1 Introduction
The increase in the solvation of trivalent lanthanides is one of the consequences of lanthanide contractions as one moves along the series. Indeed, the decrease in the ionic radius results in an increase in the charge density and greater polarity of these cations, which produces an augmentation of the number of solvent molecules in the secondary sphere of coordination. The lanthanides are, therefore, quite sensitive towards the water contents of the medium and the aqueous ions M3+ are hydrolysed in water according to (Eq. (1)):
(1) |
It may be noted that for all equilibriums studied, the hydration water molecules have been neglected and the following simple notation has been adopted (Eq. (2)):
(2) |
(3) |
On the other hand (Eq. (4)):
(4) |
1.1 Lanthanides
Biedermann et al. [1] studied the hydrolysis equilibrium of the lanthanide (III) ions. On the basis of pH measurements at constant ionic strength, maintained at 3 mol dm–3 by LiClO4, they have reported weak hydrolysis in the pH range of 6.5–8.1 and the formation of two species namely, La(OH)2+ (log β10–1 = –10.1) and La6(OH)108+ (log β60–10 = –78,7).
Yttrium mainly yields M(OH)2+ and to a lesser degree the M2(OH)24+ ions for Ce3+. However, only about 1% of the metal ion is hydrolysed without precipitate formation and consequently the main equilibrium appears to be [2]:
In aqueous solutions, the hydrolysis of trivalent lanthanides begins at a pH as low as 6. For example, for europium, log β10–1 varies between –7.8 at I = 0.1 mol dm–3 and –8.1 at I = 1 mol dm–3) [3]. At the same time, various species can be formed, such as Ln(OH)2+, Ln(OH)3, Ln(OH)4–, Ln2(OH)24+, Ln3(OH)54+.
Schwinte et al. [4], under identical experimental conditions as in this work, obtained the methanolysis constants of some lanthanide ions. These results will be compared to the hydrolysis constants obtained in this work.
The results of references [3] and [4] appear to be quite credible, as they are the only studies carried out at constant ionic strength. It may be noticed that in the last years few results concerning the hydrolysis of lanthanide ions have been reported. This is certainly due to the complexity of models and to the difficulties met in this system.
The hydrolysis of trivalent lanthanides is gaining importance because of the increasing interest in the biological aspects of their complexes [5–7]. Webb et al. have studied the yttrium–EDTMP (ethylenediamine tetra(methyl phosphonic) acid) complexes in solution and have reported their formation constants [7]. Yttrium is not a lanthanide element, but with its atomic and ionic radii and its inorganic and biological chemistry, it is similar to the lanthanides [8]. Webb has indicated that Y-EDTMP complexes, like those of Sm-EDTMP, can be used for tumour localisation.
Mention can also be made of the luminescent properties of lanthanide organophosphates and their uses in industrial chemistry [9]. With all these considerations, knowledge of the hydrolysis constants of the lanthanides appears to be primordial and the present work may also establish a link between the lanthanides and the actinides.
1.2 Thorium
Actinides have a greater facility for complexing than lanthanides. This is because of exclusively ionic bonds in the latter, whereas the former are known to form covalent bonds using their 5f electrons. Binuclear species with hydroxo bridges as well as polymeric ones have been characterised [10]. Usherenko and Skorik [11] have determined the hydrolysis constants of thorium by potentiometric methods. These authors have reported the precipitation of polynuclear species at higher concentration (CTh4+ = 5 × 10–3 mol dm–3). In a recent study on the aqueous solutions of Th(IV) using X-ray absorption fine structure spectroscopy techniques, Rothe et al. have confirmed the presence of polynuclear or colloidal species at high thorium concentrations [12].
Milic and Suranji [13] have varied the concentration of thorium and the supporting electrolyte and have showed that at defined pH the rate of hydrolysis of thorium increases with its concentration. A potentiometric study of the hydrolysis of thorium (IV) in 0.1 mol dm–3 potassium nitrate was carried out at 25 °C by Brown and Ellis [14]. Using the Miniquad software, the best model retained is that of [Th(OH)3+], [Th4(OH)124+], and [Th6(OH)159+], for which the formation constants are respectively: –2.98, –30.55 and –34.41.
Davydov and Toropov [15] have reported the predominance of the species Th(OH)3+ at pH < 4, and for thorium concentration between 2 × 10–5 and 2 × 10–4 mol dm–3. They have determined the first hydrolysis constant at ionic strength of 0.1 mol dm–3 and at 20 °C in nitrate or perchlorate solutions of thorium and have calculated β10–1 = (4.4 ± 1.2) × 10–5 or logβ10–1 = –4.36.
1.3 Hydration energy of thorium (IV)
The hydration energy of tetravalent thorium is much higher than those of lanthanides, which is due to its high charge density. Marcus [16] has calculated the value of ΔHhyd = –6000 kJ mol–1. Using thermodynamic data, he has evaluated for ri (Th4+) = 1 Å the thickness of the hydration layer at 2.36 Å and the number of fixed water molecules at 14. For the sake of comparison, he has given for lanthanum (ri = 1.05 Å), a thickness of hydration layer equal to 1.97 Å and 10 for the number of fixed water molecules.
1.4 Hydrolysis of lanthanides (III) and of thorium (IV)
A preliminary study of hydrolysis of lanthanides (III) and thorium (IV) was undertaken and this consisted of titration of their metal salts solutions by an aqueous solution of sodium hydroxide. This is necessary in order to determine the hydrolysis constants that are to be injected as fixed values for the calculation of the stability constants of the complexes of lanthanides (III) and of thorium (IV) cations with (phenolic) mono- and dimethylenephosphonic acids [17,18] synthesised by Böhmer et al. [19]. The treatment by Sirko-P [20] and Superquad [21] of the different titration curves corresponding to different lanthanides permitted the interpretation of this increase of H+ ions concentration by the following hydrolysis equilibriums:
The overall hydrolysis constants K*1,i are related to the overall stability constants, β1,0,i of the equilibrium:
2 Experimental section
2.1 Reagents
The lanthanide (III) chlorides were commercial products p.a. (Janssen for Ce, Pr and Eu; Aldrich for Nd; and Ventron for Sm). Solutions of 0.1 mol dm–3 NaClO4
2.2 Potentiometric measurements
The present investigation of metal-binding equilibriums was carried out as a series of potentiometric titrations at 25.0 ± 0.1 °C and constant ionic strength of 0.1 mol dm–3 (NaClO4), adding NaOH to lanthanum chlorate and thorium nitrate solutions containing initially a slight excess of HClO4. In each titration the total metal concentration M was kept constant in order to make the calculations simple. Since the proton and metal concentrations were kept low compared with the concentration of perchlorate ion, the activity factors are assumed constant, and hence, for calculations, concentrations could be used instead of the activities. The titrating agent NaOH (1 or 0.1 mol dm–3) used was stored in a polyethylene container equipped with a CO2 trap. All experiments were performed according to the method previously described [18].
Under the above experimental conditions, a hydrolysis study was undertaken for the lanthanide salts noted Ln3+ (Ce3+, Pr3+, Nd3+, Sm3+, Eu3+) and of thorium noted as Th4+. 10 ml of an aqueous solution of LnCl3 (CLn3+ = 10–3 mol dm–3) and of Th(NO3)4 (CTh4+ = 10–4 mol dm–3) were titrated against NaOH solutions of 0.1 mol dm–3 and 0.01 mol dm–3 respectively. This was carried out under an atmosphere of argon at 25 °C and at constant ionic strength, I = 0.1 mol dm–3, maintained by NaClO4.
2.3 Computations
The numerical treatment of the experimental data was carried out with the help of the softwares Sirko_P [20] and/or Superquad [21]. Several titrations, each including at least 150 pairs of data, were simultaneously processed with Sirko. The values reported in the tables are the mean values of the results of N independent runs, given with their 95% confidence interval ±2 σN–1,where σ is the standard deviation. R-factor (Rf) is the result of a statistical test related to the fit between the experimental and calculated curves.
3 Results
3.1 Lanthanides
During titrations up to five equivalents of base were added. These curves are presented in Fig. 1 and the results of calculations are summarised in Table 1.
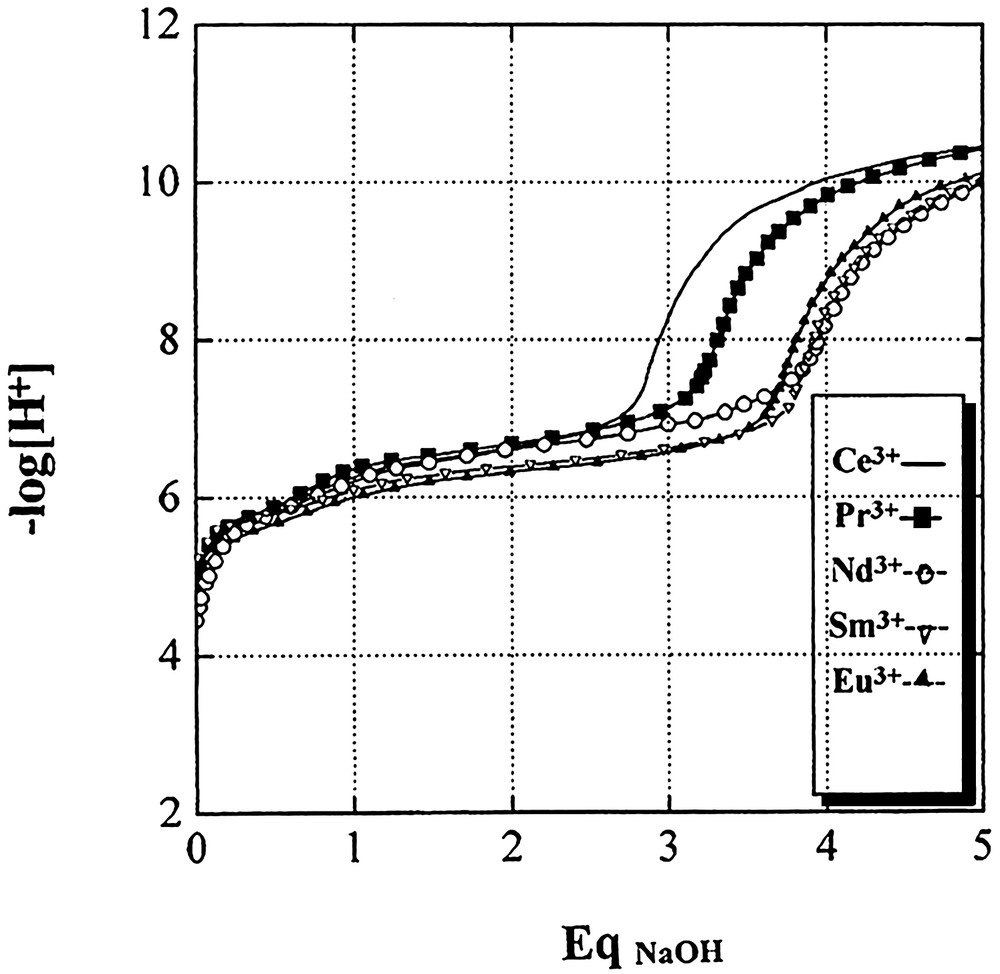
Titration curves of different lanthanide (III) cations against NaOH in aqueous solution at 25 °C (CLn3+ = 10–3 mol dm–3 and I = 0.1 mol dm–3 NaClO4).
Logarithms of stability constants of the hydroxo species of lanthanide (III) cations (25 °C, I = 0.1 mol dm–3 NaClO4 and CLn3+ = 10–3 mol dm–3)
Cation | xyz | species | log βxyz ± σn–1 | pH range | Rf (%) |
Ce3+ | 10–1 | Ce(OH)2+ | –6.87 ± 0.08 | 2.46–11.29 | 1.8 |
10–3 | Ce(OH)3 | –19.82 ± 0.05 | |||
10–4 | Ce(OH)4– | –28.93 ± 0.06 | |||
20–3 | Ce2(OH)33+ | –15.93 ± 0.09 | |||
20–7 | Ce2(OH)7– | –44.7 ± 0.1 | |||
Pr3+ | 10–1 | Pr(OH)2+ | –7.5 ± 0.2 | 2.43–11.27 | 1.7 |
10–3 | Pr(OH)3 | –19.78 ± 0.04 | |||
10–4 | Pr(OH)4– | –29.84 ± 0.05 | |||
20–3 | Pr2(OH)33+ | –15.60 ± 0.08 | |||
20–5 | Pr2(OH)5+ | –29.42 ± 0.09 | |||
Nd3+ | 10–1 | Nd(OH)2+ | –6.19 ± 0.06 | 2.45–11.21 | 2.6 |
10–2 | Nd(OH)2+ | –12.8 ± 0.1 | |||
10–3 | Nd(OH)3 | –20.1 ± 0.2 | |||
10–4 | Nd(OH)4– | –26.8 ± 0.1 | |||
Sm3+ | 10–1 | Sm(OH)2+ | –6.27 ± 0.06 | 2.40–11.22 | 2.3 |
10–3 | Sm(OH)3 | –18.77 ± 0.07 | |||
10–4 | Sm(OH)4– | –26.11 ± 0.07 | |||
20–3 | Sm2(OH)33+ | –15.3± 0.2 | |||
Eu3+ | 10–1 | Eu(OH)2+ | –7.4 ± 0.7 | 2.50–11.20 | 1.9 |
10–3 | Eu(OH)3 | –19.3 ± 0.1 | |||
10–4 | Eu(OH)4– | –26.2 ± 0.1 | |||
20–3 | Eu2(OH)33+ | –15.4 ± 0.2 |
The absence of formation of M(OH)2+ is to be noted for all lanthanide ions except for Nd3+. This means that this species probably exists in all cases but in negligible quantities, and consequently, it is rejected from the proposed model submitted for the calculations for refinement
The values of log β10–1 are between 6 and 7.5 log units and take into consideration the presence of hydroxo and binuclear species. The values reported in the literature are between 5.5 and 10, but they have been calculated under the assumption that only M(OH)2+ is present within the interpreted zone. The uncertainties given for logβ10–1 for our results and those of the literature are centred on the same mean values. Eventually, Klungness and Byrne have recently published their results on the hydrolysis behaviour of the rare earths at 0.7 molar and zero ionic strength and have shown the dependence of hydrolysis constants on temperature and ionic strength [23]. Their values differ from ours by about one log unit. The following discussion will be based on our calculated values.
3.2 Thorium
Six base equivalents are necessary for titration in this case. These titration curves are illustrated in Fig. 2 and the results are presented in Table 2.
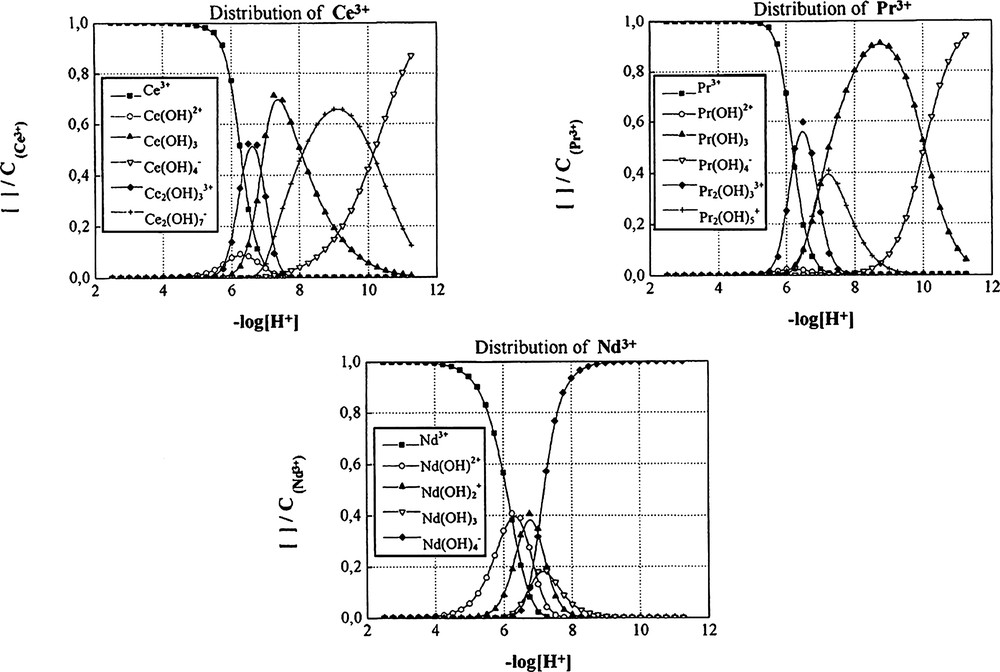
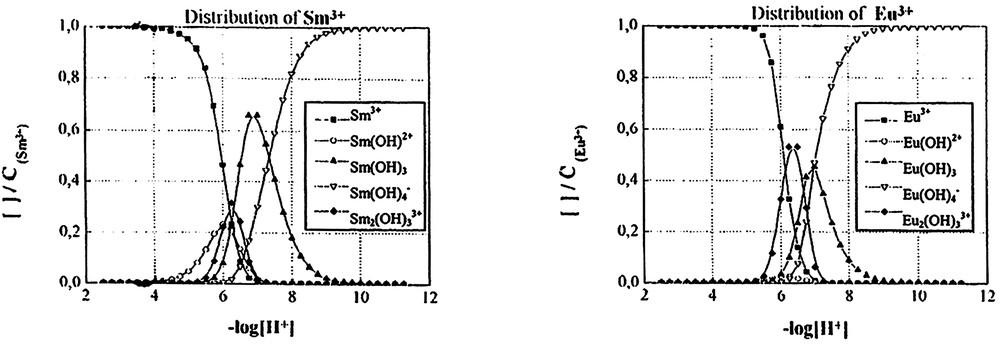
Distribution curves of the hydroxo species of different lanthanide (III) cations as a function of –log [H+] with CLn3+ = 10–3 mol dm–3.
Logarithms of stability constants of the hydroxo species of thorium (IV) cation (25 °C, I = 0.1 mol dm–3 NaClO4, and CTh4+ = 10–4 mol dm–3)
Cation | xyz | Species | log βxyz ± σn–1 | pH range | Rf (%) |
Th4+ | 10–2 | Th(OH)22+ | –8.36 ± 0.11 | 3.824–9.726 | 0.9 |
10–3 | Th(OH)3+ | –11.63 ± 0.09 | |||
10–4 | Th(OH)4 | –18.24 ± 0.13 | |||
20–7 | Th2(OH)7+ | –24.32 ± 0.08 |
The interpretation of the experimental data leads to a very good agreement for the model Th(OH)3+, Th(OH)4 and Th2(OH)7+. The Th(OH)3+ species was not retained for calculations because of its presence in extremely low quantities. This was previously reported by Moulin et al. [24], and all authors who have calculated the formation constant of this hydroxide, have reported very low values.
4 Discussion
4.1 Nature of the species formed
If one refers to Table 1, which presents all the results within pH range (2–11), several anionic mononuclear hydroxo complexes are observed for the lanthanides, namely M(OH)2+, M(OH)3 , M(OH)4–, M2(OH)33+ for Ce, Pr, Sm et Eu and two binuclear hydroxo complexes M2(OH)5+ for Pr and M2(OH)7– for Ce. In the case of thorium, the identified hydroxo complexes are Th(OH)3+, Th(OH)3+, Th(OH)4, and Th2(OH)7+.
The Th4+ cation undergoes hydrolysis to a greater extent than the cations of lanthanides as is evident from the high values of its calculated constants for the above species.
4.2 Distribution curves of the complexes
4.2.1 Lanthanides
The distribution curves for the hydroxo species for CM = 10–3 mol dm–3, drawn with the help of software Haltafall [25], are shown in Fig. 3. It is seen that at pH 6, the percentage of free cations is 75% for Ce3+, Pr3+, 60% for Nd3+, Eu3+, whereas for Sm3+ only 50% of free cations are present at this pH. Therefore, the Sm3+ cation is the one most easily hydrolysed. In any case, the other species coexist within the range of pH between 6 and 8 with a predominance for the neutral species M(OH)3.
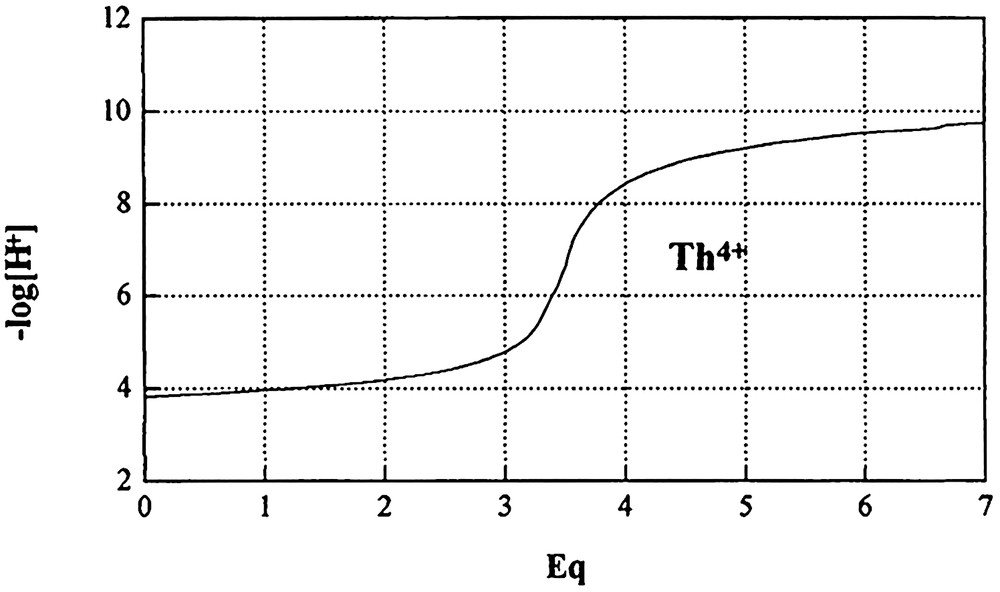
Titration curves of thorium (IV) cation against NaOH in aqueous solution at 25 °C (CTh4+ = 10–4 mol dm–3and I = 0.1 mol dm–3 NaClO4).
4.2.2 Thorium
Fig. 4 gives the distribution curves for the various hydrolysed species formed with thorium.

Distribution curves of the hydroxo species of thorium (IV) as a function of –log [H+] with CTh4+ = 10–4 mol dm–3.
At pH = 4, only 30% of Th4+ is in the free state, as most of it is transformed into Th(OH)3+ (60%) and Th(OH)22+ (10%). The predominant species are quite well defined within precise zones of pH. Thus, Th(OH)3+ attains its maximum of formation (70%) between pH 4 and 5, whereas at pH values between 8 and 9, the neutral species Th(OH)4 is formed at 70%. The binuclear species Th2(OH)7+ is formed predominantly, about 78%, within a broad interval of pH, between 5 and 8. It may be remarked here that in the acidic zone, pH = 4, only 10% of the dihydroxo species, Th(OH)22+ is formed and probably herein lies the explanation for the absence of the formation of the monohydroxo species Th(OH)3+.
4.3 Comparative study
4.3.1 Lanthanides
Table 3 collects the different species found by Schwinte [4] along with their methanolysis constants for Pr3+ and Eu3+. They are compared with the stoichiometry and the stability constants of the identified species with the same cations in this work.
Comparison of the solvolysis constants of lanthanide (III) in methanol and in aqueous solution
Cation | xyz | [Methanolysis ]a | [Hydrolysis ]b | ||
Species R = Me | log βxyz | Species | log βxyz | ||
Pr3+ | 10–1 | Ln(OR)2+ | –6.96 | Ln(OH)2+ | –7.49 |
10–2 | Ln(OR)2+ | –16.01 | — | — | |
10–3 | Ln(OR)3 | –26.53 | Ln(OH)3 | –19.78 | |
10–4 | Ln(OR)4– | –39.76 | Ln(OH)4– | –29.84 | |
20–3 | — | — | Ln2(OH)33+ | –15.60 | |
20–5 | Ln2(OR)5+ | –39.05 | Ln2(OH)5+ | –29.42 | |
Eu3+ | 10–1 | Ln(OR)2+ | –7.11 | Ln(OH)2+ | –7.39 |
10–2 | Ln(OR)2+ | –15.27 | — | — | |
10–3 | Ln(OR)3 | –27.23 | Ln(OH)3 | –19.27 | |
10–4 | — | — | Ln(OH)4– | –26.25 | |
20–3 | Ln2(OR)33+ | –18.51 | Ln2(OH)33+ | –15.36 | |
20–5 | Ln2(OR)5+ | –38.66 | — | — |
a Methanolysis: CLn3+ = 10–3 mol dm–3, 25 °C, and I = 0.01 mol dm–3 Et4NClO4.
b Hydrolysis: CLn3+ = 10–3 mol dm–3, 25 °C, and I = 0.1 mol dm–3 NaClO4.
A careful study of Table 3 highlights three main observations:
- • (i) the nature of the species in methanolic solutions is identical to the species identified in the aqueous medium;
- • (ii) the overall stability constant of the species Ln(OH)2+ is of the same magnitude whatever the solvent;
- • (iii) the solvolysis constants of the species present in alkaline medium are rather higher in the methanol medium.
4.3.2 Thorium
Different solvolysed species and their constants found by Schwinte [4] during her methanolysis study and those found in water in this hydrolysis study are collected in Table 4. The species Th(OH)3+ is not observed whereas Th(OR)3+ exists. It is concluded that thorium is more easily solvolysed in the aqueous medium than in methanol. This is supported by the fact that a second hydrolysed species Th(OH)22+ is observed, which is not the case in methanol.
Comparison between the solvolysis constants of thorium (IV) in methanol and in aqueous solution
xyz | Solventa | Species | log βxyz | Solventb | Species | log βxyz |
10–1 | CH3OH | Th(OR)3+ | –3.36 | H2O | — | — |
10–2 | — | Th(OH)22+ | –8.36 | |||
10–3 | Th(OR)33+ | –15.94 | Th(OH)3+ | –11.63 | ||
10–4 | Th2(OR)4 | –24.68 | Th(OH)4 | –18.24 | ||
20–5 | Th2(OR)53+ | –22.6 | — | — | ||
20–7 | Th2(OR)7+ | –36.6 | Th2(OH)7+ | –24.42 | ||
20–9 | Th2(OR)9– | –59.2 | — | — | ||
a Methanolysis: CLn3+ = 10–3 mol dm–3, 25 °C and I = 0,01 mol dm–3 Et4NClO4.
b Hydrolysis: CTh4+ = 10–4 mol dm–3, 25 °C and I = 0.1 mol dm–3 NaClO4.
Nevertheless, it is remarked that in the same pH range, globally, the nature of species in methanol conforms to those found in this study, i.e., in water. The only constant that is frequently cited in the literature in an hydrolysis study of thorium in aqueous medium is that of Th(OH)22+. It varies between –6.5 and –8.0, which is in agreement with the value found in this study, equal to –8.36.
5 Conclusion
The literature offers a number of values for the hydrolysis constants but they have to be compared with extreme caution, as they are determined under different experimental conditions. This is especially the case as the pH range studied is quite limited up to now.
The tendency for hydrolysis increases with the increase of atomic number and the contraction of the ionic radius. Normally, the actinides are more readily hydrolysed in aqueous medium than the lanthanides and this is confirmed in this study. Strictly, actinides (III) should be compared to lanthanides (III), however, the former are very similar to the latter and so in this work thorium (IV) has been compared to lanthanides (III) in order to observe the influence of charge on the complexing ability of the ion. The Th4+ ion is more resistant to hydrolysis than the rest of the actinides, but still it undergoes significant hydrolysis for pH values of 3 and onwards. Different species, more or less complex, are formed depending either on the conditions of pH or on the anions that are present and on their concentrations. Mainly polymeric hydroxo species are formed.