive and ph sensitive azobenzene-functionalized dendrimers;
- • dansyl-functionalized dendrimers;
- • Hamilton receptor-functionalized dendrimers.
2 Ruthenium polypyridyl-cored dendrimers
2.1 Site isolation
An approach commonly used to prevent intermolecular interactions between chromophores, that could lead to undesired processes such as their self-aggregation or quenching of their luminescence, is site isolation. In some cases, in fact, it is desirable to prevent the interaction with the environment (solvent, dioxygen, other molecules present in solution…) and maintain the properties of a chosen chromophore as it would be in a protected microenvironment. A way to achieve such isolation is by introducing substituents on the chromophore such as dendritic wedges [8]. By the introduction of dendritic substituents, (i) a microenvironment is created around the chromophore, which is inaccessible to certain molecules or ions because of such a great difference in polarity, that they cannot be ‘solubilized’ by the dendritic wedges, and (ii) a barrier is created based on steric crowding, which makes the core unreachable for large molecules.
Novel [Ru(bpy)3]2+ complexes were synthesized using 2,2'-bipyridine ligands bearing dendritic wedges on the 4,4'-positions. Both Newkome-type poly(ether amide) wedges [9] and Fréchet-type poly(benzyl ether) wedges [10] were successfully employed to surround the [Ru(bpy)3]2+ core. The second generation of each type is depicted in Fig. 1.

Second generations of the Fréchet-type 1 and 2 and the Newkome-type 3 [Ru(bpy)3]2+ complexes [9,10].
The [Ru(bpy)3]2+-cored dendrimers exhibited the expected absorption and emission properties of [Ru(bpy)3]2+ compounds [11]. However, in all cases, the excited state lifetime was found to be much longer than expected in the air-equilibrated solutions. Obviously, the dendritic wedges are capable of protecting a [Ru(bpy)3]2+ core to a very large extend from 1O2 (singlet oxygen), which is commonly known as an efficient triplet quencher. Since 1O2 is only a very small quencher molecule, the site isolation effect should in this case rather be related to the ‘insolubility’ of 1O2 in the dendritic wedges, than to the steric crowding introduced around the [Ru(bpy)3]2+ core by the branches. Interestingly, the lifetime of a second-generation Newkome-type dendrimer was found to be twice as long and the emission red-shifted with 30 nm compared to a third-generation Fréchet-type dendrimer in aerated acetonitrile, indicating that the more polar Newkome-type dendrimers stabilize better the triplet metal-to-ligand charge-transfer excited state (3MLCT) of the [Ru(bpy)3]2+ core.
2.2 Photo-induced electron transfer (PET)
To explore in more detail the accessibility of the [Ru(bpy)3]2+ core by quencher molecules, the interaction between the cationic quencher methyl viologen (MV2+), the neutral quencher tetrathiafulvalene (TTF), and the anionic quencher anthraquinone-2,6-disulfonate (AQ2–) and the Fréchet-type dendritic [Ru(bpy)3]2+ complexes were investigated [10]. These three quencher molecules are easy to reduce (MV2+, AQ2–) or oxidize (TTF) and the excited state of the ruthenium moiety has enough energy to undergo to reductive or oxidative electron transfer. Therefore, upon excitation of [Ru(bpy)3]2+ a very efficient bimolecular photo-induced electron transfer is observed in solution with all the above-mentioned quenchers. Upon encapsulation of the ruthenium core into a dendritic structure a site isolation effect was observed in all cases. The quenching efficiency as well as the rate of the electron transfer process, decreases going to higher generations. However, the rate constants for the quenching reactions involving the different quencher molecules were not always predictable. Several factors were suggested to influence the charge transfer processes: (i) the dependence of the diffusion rate constants on the radius of the compound, (ii) the protection of the dendritic wedges with respect to diffusion of the quencher within the dendrimer, (iii) the competition between the solvent and the dendritic branches for the solvation of the core, (iv) Coulombic interactions between the positively charged core and the charged quenchers, and (v) the folding of the dendritic branches around the core.
2.3 Light-harvesting (energy transfer)
The substitution of [Ru(bpy)3]2+ with dendritic wedges does not only provide a shielding of the luminescent core from quenchers, but can also provide a possibility to introduce functionality at the periphery of the dendrimer. For example, suitable chromophores decorating the periphery of the dendrimer can lead to an efficient energy transfer from the excited chromophores to the ruthenium core [12]. Such a light-harvesting system has the advantage of a large absorption cross-section and thus an efficient collection of the incident light that can be funnelled to the ruthenium moiety.
For this purpose, Fréchet-type dendrimers bearing a [Ru(bpy)3]2+ moiety in the core and naphtyl units at the periphery have been synthesized [13]. A schematic representation of this type of dendrimers is given in Fig. 2.

Schematic representation of a third-generation Fréchet-type [Ru(bpy)3]2+ complex bearing light-harvesting naphtyl moieties at the periphery [13].
Since the chromophoric groups present in the dendritic complex are separated by aliphatic connections, the interactions between the chromophores were found to be weak. The bare wedges show an emission band in the region of the naphtyl-type units. This emission is almost completely quenched in case of the [Ru(bpy)3]2+ cored dendrimer, regardless the excitation wavelength. This indicates that a very efficient energy transfer process occurs from the aromatic units in the wedges to the [Ru(bpy)3]2+ moiety in the core.
3 Light responsive and pH sensitive azobenzene-functionalized dendrimers
3.1 Photophysical properties of azobenzene
Azobenzene and its derivatives have been widely investigated for their photophysical properties and in particular for their reversible photo-isomerisation reactions (Fig. 3). Their photochromic behaviour has been extensively used to construct photoswitchable devices [14–17]. Azobenzene compounds do not show any appreciable fluorescence or phosphorescence, unless they are sterically hindered. The photo-isomerisation of azobenzenes is known to be one of the cleanest photoreactions, fully reversible and quite fatigue resistant. The thermodynamically stable E isomer can be photochemically converted to the Z form, which is converted back to the E form either by light excitation or thermally (Fig. 4).
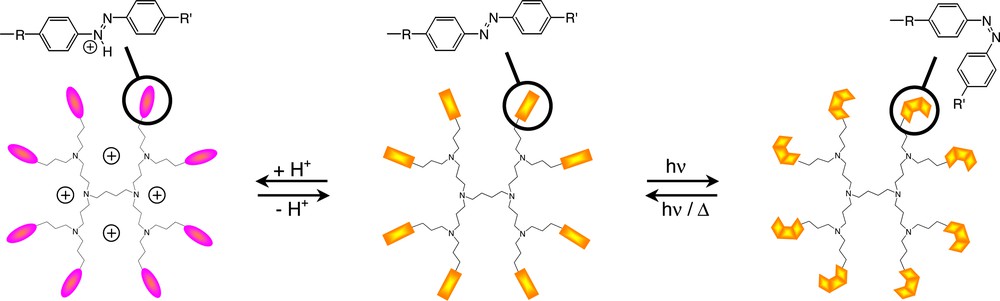
Photoactive and pH responsive poly(propyleneamine) dendrimers.

The E/Z isomerisation of azobenzene.
The two isomers have somewhat different absorption spectra. The E isomer shows an intense ππ* band in the near-UV region and a weak symmetry-forbidden nπ* band in the visible region. Upon isomerisation to the Z form the ππ* band shifts towards shorter wavelengths, while a significant increase is observed in the nπ* band. The isomerisation of azobenzene involves a large structural rearrangement and large change in the dipole moment. The rate and efficiency of the photoreaction, as well as their absorption spectra, are strongly influenced by the substituents on the azo-moiety.
3.2 Holographic materials
To explore the possibility of using dendrimers as photoswitchable materials, and therefore to have a large number of photo-isomerisable groups implemented on the same molecule, poly(propyleneamine) dendrimers have been functionalized at the periphery with either meta- or para-azobenzene moieties [18,19].
The properties of the azobenzene are maintained in the azobenzene-functionalized dendrimers, suggesting the absence of a strong interaction or steric crowding among the units. The azobenzene moieties could be reversibly switched, and the same quantum yield of photo-isomerisation for each unit, independently by the generation, was observed. Furthermore, the meta-azobenzene-functionalized poly(propyleneamine) dendrimers were, in contrast to the para-azobenzene-functionalized poly(propyleneamine) dendrimers, successfully employed in holographic materials. Holographic gratings with diffraction efficiencies up to about 20% can be optically recorded in thin films of the meta-azobenzene-functionalized poly(propyleneamine) dendrimers ((Fig. 5).
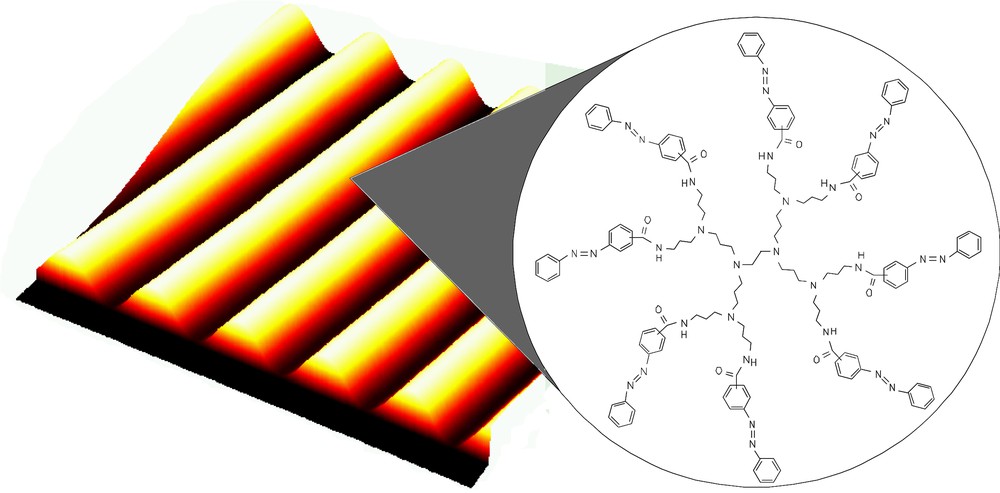
A first-generation azobenzene dendrimer employed as a holographic material [18].
3.3 Photo-isomerisable hosts
The clean and reversible photo-isomerisation of the azobenzene-functionalized poly(propyleneamine) dendrimers can be used to encapsulate guest molecules within the dendritic framework. This was demonstrated by applying the dye eosin Y as a guest molecule, as demonstrated for a fourth-generation para-azobenzene functionalized poly(propyleneamine) dendrimer in Fig. 6 [19].
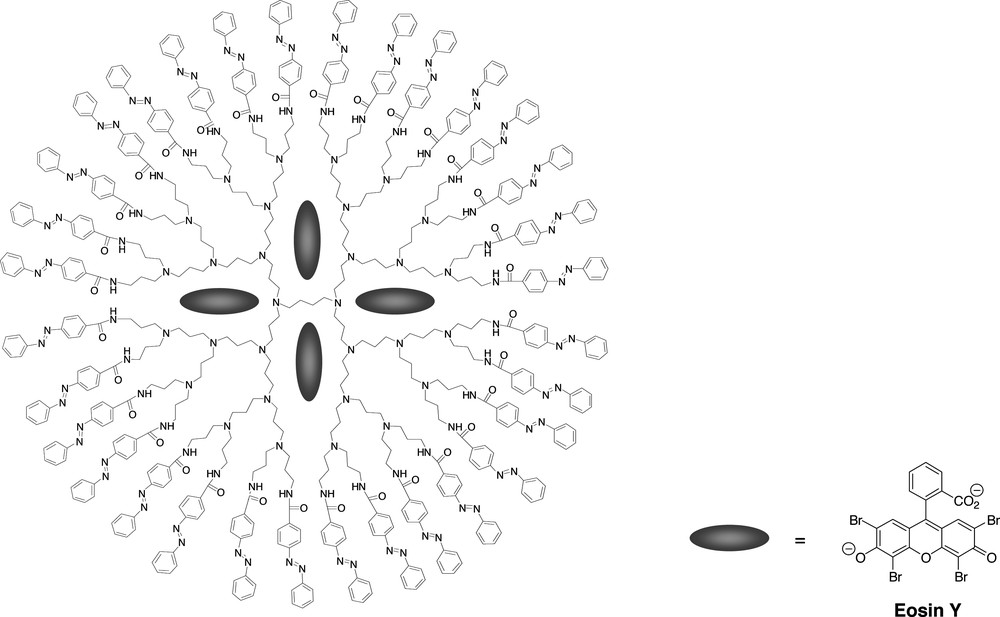
A fourth-generation para-azobenzene-functionalized dendrimer employed as a photo-isomerisable guest for eosin Y [19].
Eosin Y can sensitize the E/Z and Z/E photo-isomerisation reactions of the azobenzene units via a triplet–triplet energy-transfer mechanism, while its lowest singlet, fluorescent excited state can be quenched by the amines present in the dendrimer core. Fluorescence quenching experiments showed that eosin Y is hosted in the dendrimer and suggested that the Z forms are more efficient hosts than the E forms.
3.4 Methyl orange-functionalized dendrimers
Methyl orange is a well-known pH indicator belonging to the family of azobenzene derivatives. A clear colour change at the pH transition point (between pH 3.1 [red] and pH 4.4 [yellow] in H2O) [20] is observed upon protonation of the azo moiety, leading to an azonium ion, which is stabilized by mesomery ((Fig. 7) [21]. On the other hand, methyl orange is a light-responsive molecule, since it contains a photo-isomerisable azo moiety, which can be converted from the thermodynamically more stable E form into the Z form upon light excitation. Subsequently, the Z form will normally recover to the E form, either thermally or photochemically (Fig. 7).
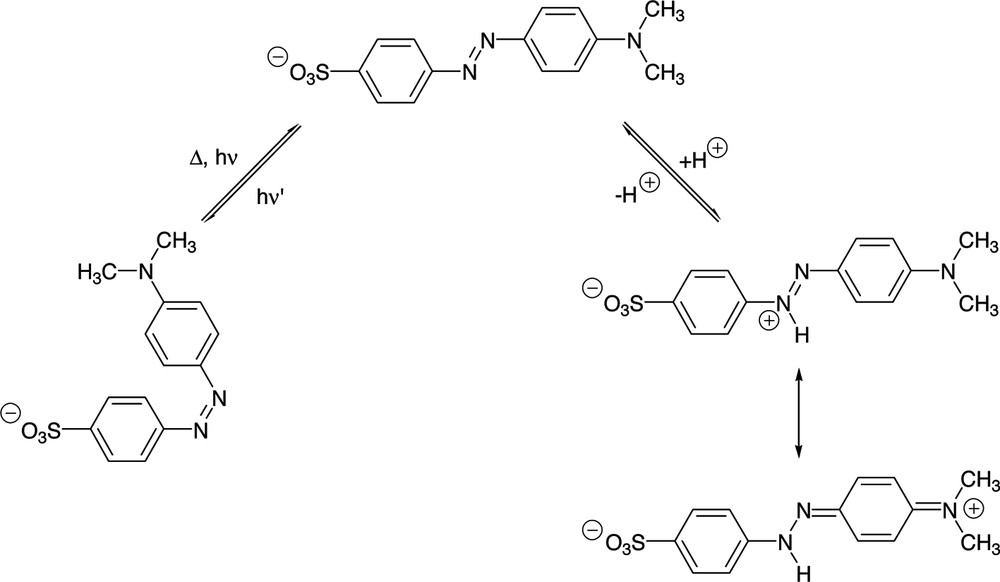
The protonation to an azonium ion and the E/Z isomerisation of methyl orange.
Therefore, this double functionality with respect to light and pH of methyl orange, once attached at the periphery of a dendrimer, is an interesting tool to study the possible interactions within dendritic molecules.
For the first time, poly(propyleneamine) dendrimers were functionalized with a pH indicator, namely methyl orange, which responds to two different external stimuli, i.e. pH and light. The structure of a third generation methyl orange-functionalized poly(propyleneamine) dendrimer is depicted in Fig. 8 [22].

Third-generation methyl orange-functionalized poly(propyleneamine) dendrimer [22].
Similar to the azobenzene-functionalized dendrimers (see above), the photophysical properties are generation independent, except for the molar extinction coefficient, which deviated significantly from the expected value in the case of generations 4 and 5. This significant deviation was attributed to the locally high concentrations of methyl orange units. Furthermore, both the E/Z and the Z/E isomerisation rates are extremely high compared to azobenzene itself [17,23], since the ‘push-pull’ system formed by the NMe2 group (electron donor) and the SO2 group (electron acceptor) lowers the energy barrier for the isomerisation process.
The response of the methyl orange units towards pH changes was found to be generation dependent (dendritic effect), and strongly influenced by the tertiary amines in the core. It is known that the tertiary amines placed in the interior of the dendrimer form the most basic sites and will be protonated first [24]. Obviously, the protonation of the methyl orange moieties is strongly affected by the positive charges already present in the core. For the higher generations, the color change is not so rapid and dramatic as for the monomer, indicating that not all the methyl orange groups can be protonated. A large excess of acid is necessary to induce a full colour change. The broadening of the absorption spectra of the protonated form may be the result of sharing protons between two methyl orange units. Once the methyl orange units are protonated, an emission is observed at 77 K.
4 Dansyl-functionalized dendrimers
4.1 Energy transfer
Another interesting chromophore that is sensitive to pH changes is the dansyl (5-(dimethylamino)-1-naphtalene-sulfonamido). Such compound is extensively used for sensing and labelling purposes, because of its intense absorption band in the near-UV and its strong fluorescence in the visible region [25–27]. In this view, poly(propyleneamine) dendrimers have been functionalized at the periphery with dansyl units (Fig. 9) [28–32].
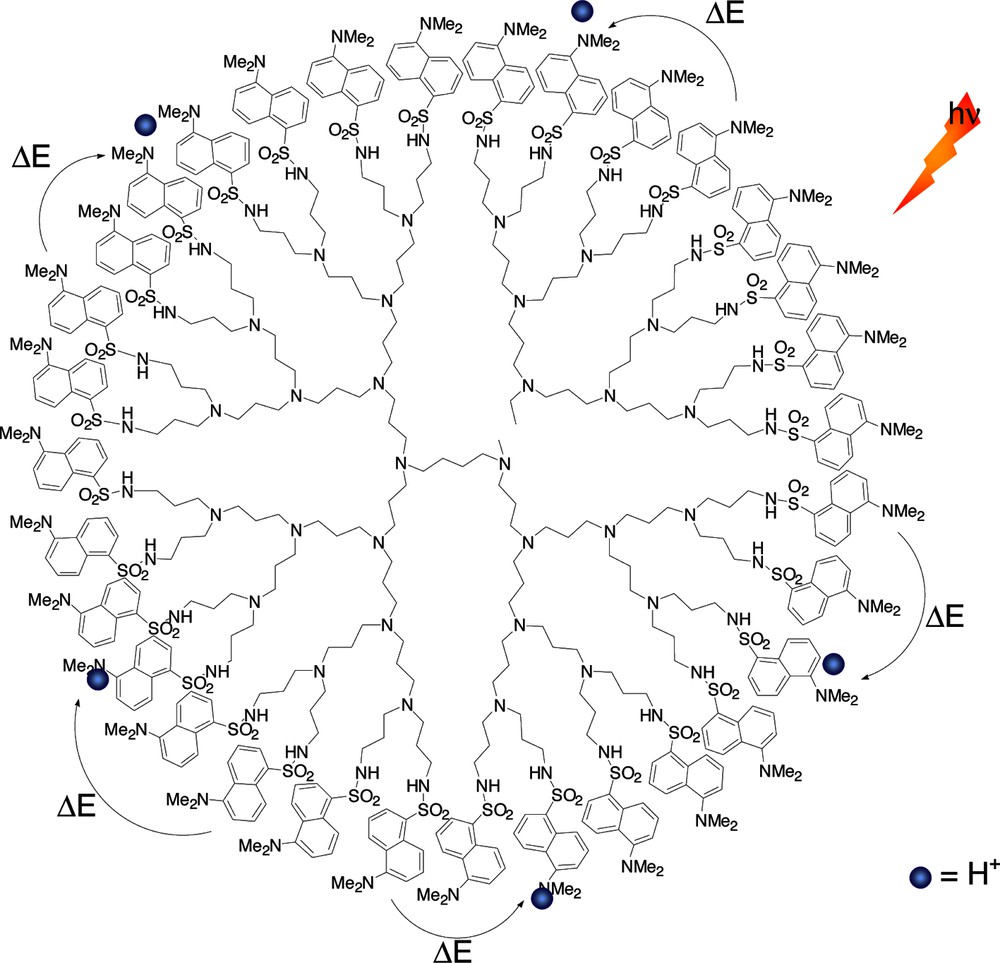
Quenching of the excited state of the non-protonated by protonated dansyl units via energy transfer at the periphery of a fourth-generation dansyl functionalized poly(propyleneamine) dendrimer [28].
In acetonitrile/dichloromethane solution each dansyl unit of the dendritic structure behaves the same as the monodansyl reference compound. The changes in the absorption and fluorescence properties upon the addition of acid showed an interesting interplay between the protonation of the tertiary amines in the core and the dansyl units at the periphery. Upon partial protonation of the dansyl moieties, energy transfer occurs from the fluorescent excited state of the non-protonated dansyl units to the ground state of the protonated ones (Fig. 9) [28].
Surprisingly, the coordination of a single Co(II) ion to the poly(propyleneamine) core resulted in the quenching of the emission of all dansyl units, rendering a very large signal amplification and thus a much larger sensitivity for Co(II) ions [29,30]. The true mechanism (electron or energy transfer) could not be revealed, since the excited state of the dansyl units is both a good energy- and electron donor, while Co(II) amine complexes have several low-energy excited states and are easily to oxidize. We believe that this represent a clear example of the role played by a dendritic structure vs a simple molecule. The complete quenching of several chromophores by a single quencher is possible only in dendrimers.
4.2 Encapsulation of fluorescent dyes
The dansyl-functionalized dendrimers could also be employed for the extraction in dichloromethane of fluorescent dyes, such as eosin Y [31,32], fluorescein [32], and rose bengal [32] from water. Once hosted in the dansyl-functionalized dendrimer, one molecule of eosin Y was found to be capable of quenching the fluorescence of all dansyl moieties at the periphery via energy transfer. The bi-exponential decay of the excited state of eosin Y encapsulated in the dendrimer was attributed to the possibility for eosin Y to occupy two different sites, closer or farther from the dansyl, within the dendrimer. Fluorescein and rose bengal show qualitatively similar behaviour as eosin Y. Furthermore, eosin Y was found to sensitize dioxygen emission via its triplet state. This sensitization process was four times more efficient in case of free eosin Y than in case of eosin Y hosted in the dendrimer, demonstrating once more the protection of the branches and the shielding from the interaction with dioxygen.
5 Hamilton receptor-functionalized dendrimers
5.1 The Hamilton receptor
A receptor containing 2,6-diaminopyridine, which is able to bind strongly barbiturates via multiple hydrogen bonds was introduced in 1988 by Hamilton et al. ((Fig. 10) [33].
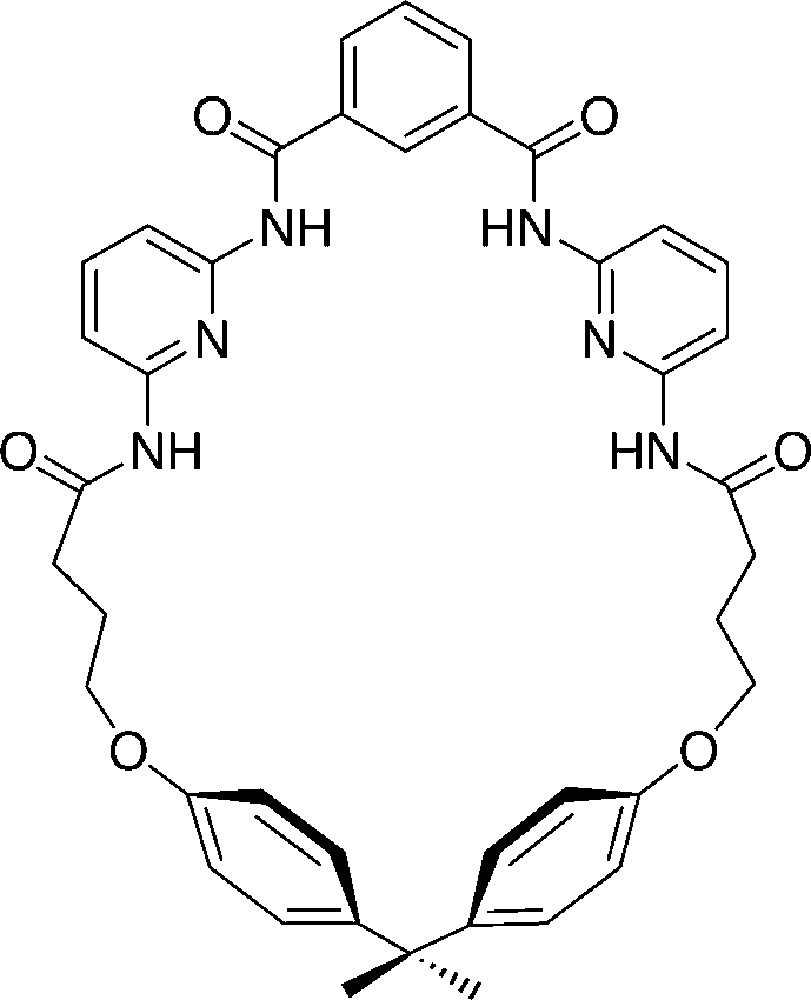
The barbiturate receptor published in 1988 by Hamilton et al. [33].
Since then several papers appeared reporting on its ability to subtract barbiturates from serum [34,35], its use as a model for enzyme catalysis [36–38], as a building block in supramolecular materials [39,40], and as a receptor in photoactive hydrogen bond-based assemblies [41,42]. From these studies, it was clear that the receptor can be a powerful tool to create stable supramolecular assemblies with barbiturates, even in solvents that are competitive for the receptor site [43].
5.2 Hamilton receptor-functionalized dendrimers
The assembly of multiple components in a predefined way in order to perform a specific function, such as photo-induced energy or electron transfer processes, is an important issue in supramolecular chemistry [42,44–55]. In general, self-assembly and molecular recognition involve the use of a mono- or bifunctionalized host or guest. Multi-binding events in artificial systems within the same molecule are very rare, especially when hydrogen bonds are used to glue the complementary components. Since dendrimers are build-up very regularly, it is in fact possible to implement receptor sites in the core, in the branches or at the periphery [19,56–66]. The creation of structures, that can bind selectively a certain class of compounds, contributes to the development of artificial binding sites closely resembling those found in proteins [67–69]. Of particular interest are host-guest systems in which the binding of a guest can be used to promote new functions, such as energy and electron transfer processes.
In this view poly(propyleneamine) dendrimers substituted at the periphery with receptors were synthesized that can bind barbiturates and its derivatives via six hydrogen bonds (‘Hamilton’ receptor). The structure of a second-generation ‘Hamilton’ receptor-functionalized dendrimer employed as a supramolecular host for barbiturates is depicted in Fig. 11 [70].

A second-generation ‘Hamilton’ receptor-functionalized poly(propyleneamine) dendrimer as a supramolecular host for barbiturates [70].
5.3 Energy transfer across hydrogen bonds
The HR dendrimers have a strong absorption and a weak emission attributed to the Hamilton receptor units. Binding of a barbiturate guest, e.g., [Re(CO)3(Br)(barbi-bpy)] (barbi-bpy = 5-[4-(4′-methyl)-2,2'-bipyridyl]methyl-2,4,6-(1H,3H,5H)-pyrimidinetrione), which has an excited state at lower energy, than the HR-dendrimers results in an energy transfer from the ‘Hamilton’ receptors to [Re(CO)3(Br)(barbi-bpy)] with a rate of 3.6 × 1010 s–1 (Fig. 12) [70].
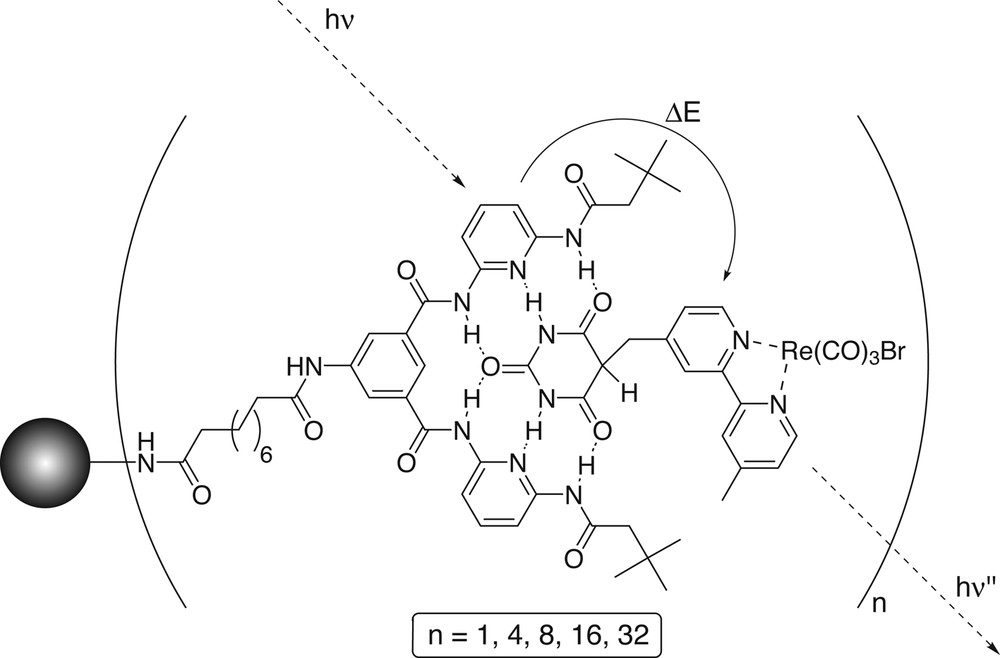
Energy transfer from the excited state of the ‘Hamilton’ receptors at the periphery to [Re(CO)3(Br)(barbi-bpy)] bound to the receptor site [70].
The emission of [Re(CO)3(Br)(barbi-bpy)] shifts towards higher energy upon binding to the HR-dendrimers as a result of deprotonation of the barbituric acid moiety attached to the bipyridine ligand. The deprotonation of the barbituric acid unit is essential to have a high binding constant between the [Re(CO)3(Br)(barbi-bpy)] and ‘Hamilton’ receptor and therefore a good electronic coupling between the two moieties. The monitoring of the photo-induced energy transfer process is an easy way to follow the assembly process. The assembly is fully reversible and addition of Barbital results in the recovery of the receptor emission and a decrease in the emission of [Re(CO)3(Br)(barbi-bpy)].
In the binding studies, the emission of the ‘Hamilton’ receptor was successfully used to probe the binding of guest molecules. As a consequence the ‘Hamilton’ receptor-functionalized dendrimers can be regarded as a supramolecular sensor for barbiturates.
Acknowledgements
We wish to thank all the co-workers that contribute to the work described and COST D11. A.D. thanks CW-NWO for financial support.