1 Introduction
Polyoxometalates obtained with d0 transition metal cations such as VV, MoVI and WVI can be considered as potential ‘electron reservoirs’ and studies on the obtention of their reduced derivatives have started a long time ago. The first application of electrochemical techniques to the study of the reduction of polyoxometalates was due to Souchay [1] who applied the polarography to the characterization and the study of the redox behavior or polyoxotungstates. Extension to the more oxidizing polyoxomolybdates began in the 1960s with Massart [2] who used platinum as the working electrode.
Two types of reduced polyoxometalates have to be considered, commonly named ‘heteropoly-blues’ and ‘heteropoly-browns’, by reference to their color. In heteropoly-blues, the electrons are trapped on single metal atoms at very low temperature but are delocalized at higher temperature (hopping). They are obtained from the oxidized species by successive reversible one or two electrons reduction steps, depending on the pH. For example, reduced species I–V (roman numerals denote the number of electrons added in the polyoxometalate) have been obtained between acid medium and 2 M NaOH as the reduction state increased for the Keggin anion α-[SiW12O40]4– [3]. On the contrary, heteropoly-browns are known only for tungsten compounds and with definite number of electrons, 6, 12, 18 or 24, in the Keggin structure. The six-electron reduced compound has been obtained with α-[XW12O40]n– anions (X = B, Si, H2) in acid solution. The six electrons are localized on three edge-shared tungsten atoms and each tungsten atom of this triad has a water ligand, α-[XW9O37{WIV(H2O)}3]4– [4,5]. It was denoted VI′ in order to differentiate with the heteropoly-blue VI.
The mechanism of formation of VI′ was first demonstrated for α-[SiW12O40]4– in agreement with polarographic behavior and controlled potential coulometry [6] and was later confirmed by cyclic voltammetry [7]. VI′α is formed in acid solution (pH < 1) from II by an ECE mechanism:
The first reversible two-electron step is followed by the irreversible disproportionation of IVα in acid solution and VI′α undergoes a reversible two-electron reduction at the same potential since the half-wave potentials of the redox couples IIα/IVα and VI′α/VIII′α are almost identical. Obtention of the brown species VI′α needs an electrolysis at a less negative potential in order to oxidize VIII′α. It is worthy of note that localized and delocalized electrons coexist in VIII′. The mechanism is slightly different for the boron anion α-[BW12O40]5– since the first reduction wave in acid solution corresponds to six electrons [8]:
An analogous mechanism has been later demonstrated for the metatungstate anion [H2W12O40]6– [9].
Two isomers β and γ of the Keggin anion α-[SiW12O40]4– are known. They derive formally of the α structure by rotation of π/6 of one and two trioxotungstic groups, respectively. Consequently, the symmetry of the anion decreases from Td to C3v and C2v and there are two types of trioxotungstic groups both in β and γ isomers, in the ratio 1/3 and 2/2, respectively. The influence of this non equivalence of the tungsten atoms has been studied for the blue 2e reduced γ isomer by 183W NMR [10]: the electrons are preferentially localized on four of the tungsten atoms, belonging to two equivalent trioxotungstic groups. We report in this paper the electrochemical synthesis of the brown reduced derivatives of the β and γ-[SiW12O40]4– anions with the initial aim to determine if there is a preferential localization of the three adjacent WIV centers on one of the two types of trioxotungstic groups.
2 Experimental section
The acid β-[H4SiW12O40] was prepared according to the previously reported procedure [11]. Since oxidized γ-[H4SiW12O40] is not stable, the reduced derivatives were obtained from K8γ-[SiW10O36] prepared as previously reported [12].
Polarograms were recorded in deaerated 1 M H2SO4 solution with a dropping mercury electrode and potentials were quoted against a saturated calomel electrode. For clarity, the oscillations in current arising from the growth and fall of the individual drops have been smooth down in the Figs. 1, 3 and 4. Controlled potential electrolyses were performed under nitrogen flow in 1 M H2SO4 with a mercury pool as the working electrode and a graphite counter electrode of large surface area.

Polarogram of the solution of the β-12-tungstosilicate during electrolysis in 1 M H2SO4; a : oxidized anion Oβ; b and c : after addition of n = 4 (IVβ) and n = 10 electrons (X′β), respectively; d and e: after reoxidation, with n = 8 (VIII′β) and n = 6 (VI′β), respectively.
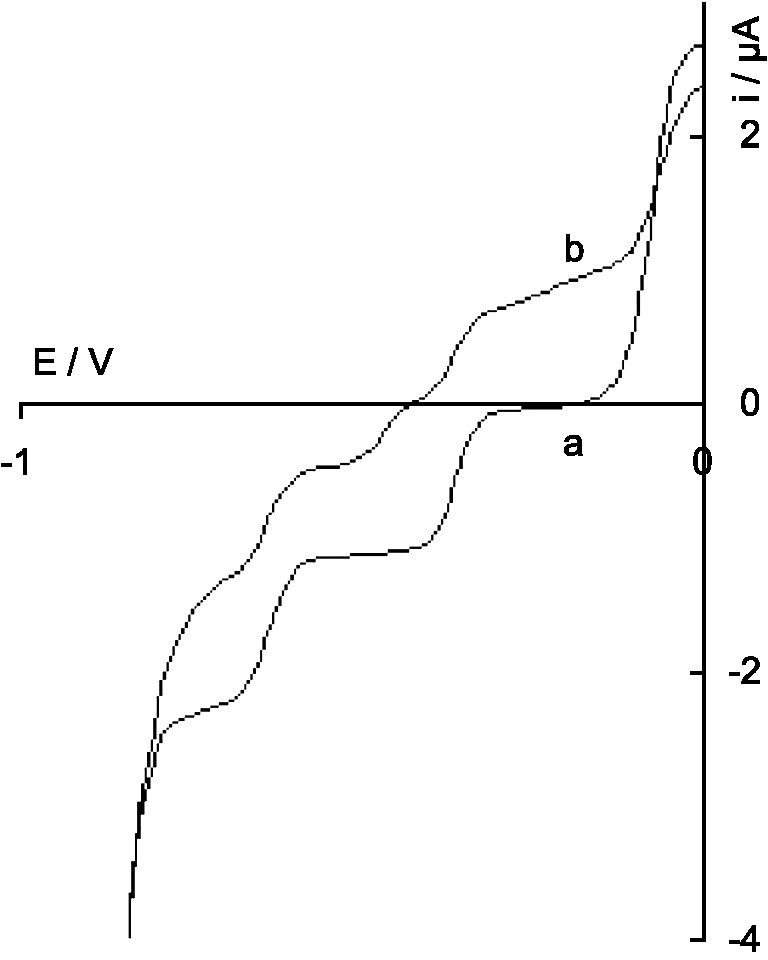
Variation with time of the polarogram of the brown anion VI′β; a: immediately after electrolysis; b: after 1 day.

Polarogram of the solution of the γ-12-tungstosilicate during electrolysis in 1 M H2SO4; a : two-electron reduced anion IIγ; b: after addition of n = 4 electrons in the anion; c: after addition of n = 8 electrons (VIII′γ); d: after reoxidation, with n = 6.
3 Results
3.1 Electrochemical reduction of β-[SiW12O40]4–
The polarogram of β-[SiW12O40]4– (noted 0β) shows three waves of 1, 1 and 2 electrons, respectively (Fig. 1, curve a). The first two waves are not well separated in 1 M H2SO4 since the reduced species are protonated (see for example [3] for the influence of the pH on the redox behavior of polyoxotungstates). Subsequent multielectronic waves are more or less mixed up with the reduction of the protons in this medium.
Reduced compounds Iβ, IIβ and IVβ were successively obtained by controlled potential electrolysis at –0.55 V/SCE as shown by the variation of the equilibrium potential of the solution in function of n, the number of added electrons (Fig. 2). The curve b of the Fig. 1, recorded with the solution of IVβ, shows three anodic waves with the same half-wave potentials that the cathodic waves characteristic of 0β, underlying the reversible behavior of these systems.

Variation of the equilibrium potential of the solution of the β-12-tungstosilicate in function of the number of electrons added in the polyanion.
More reduced derivatives were obtained when electrolysis was carried on at a potential of –0.75 V/SCE. For n > 4, the potential of the solution was not very stable suggesting that a chemical reaction occurred with the electrochemical process. It did not much change up to n = 10 (Fig. 2). The polarogram of this solution shows three anodic waves at –0.64, –0.37 and –0.07 V/SCE (Fig. 1, curve c) but the reversible waves characterizing IVβ, especially the well defined IVβ/IIβ wave at –0.47 V, are not observed. The first two new anodic waves have the same intensity that the initial IIβ/IVβ one, so they correspond to two-electron electrochemical processes. The relative height of the third wave depends on the concentration of the β-12-tungstosilicate and increases as the concentration decreases and tends towards 6e for a concentration as low as 10–5 M. Such a dependence has been already observed with the α isomer for the 6e anodic wave characterizing the oxidation of VI′α to 0α in acid solution [6,7].
The blue solution corresponding to n = 10 was oxidized at a potential –0.25 V/CSE in order to remove electrons from the anion. Two species were successively obtained, corresponding to the two equivalent points on the curve showing the variation of the equilibrium potential of the solution (Fig. 2). For n = 8, the solution was always blue and the first anodic wave became cathodic (Fig. 1, curve d). For n = 6, the second wave became cathodic in its turn and the solution was then brown (Fig. 1, curve e). The cathodic and anodic half-wave potentials have the same value, showing the reversible behavior of these systems. Lastly, oxidation at 0 V led to the progressive and irreversible restoration of the polarogram of 0β with, simultaneously, the disappearance of the three waves observed for n = 6. All these observations, and analogy with the known behavior of the α isomer, show that the species obtained for n = 6 corresponds to the brown anion VI′β and those obtained for n = 8 and 10 correspond to the more reduced blue derivatives of VI′β, abbreviated VIII′β and X′β, respectively. The electrochemical characteristics of the redox systems of β-[SiW12O40]4– are indicated in Table 1.
Half-wave potential values (± 0.1 V) of the α, β and γ-[SiW12O40]4– isomers in 1 M H2SO4. All the waves are reversible, excepted VI′/0, which is irreversible
Isomère | 0/I | I/II | II/IV | IV/VI | VI′/0 | VI′/VIII′ | VIII′/X′ |
α | –0.24 | –0.48 | –0.60 | – | –0.08 | –0.58 | – |
β | –0.12 | –0.24 | –0.46 | – | –0.07 | –0.36 | –0.63 |
γ | –0.12a | –0.22a | –0.46 | –0.58 | –0.05 | –0.32 | – |
a Ill-defined anodic waves.
Actually, VI′β is only metastable in acid solution since its solution, initially brown, became blue. The polarogram of the solution kept in 1 M H2SO4 under argon (Fig. 3, curve a) slowly changed with time, with a decrease of the intensity of all the waves characterizing VI′β, the translation of the VI′β/VIII′β wave from the cathodic to the anodic area and the appearance of the cathodic wave IIβ/IVβ (Fig. 3, curve b). After 1 day, the ratio of the waves VIII′β/VI′β and IIβ/IVβ was about 2/1. This corresponds to the slow disproportionation reaction in 1 M H2SO4:
Attempts to stabilize VI′β in aqueous solution failed. For example, when VIII′β was oxidized in acetic buffer (pH 4.3) at a potential of –0.4 V in order to obtain VI′β, the rate of the above reaction is so much high that IIβ was obtained as electrolysis progressed.
3.2 Electrochemical reduction of γ-[SiW12O40]4–
If the oxidized species γ-[SiW12O40]4– is not stable in aqueous solution, the two-electron reduced species IIγ can be obtained by electrolytic reduction of γ-[SiW10O36]8– in 1 M H2SO4 after addition of 2 Na2WO4 per mol, at a potential equal to –0.55 V/CSE, according to the reaction:
The polarogram of IIγ in 1 M H2SO4 shows four waves: two anodic one-electron waves, not well separated (these waves are well separated at higher pH) and two cathodic two-electron waves corresponding to the reduction steps IIγ/IVγ and IVγ/VIγ, with half-wave potentials –0.47 and –0.58 V, respectively (Fig. 4, curve a).
More reduced derivatives were obtained when electrolysis was carried on at a potential of –0.65 V. For n > 2, if the wave IIγ/IVγ shifted progressively to the anodic area, the intensity of the waves IIγ/IVγ and IVγ/VIγ decreased and new anodic waves at –0.33 and –0.05 V increased (Fig. 4, curve b for n = 4). For n = 8, only these anodic waves could be seen (Fig. 4, curve c) and have been attributed to VIII′γ/VI′γ and VI′γ/0γ electrochemical systems, respectively, from their intensity and by analogy with the behavior of α and β isomers.
Oxidation of this solution at a potential of –0.2 V does not lead to pure VI′γ species since, if the VIII′γ/VI′γ wave shifted partially to the cathodic area as electrolysis progressed, its intensity decreased and the characteristic waves of IIγ (cathodic IIγ/IVγ and IVγ/VIγ waves at –0.47 and –0.58 V, respectively) increased (Fig. 4, curve d). This means that, as for the β isomer, but at a higher rate in 1 M H2SO4, a disproportionation reaction of VI′γ occurred and IIγ was formed by an EC mechanism:
4 Discussion
The electrochemical syntheses of the brown 6e reduced β and γ isomers of [SiW12O40]4– in acid solution are summarized in Fig. 5 and half-wave potentials are indicated in Table 1. As for the α isomer, they are obtained by successive reversible electrochemical steps and disproportionation reactions and apparent differences between isomers result from differences of the kinetic stability of the reduced species and values of the half-wave potentials.

Schemes indicating the reduced species obtained during controlled potential electrolysis of the β and γ 12-tungstosilicates in 1 M H2SO4.
First, the 4e blue reduced derivative is stable in acid solution for the β isomer (or, at least, has a great kinetic stability), but is not stable for the α and the γ isomers which undergo a disproportionation reaction leading to the brown species:
This reaction had been directly demonstrated for the α isomer [3] and is obvious for the γ one if we consider the curve b of the Fig. 4: the wave IVγ/IIγ is effectively anodic after addition of 4e in the γ-anion, but its intensity is smaller than for n = 2 and the waves characteristic of the brown species are present in the anodic area. This means that the disproportionation reaction of IVγ is not very rapid in 1 M H2SO4 aqueous solution, on the contrary of the α isomer for which the IVα/IIα anodic wave has never been observed during electrolysis in acid solution [7].
For the three isomers, the brown 6e reduced anions can be reduced by 2e reversible electrochemical steps which have half-wave potentials less negative than the system II/IV (Table 1). Consequently, when VI′ is formed by the disproportionation reaction, it is further reduced at the electrode and a more reduced species is obtained. The final product depends on the relative values of the applied potential and of the half-wave potentials of the systems VI′/VIII′ and VIII′/X′. For the γ isomer, The IIγ/IVγ and IVγ/VIγ waves have E1/2 between VI′γ/VIII′γ and VIII′γ/X′γ and VIII′γ is the final product of the electrolysis, as it had been observed for the α isomer. For the β isomer, IVβ is stable and we have to apply a more negative potential to carry on with the electrolysis. The applied potential corresponds to a multielectronic wave just before the reduction of protons and is more negative that the E1/2 value of the VIII′β/X′β wave. So, X′β is the final product of the electrolysis. It may be presumed that VIβ is formed at the electrode when the applied potential is –0.75 V and that, like the boron analog, it is rapidly converted to the VI′β brown species with the same overall reduction state. Then VI′β undergoes two reversible two-electron reductions to VIII′β and X′β at the applied potential:
Reoxydation steps at controlled potential are thus necessary to obtain the brown species, VI′β or VI′γ. For the β isomer, the brown VI′β species has a high kinetic stability in acid solution and is obtained when the applied potential is –0.30 V. It can be isolated as a cesium salt for example, by addition of cesium chloride to the solution. On the contrary, IIγ has appeared as electrolysis progressed when VIII′γ was oxidized at –0.2 V, showing that VI′γ is very unstable in 1 M H2SO4.
Decrease of the stability of VI′ in the order α > β > γ can be discussed considering the distortions induced by isomerization and reduction of one trioxo-tungstic group [{WVI=O}3O10] to [{WIV(H2O)}3O10] by addition of six electrons and six protons. It has been shown [13] that rotation of trioxo-tungstic groups does not induce a change in their geometry nor modification of bond lengths, but only modifications of bond angles of the junctions between these groups. Especially, the W–O–W angle of the μ-oxo junction between a rotated group and the non-rotated adjacent groups decreases (153–145°), both for β and γ isomers. On the contrary, reduction and protonation of a triad leads to strong structural modifications: the displacement of the W atoms towards the center of the structure leads to a decrease of the W–W distance. Intuitively, these modifications would induce an increase of the W–O–W bond lengths and angle of the junction. So rotation and reduction of a trioxo-tungstic group would have opposite consequences and it is not surprising that the stability of the brown VI′ anion decreases in the sequence α > β > γ as the number of rotated groups increases. It is remarkable that addition of two electrons in these anions leads to stable (but very air sensitive) VIII′ species, at least in a kinetic sense. Stabilization of VI′β and VIγ would probably need utilization of a non aqueous solvent.