1 Introduction
Dendrimers are complex but well defined multibranched chemical compounds, with a high degree of order, and the possibility of containing selected chemical units in predetermined sites of their tree-like structure [1]. Dendrimer chemistry is a rapidly expanding field for both basic and applicative reasons [2].
From a topological viewpoint, dendrimers contain three different regions: core, branches, and surface. Another important property of dendrimers is the presence of internal cavities where ions or neutral molecules can be hosted [3]. Such a property can potentially be exploited for a variety of purposes, which include catalysis and drug delivery.
Luminescent units can be incorporated in different regions of a dendritic structure and can also be hosted in the dendrimer cavities. Coupling luminescence with the host-guest dendrimer chemistry can lead to systems capable of performing very interesting functions. In this short account, we will review some recent results obtained in our laboratories with luminescent dendrimers capable of encapsulating metal ions or organic molecules in their internal cavities.
2 Dendrimers with a luminescent metal-complex core
Our studies started with a dendrimer (12+) in which a well-known luminescent unit, [Ru(bpy)3]2+ (bpy = 2,2′-bipyridine), is not hosted, but covalently encapsulated as a core in a dendrimer whose branches contain the potentially luminescent 1,3-dimethoxybenzene- and 2-naphthyl-type chromophoric units [4]. It is well known that in the [Ru(bpy)3]2+ metal complex the lowest excited state is a metal-to-ligand charge-transfer triplet, 3MLCT, which emits a relatively strong phosphorescence (λmax = 610 nm). We observed that in dendrimer 12+ the fluorescence of the dimethoxybenzene– and naphthyl-type units is almost completely quenched in acetonitrile solution, with concomitant sensitization of the [Ru(bpy)3]2+ phosphorescence. These results show that very efficient energy-transfer processes take place converting the very short lived (nanosecond time scale) UV fluorescence of the aromatic units of the wedges to the relatively long lived (microsecond time scale) orange phosphorescence of the metal-based core. This dendrimer is therefore an excellent example of light-harvesting antenna system as well as of a species capable of changing the colour of the incident light. It should also be noted that in aerated solution the phosphorescence quantum yield of the dendritic core is more than twice intense as that of the [Ru(bpy)3]2+ parent compound because the dendrimer branches protect the Ru-bpy based core from dioxygen quenching [5].

An alternative route, followed by Fréchet, to encapsulate a luminescent metal complexes as a core in a dendrimer is self-assembly of functionalized carboxylate-core dendrons around Er3+, Tb3+, or Eu3+ ions [6]. Experiments carried out in toluene solution showed that UV excitation of the chromophoric groups contained in the branches caused the sensitized emission of the lanthanide ion. The much lower sensitization effect found for Eu3+ compared with Tb3+, ascribed to a weaker spectral overlap, could in fact be related to electron transfer quenching of the donor excited state in the case of the easy to reduce Eu3+ ion [7].
3 Luminescent dendrimers hosting metal ion quenchers
Dendrimers of the poly(propylene amine) family functionalized with fluorescent dansyl units at the periphery [8] have been used as hosts for metal ions. Each dendrimer nD, where the generation number n goes from 1 to 5, comprises 2n+1 (i. e., 64 for 5D) dansyl functions in the periphery and (2n+1 – 2) (i.e., 62 for 5D) tertiary amine units in the interior. Compound 2 represents the fourth generation dendrimer 4D. These dendrimers show intense absorption bands in the near UV spectral region (λmax = 252 and 339 nm; εmax = 12 000 and 3900 l mol–1 cm–1, respectively, for each dansyl unit) and a strong fluorescence band in the visible region (λmax = 500 nm; Φem = 0.46, τ = 16 ns).
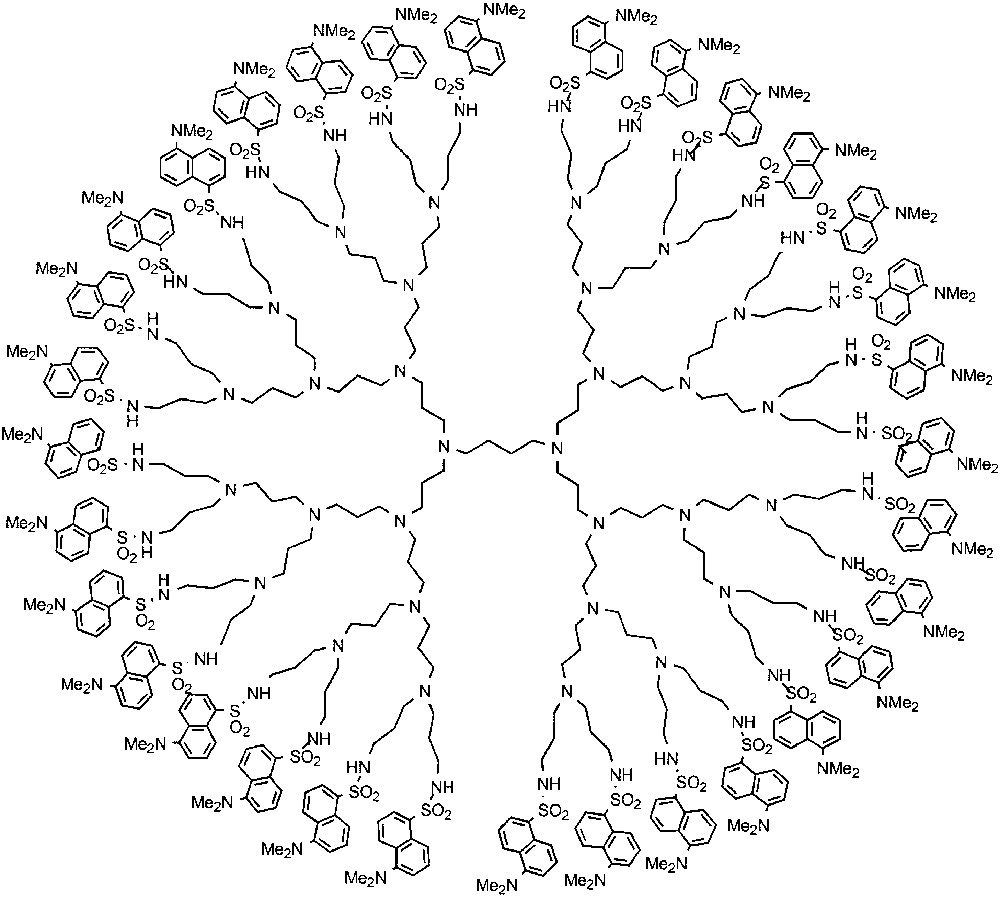
These dendrimers can coordinate metal ions by the aliphatic amine units contained in the interior of the dendrimer [9] and can be used as fluorescent sensors for metal ions, such as Co2+, capable to quench the dansyl fluorescence. The advantage of a dendrimer for this kind of application is related to the fact that a single analyte can interact with a great number of fluorescent units, which results in signal amplification. For example, when a Co2+ ion enters in dendrimer 2, the fluorescence of all the 32 dansyl units is quenched, with a 32 times increase in sensitivity with respect to a conventional dansyl sensor. This concept is illustrated in Fig. 1.
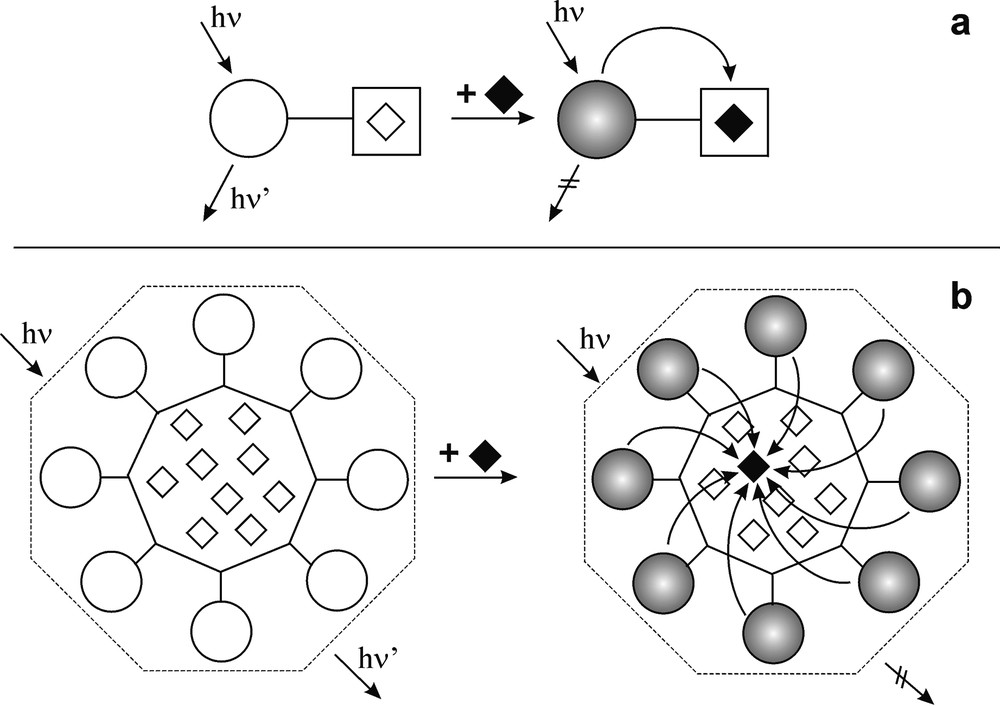
Schematic representation of (a) a conventional fluorescent sensor and (b) a fluorescent sensor with signal amplification. Open rhombi indicate coordination sites and black rhombi indicate metal ions. The curved arrows represent quenching processes. In the case of a dendrimer, the absorbed photon excites a single fluorophore component, i.e. quenched by the metal ion, regardless of its position.
4 A luminescent dendrimer hosting luminescent metal ions
Dendrimer 3 is quite interesting since it contains in the interior 18 amide groups, which are known to strongly coordinate lanthanide ions, and in the periphery 24 chromophoric dansyl units that, as mentioned above, show intense absorption bands in the near UV spectral region and an intense fluorescence band in the visible region. We have investigated [10] the complexation of dendrimer 3 by six lanthanide ions (Gd3+, Tb3+,Yb3+, Nd3+, Eu3+, Er3+) characterized by very different energy-level patterns (Fig. 2) and capable of exhibiting their own luminescence. Among these ions, Eu3+ and Yb3+ are rather easy to reduce (E0 = –0.35 and –1.1 V, respectively, for the aqua ions, vs standard hydrogen electrode), whereas none of them can be easily oxidized.
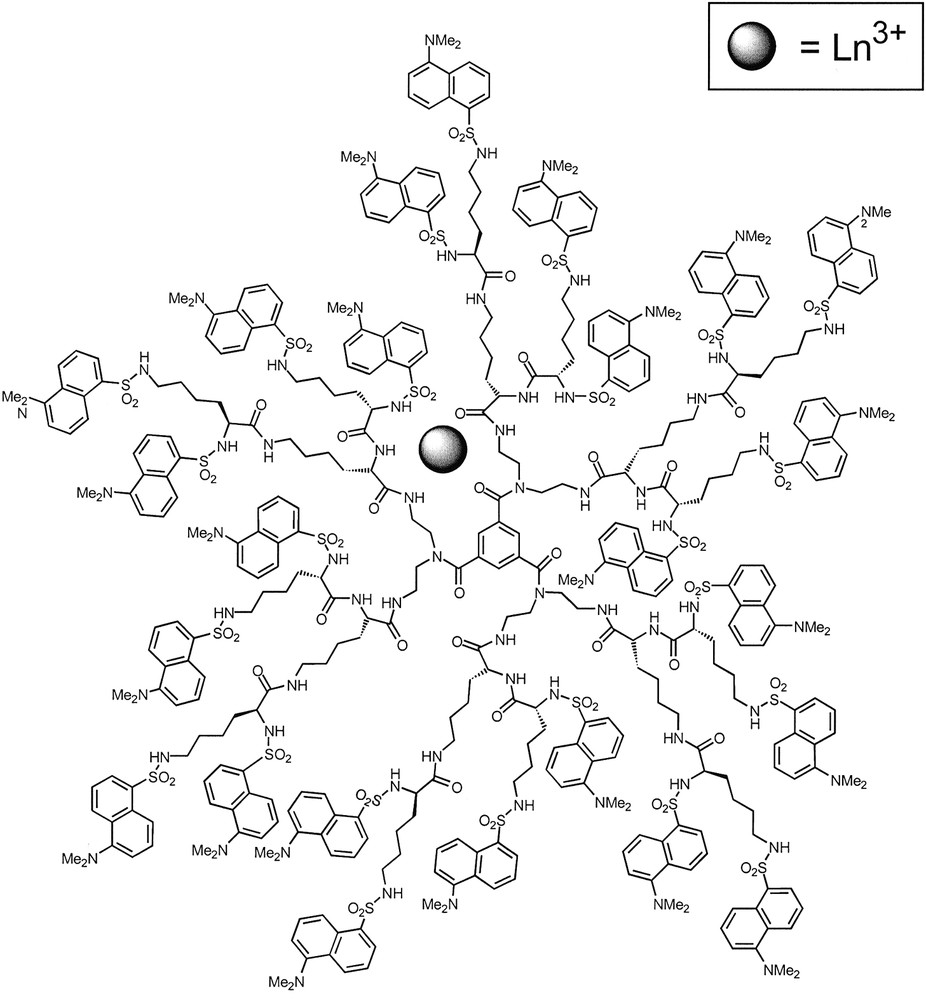
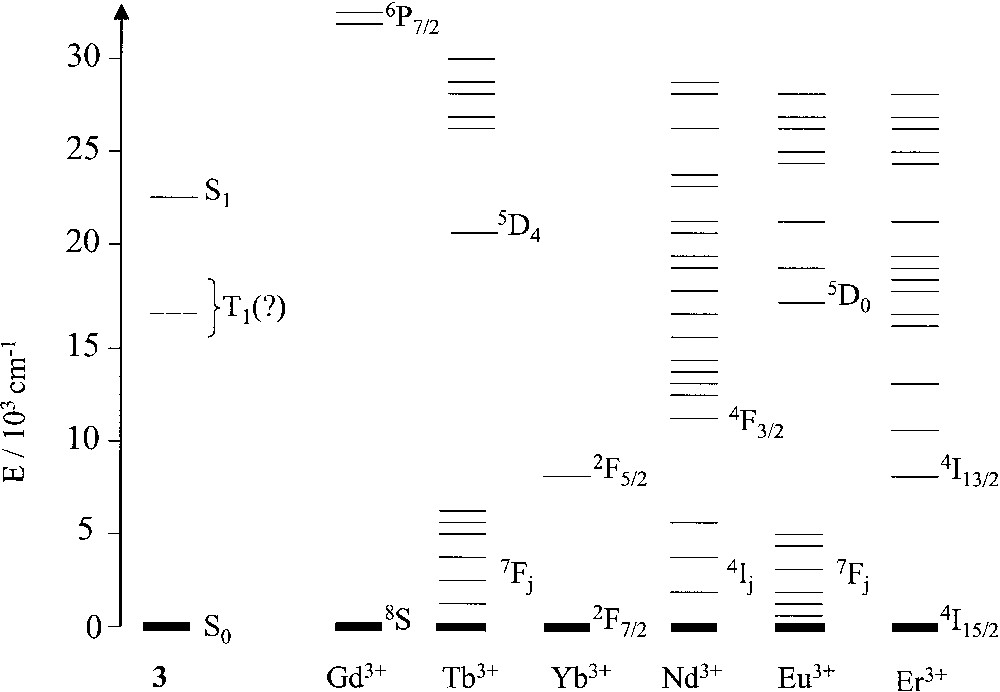
Energy-level diagrams for the dansyl units of dendrimer 3 and the investigated lanthanide ions. The position of the triplet excited state of 3 is uncertain because no phosphorescence can be observed.
Formation of the metal complexes [Ln3+⊂3] is accompanied by a quenching of the fluorescent excited state of the dansyl units. The quenching effect is very large for Nd3+ and Eu3+, moderate for Er3+ and Yb3+, small for Tb3+, and very small for Gd3+.
In the case of [Gd3+⊂3], neither energy– nor electron-transfer quenching of the fluorescent dansyl excited state S1 are allowed. The very small quenching effect can be assigned either to induced intersystem crossing or to a charge perturbation on the fluorescent dansyl excited state. In the first case, the triplet excited state of the dansyl unit (T1) should be formed and, in rigid matrix at 77 K, the phosphorescence of T1 should be observed. The emission spectrum recorded under such conditions, however, showed no evidence of phosphorescence. In any case, the results obtained for Gd3+ show that the much larger quenching effects observed for other lanthanide ions cannot be due to induced intersystem crossing of the S1 excited state of dansyl.
For [Nd3+⊂3], quenching by electron transfer of the dansyl fluorescence is thermodynamically forbidden. The strong quenching effect observed is accompanied by the sensitized near infrared (NIR) emission of the 4F3/2 excited state of the metal ion. Energy transfer by a Förster-type mechanism is efficient because of the extensive overlap between the dansyl emission band and the energy levels of Nd3+.
In the case of [Eu3+⊂3], both energy and electron transfer quenching are thermodynamically allowed. At room temperature, the strong quenching on the dansyl fluorescence is not accompanied by the sensitized emission of the metal ion. This result is consistent with the fact that the lowest excited state of the system is an electron-transfer level, [Eu2+⊂3+]. Therefore, either an electron-transfer quenching process leads directly to this non emissive level, or energy transfer to the luminescent 5D0 metal-centred level is followed by an electron-transfer process, faster than the 5D0 emission, leading to the [Eu2+⊂3+] level. In rigid matrix at 77 K, i.e. under conditions in which electron-transfer processes are disfavoured, the quenching of the dansyl fluorescence is accompanied by the sensitized emission of the Eu3+ ion in the visible region, as expected for an energy-transfer quenching mechanism.
For [Tb3+⊂3], the small quenching effect is assigned to energy transfer.
In the case of [Er3+⊂3], electron-transfer quenching is not allowed. The moderate quenching effect is accompanied by the sensitized emission of the 4I13/2 excited state of the metal ion (1525 nm). Therefore, the most important quenching mechanism is energy transfer from the S1 excited sate of the dansyl units to a low-density isoenergetic manifold of metal ion excited states.
The case of [Yb3+⊂3] is very interesting. A moderate quenching effect is accompanied by the sensitized emission of the 2F5/2 Yb3+ level (Fig. 2). The interpretation of this result is not straightforward. In fact, whereas the moderate quenching ability on the dansyl fluorescence could be assigned to an electron-transfer quenching mechanism, which is thermodynamically allowed, the sensitization of the 2F5/2 emission is an unexpected result since energy transfer from the S1 excited state of the dansyl unit appears to be unlikely because of the lack of spectral overlap. It should be noted, however, that for [Yb3+⊂3], contrary to what happens in the case of [Eu3+⊂3], the [Yb2+⊂3+] electron transfer level lies above the luminescent metal-centred level (Fig. 3a). Therefore, quenching by electron transfer can be followed by a back electron transfer leading to the formation of the lower lying, luminescent 2F5/2 level rather than the 2F7/2 ground state. In rigid matrix at 77 K, i.e. under conditions in which the [Yb2+⊂3+] electron transfer level moves to much higher energy, the sensitized emission of the 2F5/2 Yb3+ level can no longer be observed, confirming that the low energy 2F5/2 level cannot be populated by direct energy transfer from the S1 excited state of the dansyl unit. On protonation of the dansyl units, the electron-transfer quenching of the T1 excited state is prevented for thermodynamic reasons. On the other hand, there is no overlap between the phosphorescence band of protonated dansyl and the only available 2F5/2 excited state of Yb3+ (Fig. 3b). Therefore, energy transfer cannot occur. Accordingly, at 77 K, the phosphorescence band of protonated dansyl is not quenched and the near-infrared emission of Yb3+ is not sensitized.
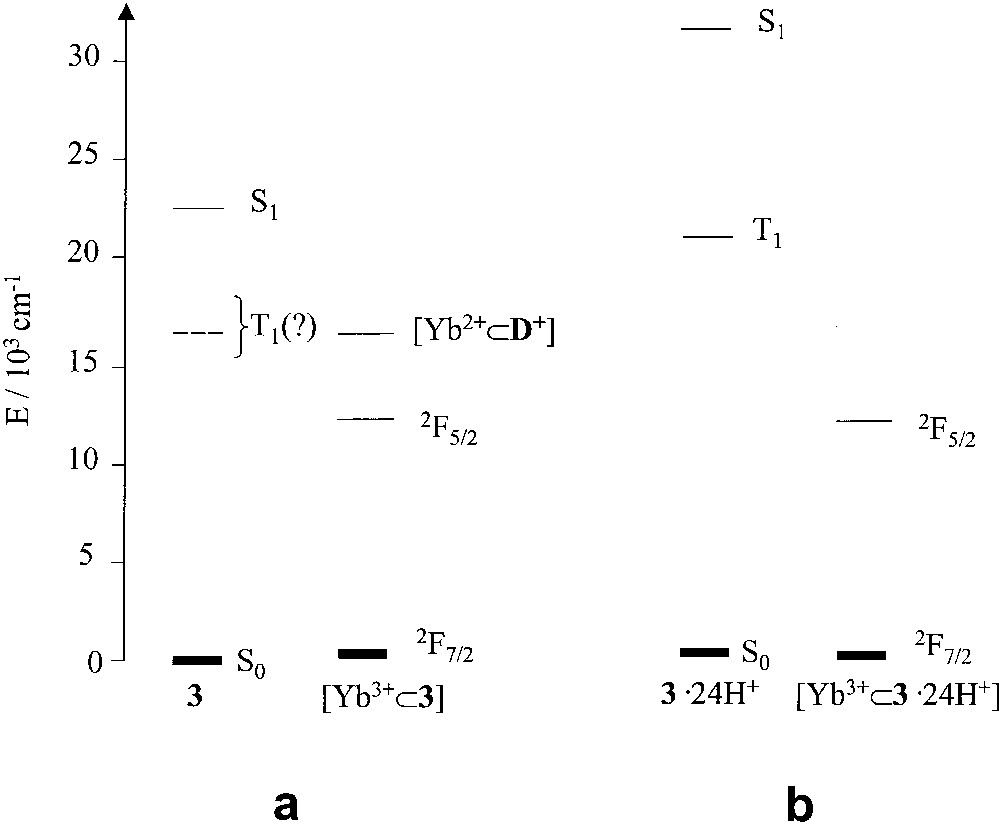
Energy level diagrams for 3 and [Yb3+⊂3] (a); 3.24H+ and [Yb3+⊂3.24H+] (b). Notice the presence of a low energy [Yb2+⊂3+] electron transfer level for [Yb3+⊂3].
5 Luminescent dendrimers hosting luminescent dyes
The above-mentioned nD dendrimers of the poly(propylene amine) family functionalized with fluorescent dansyl units at the periphery (e.g., the fourth generation compound 2) have also been used as hosts for fluorescent dye molecules [11]. In dichloromethane solution, the nD dendrimers extract eosin from aqueous solutions with the maximum number of eosin molecules hosted in the dendrimers increasing with increasing dendrimer generation, up to a maximum of 12 for the 5D dendrimer. The fluorescence of the peripheral dansyl units of the dendrimers is completely quenched via energy transfer (Förster mechanism) by the hosted eosin molecule, whose fluorescence (λmax = 555 nm) is, accordingly, sensitized. The behaviour of fluorescein and rose bengal is qualitatively similar to that of eosin, whereas naphthofluorescein is not extracted. Quantitative analysis of the results obtained has shown that a single eosin guest is sufficient to quench completely the fluorescence of any excited dansyl unit of the hosting dendrimer. Fluorescence lifetime measurements indicated that the dye molecules occupy two different sites (or two family of substantially different sites) in the interior of the dendritic structure.
Dendrimer 4 consists of a hexaamine core surrounded by 8 dansyl–, 24 dimethoxybenzene–, and 32 naphthalene-type units [12]. In dichloromethane solution, 4 exhibits the characteristic absorption bands of the component units and a strong dansyl-type fluorescence. Energy transfer from the peripheral dimethoxybenzene and naphthalene units to the fluorescent dansyl units occur with >90% efficiency. When the dendrimer hosts a molecule of the fluorescent eosin dye (4⊃eosin), the dansyl fluorescence, in its turn, is quenched and sensitization of the fluorescence of the eosin guest can be observed. Quantitative measurements showed that the encapsulated eosin molecule collects electronic energy from all the 64 chromophoric units of the dendrimer with an efficiency > 80%. Both intramolecular (i.e., within dendrimer) and intermolecular (i.e., dendrimer host → eosin guest) energy-transfer processes occur very efficiently by a Förster type mechanism because of the strong overlap between the emission and absorption spectra of the relevant donor/acceptor units.
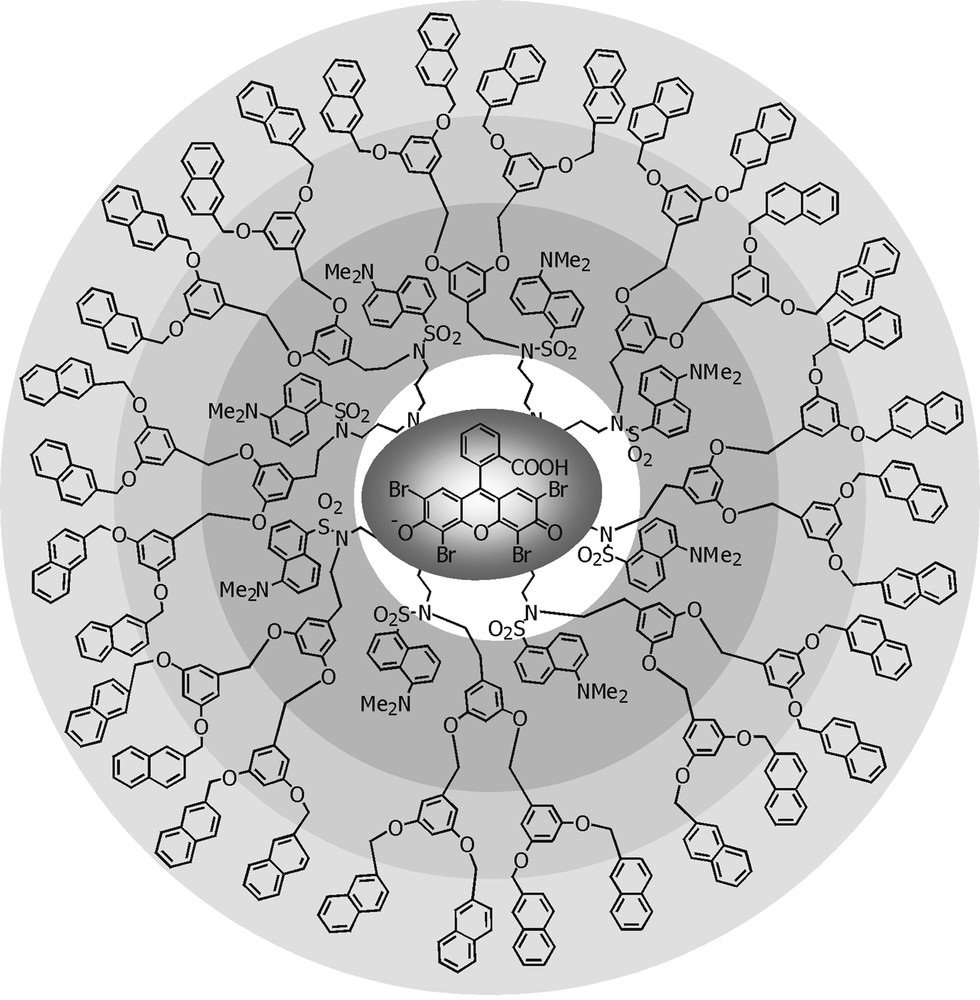
6 Conclusions
Many dendrimers exhibit excellent hosting ability towards ions or molecules. When the hosting dendrimer and/or the guest ion or molecule are luminescent, quenching/sensitization processes may occur that can be exploited for several purposes such as sensing with signal amplification, light harvesting, and changing the incident light into an emitted light of different colour. An advantage shown by such host-guest systems compared with dendrimers with a luminescent core is that the wavelength of the sensitized emission can be tuned by simply changing the guest hosted in the same dendrimer.
Acknowledgements
We would like to thank our colleagues and co-workers, whose names appear in the quoted references, for their contribution to the research described in this account. This work has been supported in Italy by MIUR (Supramolecular Devices Project) and EC (HPRN-CT-2000-00029), and in Germany by the European IST programme LIMM (Light-Induced Molecular Movements; Contract number IST-2001-35503).