1 Introduction
Miniemulsion technology has begun to gather a significant amount of attention over the course of the past few years for a number of reasons, but particularly because of the way the particles are formed: submicron droplets are generated by combining a judicious choice of stabilization system with mechanical agitation. In this manner, it is relatively easy to control the number of particles in the system before the reaction begins. In addition, this means of generating droplets that are directly polymerised is very practical for applications such as encapsulation, and the production of complex materials. Excellent reviews of the processes underlying miniemulsion polymerisation are available in the literature [1,2], and the reader is referred to these works for further discussions on miniemulsions.
The improvement of polymer products and polymerisation processes is a driving force for the research and development of different techniques for polymerisation monitoring. A major disadvantage of conventional techniques for conversion and composition monitoring such as gravimetry and 1H-NMR spectroscopy is the time lag between sampling and results. Despite their accuracy, these techniques can rarely be used for real-time process monitoring and control. Spectroscopic techniques such as mid- and near-infrared, and Raman spectroscopy are especially suitable for real-time reaction monitoring [3]. They can provide structural and kinetic information without costly modifications to existing process equipment. In addition, remote sensing probes, connected to the spectrometer and controlled by electronic modules, are easily inserted into the reactor. In the past, Attenuated Total Reflectance–Fourier Transform Infrared (ATR–FTIR) spectroscopy was mainly used for investigation of solids, liquids and thin films [4]. Recent technological advancements such as diamond-composite technology for sensor elements and light conduit technology have enabled the use of ATR–FTIR spectroscopy for real-time, in situ polymerisation monitoring. Commercially available reaction monitoring systems have been employed for monitoring of conversion and composition in a variety of polymerisations in solution [5,6], emulsion [7,8], and microemulsion [9]. They have also been used for living cationic polymerisation monitoring [10,11]. On the other hand, to the best of our knowledge there are no studies evaluating the performance of ATR–FTIR spectroscopy for the on-line monitoring of miniemulsion polymerisation.
Matsumoto et al. [12] employed the ReactIR™ 1000 to investigate BMA homopolymerisation and BMA/1,6 hexanediol dimethacrylate copolymerisation in emulsion. The absorption of backbone methyl group in the poly(BMA) at 1241 cm–1 was used for monitoring conversion.
In a previous paper [13], an interesting stabilization system for the polymerisation of styrene and of butyl methacrylate (BMA) in miniemulsion was presented, and the authors evaluated the kinetics of homopolymerisations. In addition, dynamic scanning calorimetry (DSC) analyses suggested that miniemulsions were compartmentalized systems (i.e. there was no mass transfer between growing droplets). However, the proof provided by DSC is indirect, and it was decided that it would be useful to use an on-line technique to provide more detailed and direct information on the evolution of polymer composition in the reactor. It was also desired to find an on-line tool that would allow us to analyse the kinetics in systems with different droplet compositions. For this reason, it was decided that ATR–FTIR spectroscopy would be used to follow the polymerisations in real time. To do so, three different types of polymerisations were carried out: (i) separate styrene and BMA homopolymerisations; (ii) blends of miniemulsions of styrene and of BMA simultaneously; and (iii) a miniemulsion copolymerisation of the same two monomers. All polymerisations were performed in a reactor equipped with an ATR–FTIR probe that collected the IR spectra during the course of the reaction (ReactIR™ 1000 ASI Applied Systems, Inc., Mettler-Toledo). The underlying idea is that styrene and BMA miniemulsion homopolymerisations are different in term of their kinetics from the copolymerisation of the same two monomers, and that the three resulting polymers (two homopolymers and a copolymer) are different in terms of their chemical structures. Thus, if there is no significant monomer transfer between the droplets during polymerisation, the spectra of the blend reaction should simply be the sum of the spectra from the homopolymerisation. The spectra of all the miniemulsions polymerisations were studied to determine the assignment of all the characteristic peaks, and these results allowed us to draw further conclusions about the compartmentalization in miniemulsion polymerisation.
2 Experimental
All chemicals were used as supplied. SDS (EM Science), KPS (Aldrich), stearyl methacrylate – SMA – (Aldrich), and Triton X-405 (Aldrich) were used without further purification. Distilled de-ionised (DDI) water was used for all experiments. Experimental conditions are summarized in Table 1.
Experimental conditions
Styrene | MBA | SMA | SDS | Triton | KPS | |
(% wt/total) | (% wt/total) | (% wt/monomer) | (× CMC) | (× CMC) | (% wt/total) | |
M1 | 38 | — | 2 | 0.5 | 8 | 0.03 |
M2 | — | 38 | 2 | 0.5 | 8 | 0.03 |
M3 | 19 | 19 | 2 | 0.5 | 8 | 0.03 |
M4 | 19 | 19 | 2 | 0.5 | 8 | 0.03 |
One day prior to polymerisation, the following procedure was used for the preparation of the miniemulsion. The aqueous phase (SDS, Triton X-405, and DDI water) and the oil phase (monomer(s) and SMA) were prepared in separate flasks using a magnetic stirrer. After dissolving, both phases were mixed for an hour using a magnetic stirrer. The reaction mixture was homogenized using a 550 Sonic Dismembrator (Fisher Scientific). Using a pulsed mode, the reaction mixture was sonified for 4 min at Level 9. During sonification, the mixture was continuously stirred using a magnetic stirrer and cooled using an ice bath. After the homogenisation, the miniemulsions were stored in a refrigerator at +5 °C.
All polymerisation reactions were performed in an automated 1.2-l glass reactor (LabMax®, Mettler-Toledo). The reactor was equipped with ports for feeding, sampling, nitrogen, and with a condenser. An anchor stirrer was used for mixing. The reaction temperature (70 °C), stirring speed (200 rpm) and dosing were controlled by Camille® software Mettler-Toledo). At various times, a sample was collected from the reactor for off-line analysis. The samples were placed immediately in an ice-water bath to quench the reaction. Conversion was measured off-line using gravimetry.
All polymerisations were monitored in-line using the ReactIR™ 1000 (ASI Applied Systems, Inc., Mettler-Toledo). This reaction monitoring system has been previously described [7,8]. The ATR–FTIR probe was inserted through a port in the reactor head. The position of the probe was approximately 2 mm from the stirrer. For an optimal signal-to-noise ratio, the number of scans and resolution were set to 128 and 16, respectively. In all reactions, a background spectrum and the reaction spectra were collected using these conditions. During the course of the reaction, spectra were collected every two minutes.
The following procedure was employed in all experimental runs. A known amount of previously homogenized mixture was placed into the reactor. In the case of miniemulsion blending, an appropriate amount of homogenized mixtures containing Sty and BMA monomers were mixed. A container with the initiator solution was placed onto an automatic balance for feeding into the reactor. After the ATR–FTIR probe was inserted and secured, the reactor content was purged for 15 minutes using nitrogen. Next, the reactor content was heated to 70 °C. When the temperature was stabilized, a background spectrum was collected. The addition of the initiator solution and the collection of reaction spectra were started simultaneously.
3 Results and discussion
The miniemulsion polymerisation recipes are summarized in Table 1. In run M3, the BMA and styrene miniemulsions were prepared separately. The two populations of droplets were then introduced into the same reactor and polymerised at the same time. In this experiment the reactor contents contained 50% (w/w) of BMA droplets and 50% styrene droplets. On the other hand, in run M4, styrene and BMA were mixed together to make a 50:50 (w/w) mixture of monomers. This mixture was then homogenized so that the resulting droplets were composed of 50 % of the respective monomers by weight.
Fig. 1 shows a collection of typical ATR–FTIR spectra obtained for styrene miniemulsion polymerisation. Fig. 2 shows the spectra for the unpolymerised miniemulsion (i.e. dispersions of droplets) and the spectra of the final latex at the end of the reaction for styrene, and Fig. 3 shows the same for BMA. As shown by the data in Table 2, and confirmed in Fig. 2 and 3, the characteristic peaks of the homopolymers are significantly different. Based on the assembly of the spectra collected as a function of time, the characteristic peak assignments were determined.

ATR–FTIR spectra of styrene miniemulsion polymerisation (M1), T = 70 °C.
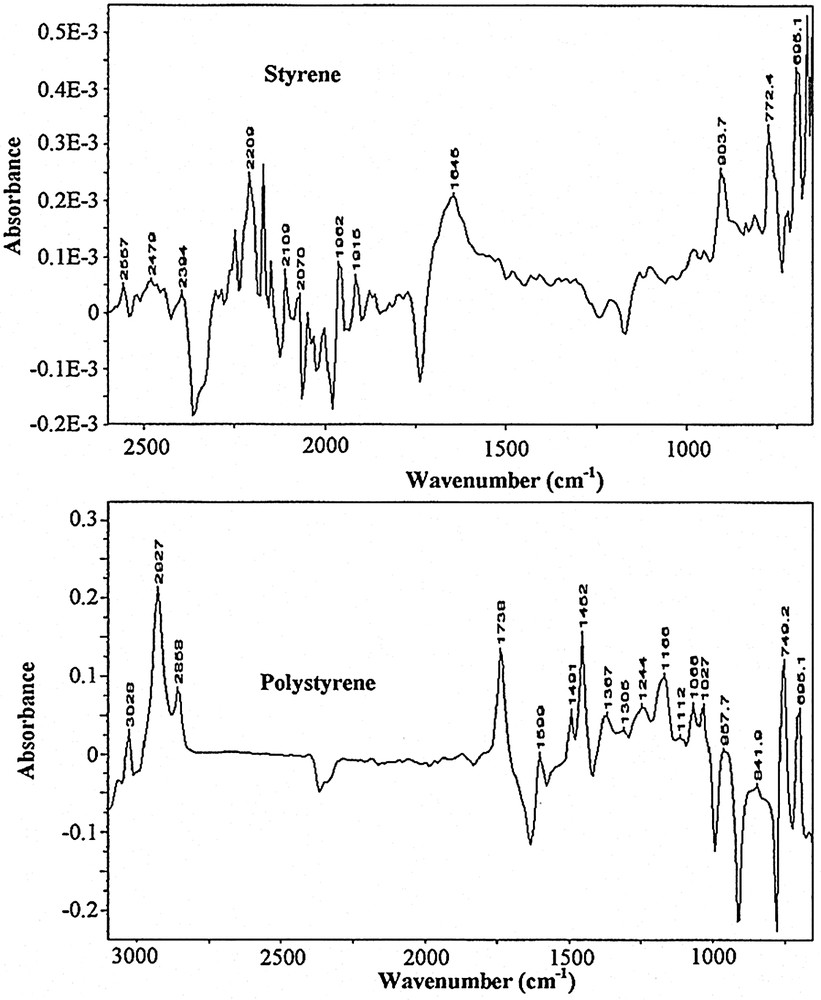
ATR–FTIR spectra of the unpolymerised (top) and polymerised (bottom) miniemulsions of styrene. As expected, the peak structure is quite different for the two monomers, and allows us to follow the disappearance of the different species relatively easily.
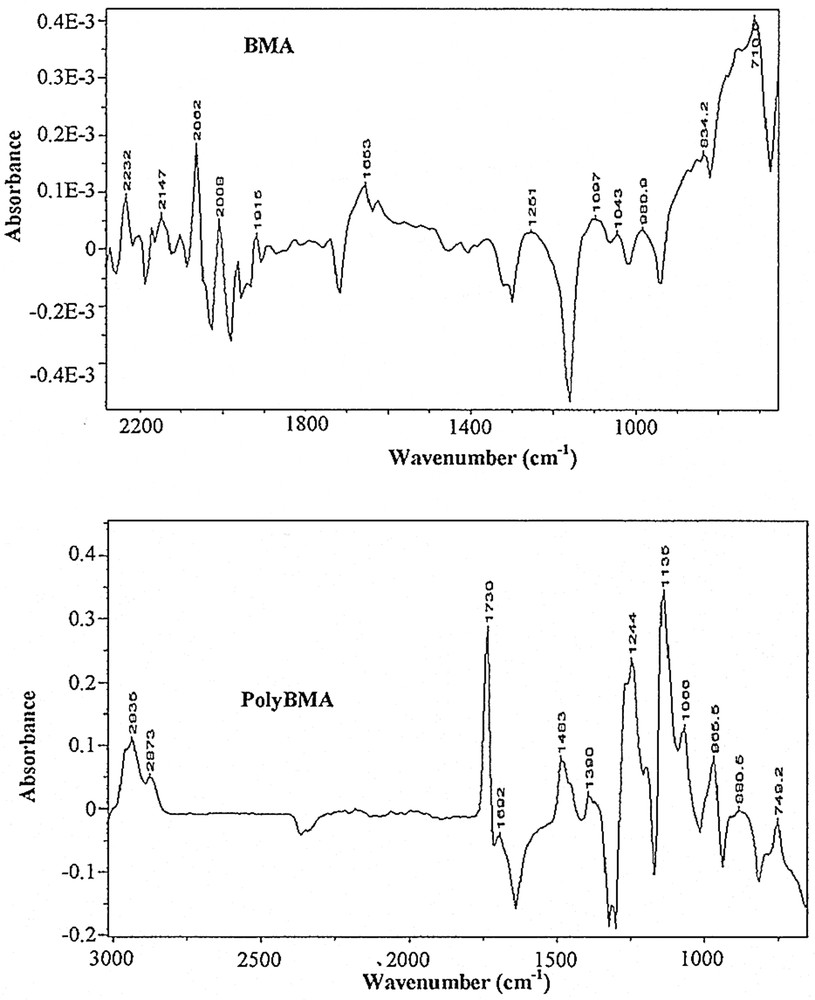
ATR–FTIR spectra of the unpolymerised (top) and polymerised (bottom) miniemulsions of BMA.
Characteristic peaks assignments for styrene (left) and BMA (right) systems
Styrene | BMA | ||
Wave number (cm–1) | Peak assignment | Wave number (cm–1) | Peak assignment |
3028 | ring –CH stretching | 2958 | CH2 asymmetric stretching |
2927 | –CH2 asymmetric stretching | 2881 | –CH3 symmetric stretching |
2842 | –CH2 symmetric stretching | 1722 | C=O stretching |
1599 | ring quadrant stretching | 1460 | –CH2 deformation |
1491 | ring semicircle stretching | 1320 | C–C–C–O stretching |
1452 | ring semicircle stretching | 1298 | C–C–C–O stretching |
1375 | –CH–CH2 wag | 1244 | C–O stretching |
1166 | –CH–CH2 wag | 1159 | C–(C=O)–O stretching |
1074 | ring semicircle stretching | 1066 | O–CH2–C asymmetric stretching |
1027 | ring semicircle stretching | 942 | –CH2 wag |
911 | ? | 749 | –CH2 wag |
757 | ring C–H wag | ||
695 | ring bending |
The conversion vs. time data were obtained off-line by following the evolution of certain characteristic polymer peak areas as a function of time. There is a clear linear relationship between the conversion and the peak area. This linear relationship has been reported by several authors [4,14]. A quantitative estimate of the conversion can be obtained by calculating the ratio of the peak area of characteristic bands (after baseline correction), according to:
(1) |
Absorbance bands such as those at 1452 cm–1 representing the ring semicircle stretching for PS, or at 2880 cm–1 for the –CH3 symmetric stretching of PBMA were found to be suitable to follow the polymerization conversions. As can be seen in Fig. 4 , the conversion obtained with the IR data agreed well with those from off-line gravimetric measurements. These results are primarily intended to show that the characteristic peaks cited are good measures of the amounts of the relative monomers in the system. We will not discuss the estimation of conversion further in this work as it will be presented in detail in a forthcoming paper from one of the research groups (U of O).
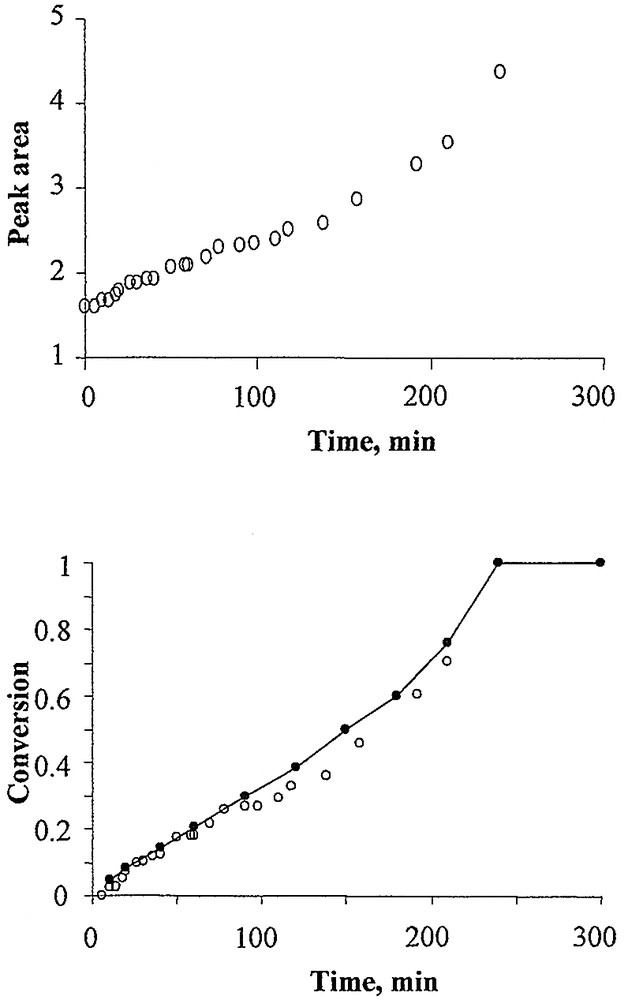
Top: evolution of peak area at 1452 cm–1 for the run M1. Bottom: conversion vs. time for the run M1. Results for M2 very similar, but not shown for the sake of brevity.
Looking now at a comparison of the miniemulsion systems containing two monomer species, recall that in run M3, the reactor contained a blend of two separate droplet phases, whereas in M4, the reaction mixture was composed of uniform droplets that were made up of 50% by weight of each monomer. In the event that mass transfer occurred between the different particles in M3, peaks corresponding to the formation of a copolymer species should be evident. In this eventuality, the copolymer peak would not necessarily be found at exactly the same position as in M4, given that we cannot guarantee that the composition in the particles in M3 would be the same as in M4. Nevertheless, as shall be seen below, no copolymer peaks were formed in M3.
By analysing the characteristic peaks obtained for runs M3 and M4 (see Table 3 and Fig. 5 ), some important differences can be observed:
- • the ring semicircle stretching of PS at 1491 cm–1 and 1452 cm–1 are present in the spectra of the ‘blends’ (M3), whereas only the peak at 1452 cm–1 is observed in the spectra of the copolymer;
- • the C–C–C–O stretching of PBMA at 1320 cm–1 and 1298 cm–1 is present in run M3, whereas the copolymer spectra show nothing in this wavelength interval;
- • new peaks (1236 cm–1, 1035 cm–1) are observed in the copolymer spectra (M4) without clear assignment; the peak at 1236 cm–1 could be attributed to the PMBA peak shift at 1244 cm–1; that at 1035 cm–1 could be attributed to the shift of the peak at 1020 cm–1, which corresponds to the ring semicircle stretching of PS; however, the accuracy of this assignment is not clear.
Characteristic peak assignments for runs M3 and M4
Wave number (cm–1) | Peak assignment | Wave number (cm–1) | Peak assignment |
2966 | –CH3 asymmetric stretching (PBMA) | 2958 | –CH2 asymmetric stretching (PBMA) |
2881 | –CH3 symmetric stretching (PBMA) | 2873 | –CH3 symmetric stretching (PBMA) |
1722 | C=O stretching (PBMA) | 1738 | C=O stretching (PBMA) |
1491 | ring semicircle stretching (PS) | 1452 | ring semicircle stretching (PS) |
1452 | ring semicircle stretching (PS) | 1375 | –CH–CH2 wag (PS) |
1383 | –CH–CH2 wag (PS) | 1236 | |
1320 | C–C–C–O stretching (PBMA) | 1166 | –CH–CH2 wag (PS) |
1298 | C–C–C–O stretching (PBMA) | 1066 | ring semicircle stretching (PS) |
1166 | –CH–CH2 wag (PS) | 1035 | |
1066 | ring semicircle stretching (PS) | 965 | –CH2 wag (PBMA) |
1020 | ring semicircle stretching (PS) | ||
942 | –CH2 wag (PBMA) | ||
911 | ? observed in M1 |
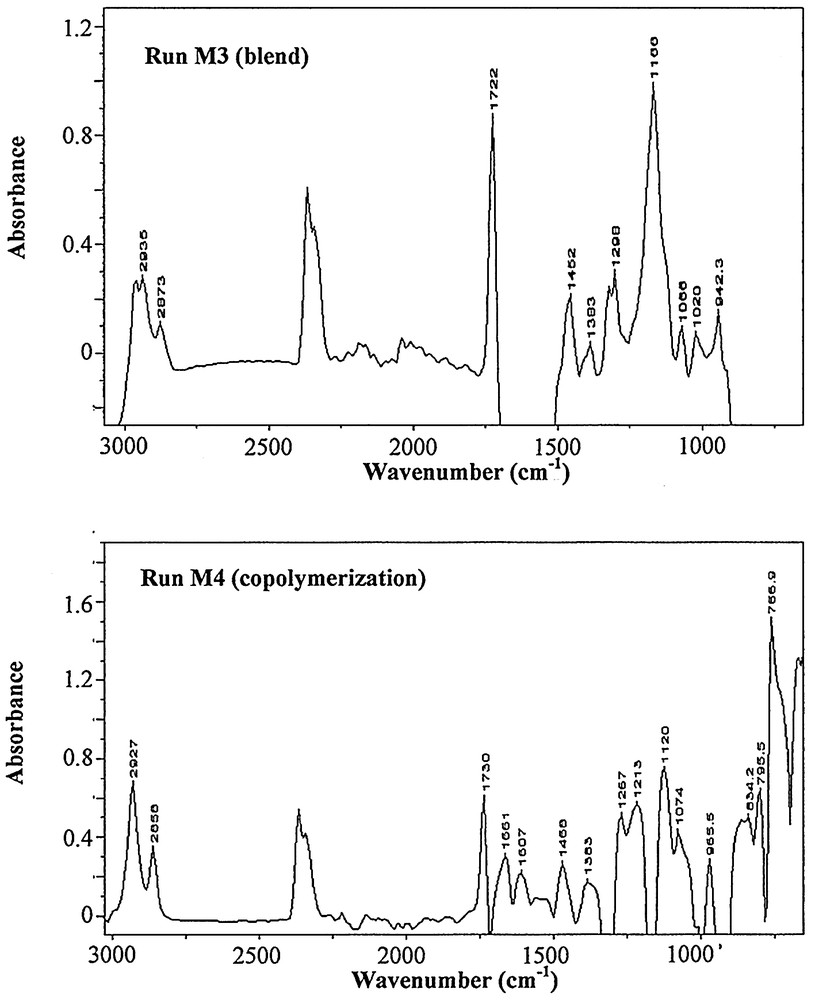
Final IR spectra for runs M3 (top) and M4 (bottom). It is clear that the structure of the spectra of the blends and copolymer are significantly different.
A comparison of the peak assignments shows that the polymer produced during the run M3 has the same chemical structure as the two homopolymers produced during runs M1 and M2. In fact, the spectrum from the end of run M3 is essentially the sum of the spectra obtained for runs M1 and M2. This, combined with the fact that the copolymer spectra differ significantly from the blended miniemulsion spectra, confirms that negligible mass transfer occurred between the droplets of styrene and BMA.
4 Conclusions
The work presented in this paper was intended to demonstrate the compartmentalization of the polymerizing droplets. Monitoring of miniemulsion polymerisation with ATR–FTIR spectroscopy allowed us to identify characteristic peaks for the different species involved (styrene, PS, BMS, PBMA, and the copolymer of styrene and BMA). The peak assignments clearly revealed that the polymer obtained with the blending of the two miniemulsions prepared separately (styrene miniemulsion + BMA miniemulsion), but polymerised together had the same characteristic peaks as the homopolymers. Different peak assignments were obtained for the copolymer with respect to the homopolymer spectra. These results clearly illustrate that negligible mass transfer occurred between the styrene and the BMA droplets. This opens up some very interesting potential applications of miniemulsion in different areas such as high solids content latexes, multimodal molecular weight distributions and particles with structured morphologies. These topics will also be the subject of a future publication.
Acknowledgements
The authors gratefully acknowledge the financial support of the France–Canada Research Fund, granted by the ‘Ambassade de France au Canada’.