1 Introduction
There is now an increased effort to focus on the design and assembly of molecular devices that contain nano-clusters [1] given their potential application in catalysis [2], magnetic materials [3] electronics [2] and optics [4,5]. In this context, the bridge between solid-state and molecular materials is an appealing entry into these areas of nanosciences and a starting point towards a broad range of chemistry [6]. Branched molecular architectures [7] also offer another facet to these concepts and allow designing materials with cluster moieties at the core [8] and extremities [9] of dendritic nanostructures [10]. The inorganic clusters [Mo6X14]2- have been known for 60 years [11,12] and the related superconducting octahedral Mo6 clusters PbMo6S8, known as Chevrel phases [13], as well as their redox and photo-physical properties [14] added further interest. The Mo(II) halide clusters consist of a substitution-resistant core [Mo6X8]4+ with eight face-bridging inner ligands and semi-labile outer (non-bridging, i.e. terminal) ligands. The outer ligand substitution chemistry has been extensively investigated for almost half a century [15], the substitution of the two apical ligands being easier than that of the four equatorial ones [16]. The hexa-methoxy cluster prepared by Nannelli and Block [17] and the hexa-triflate reported by Shriver's group [18] are the most useful starting materials for further substitution [8b].
Our strategy has consisted in investigating the substitution, via the intermediacy of the hexa-triflate (Equation 1), of all six terminal bromide ligands in [n-Bu4N]2 [Mo6Bri8Bro6] by phenolate and bipyridine ligands connected to redox-active rigid or dendronic organometallic groups. Iron- and ruthenium-centered sandwich and piano-stool structures have been chosen as organometallic connectors, since they have well-known redox properties that can be useful for redox recognition and molecular electronics [19], especially in the dendritic context [20].
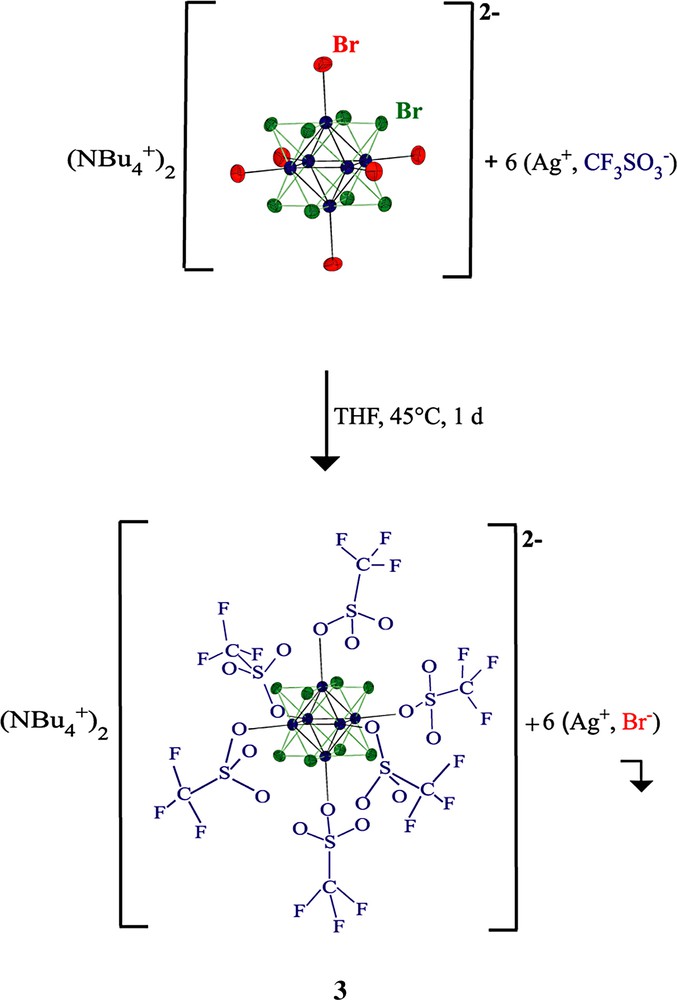
2 Results
2.1 Coordination of a pyridinyl-alcynyl-organo-ruthenium rod
The rigid organo-ruthenium pyridine ligand 1 was known [21]. We best synthesized it by a different route, however, involving the reaction of 4-ethynylpyridine with [RuCp(PPh3)2Cl] in the presence of triethylamine and [NH4][PF6] in methanol (Scheme 1). This general procedure was reported [22] for the synthesis of the complexes [RuCp(PPh3)2(η1-alcynyl)] with (Cp = η5-C5H5). The yellow complex 1 was characterized by standard spectroscopic techniques including 31P NMR, infrared spectroscopy (νCC = 2070 cm–1) and the MALDI TOF mass spectrum with the dominant molecular peak at 794 Dalton.

In order to compare the coordination of 1 to a single metal center with that in the Mo6 cluster, the orange silver complex 2 was synthesized from the ligand 1 and AgBF4 in methanol. In the infrared spectrum of the trimetallic complex 2, the alkynyl CC band was found at 2016 cm–1. Then, the reaction of 1 with the cluster [Mo6Br8(CF3SO3)6][n-Bu4N]2, 3, was carried out in refluxing THF for a week.
The new hexa-ruthenium hexa-molybdenum compound 4 was recovered by slow precipitation using pentane. A single peak was found in the 31P NMR spectrum, a single Cp peak was observed in the 1H NMR spectrum at δ = 4.42 ppm, and a single infrared absorption was obtained at νCC = 2018 cm–1. This behavior is in accord with the coordination of the cluster by the six organo-ruthenium pyridine ligands and the absence of free pyridine ligand in the sample (Equation 2).
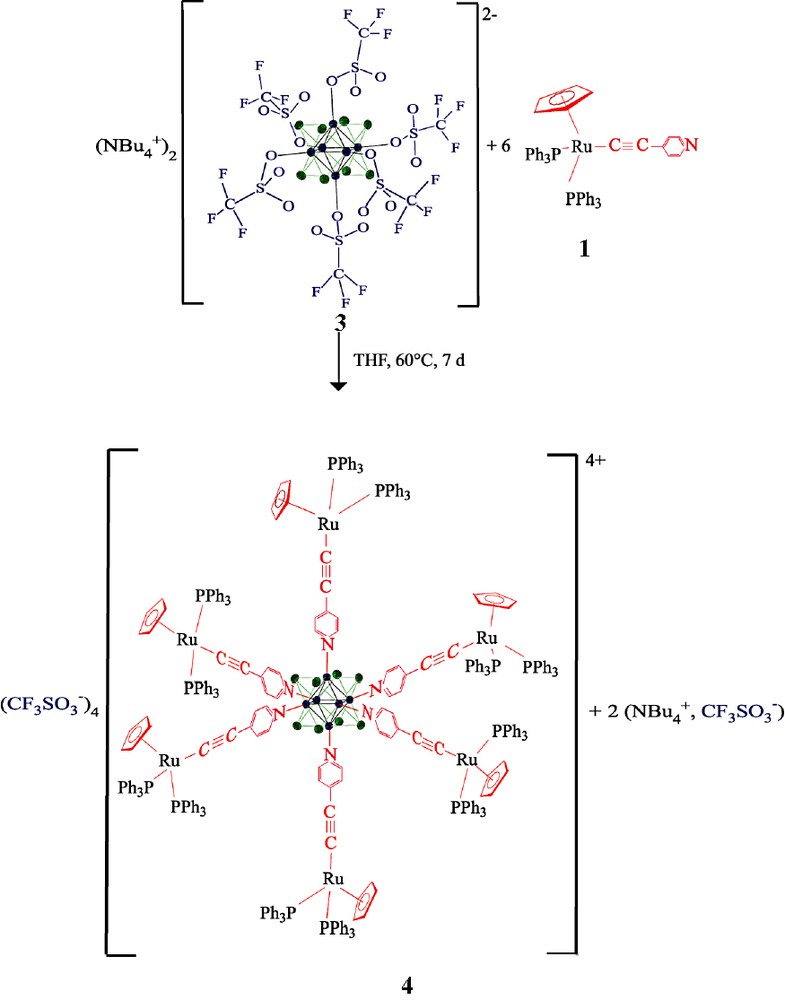
2.2 Coordination of a ferrocenyl-alcynyl-pyridine rod
In a similar approach, the red complex 1-ferrocenyl-2-(4-pyridinyl)acetylene, 5, was synthesized using the reaction of the Vargas'group (Scheme 2) [23]. The reaction of 5 with AgSO3CF3 or AgBF4 at room temperature in CDCl3 gives a red trimetallic complex 6 (shown with the triflate anion) resulting from the coordination of two equivalents of the pyridine nitrogen atom on the silver cation (Scheme 2). The compound 6 is light and air sensitive.

The reaction of the ferrocenyl-containing pyridinyl ligand 5 with the cluster 3 was carried out in refluxing THF for a week to give the new hexasubstituted hexaferrocenyl Mo6 cluster 7. The red compound 7 was isolated by precipitation from a THF solution by slow addition of pentane and characterized inter alia by an infrared alcynyl absorption at 2181 cm–1, ferrocene signals including a single Cp signal for the free Cp rings in the 1H and 13C NMR spectra (Equation 3). This complex 7 is very light and air sensitive. Its CV in CH2Cl2 shows two close reversible ferrocenyl waves at E1/2 = 0.800 V vs. [FeCp*2] with a less intense shoulder at E1/2 = 0.710 V vs. [FeCp*2]; these values compare with the value obtained for the starting monomeric complex 5, E1/2 = 0.720 V vs. [FeCp*2].

2.3 Coordination of a tri-silylferrocenyl-phenolate dendron
The known tri-silylferrocenyl phenolate dendron p-HO-C6H4C{CH2CH2CH2Si(Me)2Fc}3, 8, has already been used as part of a dendritic gold-nanoparticle-cored device for the recognition of inorganic oxo-anions [24]. Its reaction with the Mo6 cluster 3 was inspired by previous studies of substitution of the six outer (terminal) halide ligands including Gorman's work that involved dendronic phenolates [8b]. Thus, reaction of 8 with 3 was carried out overnight in THF at 60 °C, then in toluene at 80 °C for two days which eventually led to completion of the substitution reaction, giving the air-sensitive cluster-cored octadecyl-silylferrocenyl dendrimer 9. The end of the reaction was indicated by the correct ratio of the n-Bu4N+ cation/dendron signals in 1H NMR of 9 that was obtained only then (Equation 4).
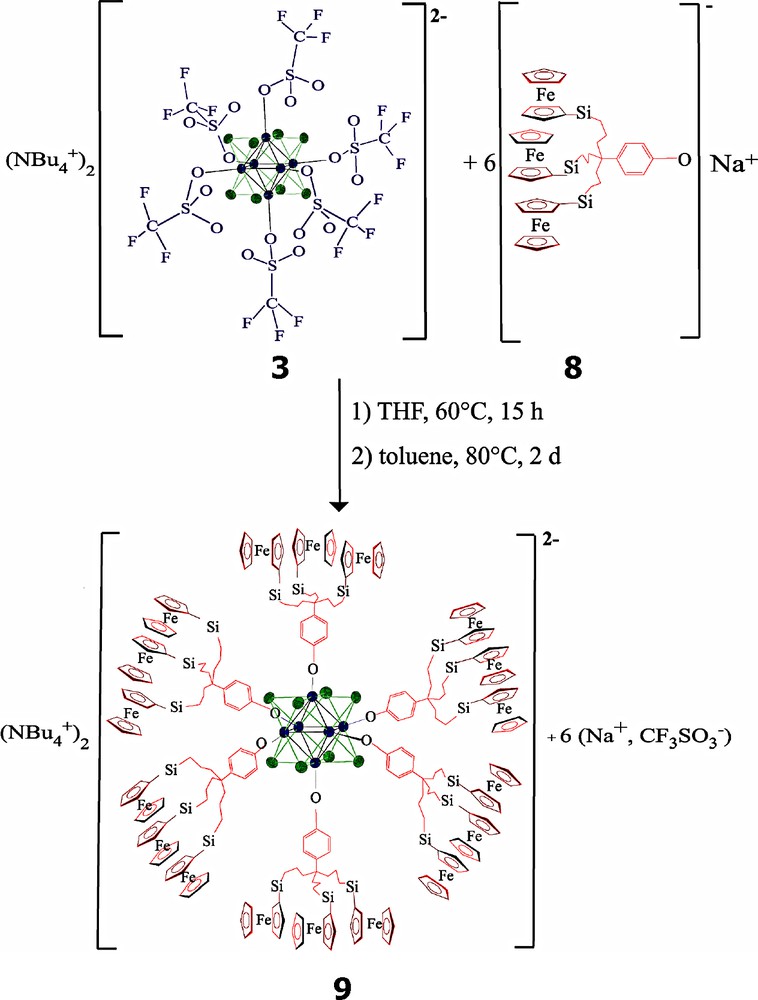
The CV of 9 showed a single oxidation wave for the 18 ferrocenyl redox centers indicating that these 18 ferrocenyl centers are seemingly equivalent as in other polyferrocenyl dendrimers [25]. Addition of [n-Bu4N]2[ATP] to the electrochemical cell leads to the appearance of a new ferrocenyl wave at less positive potential (ΔE = 90 mV) whose intensity is proportional to the proportion of [n-Bu4N]2[ATP] salt added. This is the indication of an interaction of strong type according to the Kaifer–Echegoyen model [26] as already observed previously with other polyamidoferrocenyl and polysilylferrocenyl dendrimers (Fig. 1). It is noteworthy that the shift of potential (Fig. 1) is much smaller than in gold-nanocluster-cored dendrimers.
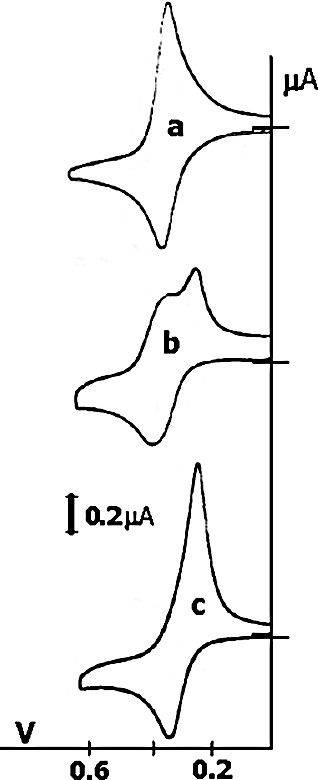
Titration of (Bu4N)2[MoBr8(OC6H4C(C3H6SiC2H6Fc)3)6] in CH2Cl2 (Pt, [Bu4N][PF6] 0.1 M, 20 °C, reference: [FeCp2*], not shown) with [Bu4N]2[ATP]; a) Before the addition, E1/2 = 0.40 V; b) with 0.30 equiv. of anion; c) with an excess of anions, E1/2 = 0.31 V. The two methyl substituents on all Si atoms are ommited for clarity.
3 Discussion
Precedents showing hexa-substitution of the outer (terminal) halides in the octahedral inorganic clusters such as 3 [8b,18] provided the basis for the new hexa-substitution reactions described in this work. The rigidity and electronic delocalization in the hexa-pyridine-substituted clusters on one hand, and the bulk of the flexible phenolate dendron on the other hand, however, were factors rendering this chemistry challenging. Finally, the two new hexa-substituted pyridine clusters are light- and air-sensitive, and the cluster-cored 18-ferrocenyl dendrimer is air sensitive which made their work-up and use tedious. Thus, parallel reactions with the mononuclear silver salts, although also very light sensitive, were welcome to facilitate assignments and help understand the spectroscopic intricacies. The substitution reactions could indeed be followed by 1H NMR using the relative intensity ratio of appropriate signals, and this technique allowed us to confirm the difficulty of hexa-substitution and the long heating time therefore required. We made the choice to follow Shriver's and Gorman's indications involving the hexa-triflates as intermediates for the substitution reactions.
It turned out that the hexa-substituted clusters (in particular the dendritic one) were very difficult to purify in order to obtain correct elemental analyses. These analytical data had to be submitted several times for each product to yield mediocre results, although the 1H NMR monitoring clearly showed the end of the substitution reactions. Inorganic impurities were obviously difficult to separate given the low carbon contents systematically obtained. Although this may be partly due to the air and light sensitivity of the compounds, it appears that triflate salts formed in the reactions may be systematic impurities due to the difficulty of their complete separation and detection. The light and air sensitivity of the hexa-alkynyl-pyridine clusters was probably also responsible for our lack of success in obtaining suitable crystals for X-ray crystal structure determinations. Yet, Schriver's group reported the crystal structure of a hexa-ferrocenyl cluster, but with carboxylate ligands [27].
The location of the alkyne C–C band in the infrared spectrum is very sensitive to the coordination and nature of alkyne substituent. For instance, this band is shifted from 2070 cm–1 in the free organoruthenium-pyridine 1 to 2016 and 2018 cm–1 in the silver- and cluster complexes 2 and 4 respectively. Likewise, in the ferrocenyl-alkynyl pyridine, the band is shifted from 2210 cm–1 in the free ligand to 2176 and 2181 cm–1 in the silver and cluster complexes respectively. These shifts show the decrease of electronic density on the triple bond due to charge transfer from the pyridine ligands to the metals. Likewise, the Cp peak observed in 1H NMR, whose singularity is an evidence of complete hexa-substitution, is shifted downfield upon coordination of both organometallic pyridines to the metals. On the other hand, it is remarkable that the change of location of signals of the pyridinyl protons α to the nitrogen atom is very minute, whereas the shift of that of the meta protons is more important, as in Lindner's pyridine-silver complexes [28]. The substituted Cp ring protons are also slightly more deshielded in the β positions than in the α one.
The CV of the three cluster-cored poly-organometallic compounds could be recorded in the ferrocenyl and ruthenium regions, but rather little information could be obtained else than a single partially reversible wave for the hexa-ruthenium system as for the monomer. The hexa-ferrocenyl compound 7 shows a major reversible wave and a weak shoulder. This behavior could tentatively be attributable either to small amounts of decoordinated monomeric ferrocene derivative 5 whose potential is the same as that of the shoulder or to the distinction between the two sets of positions (apical and equatorial). Finally, the cluster-cored 18-silylferrocenyl dendrimer appears to share most of the properties of previously reported covalent [25], hydrogen-bonded [29] and gold-nanoparticle cored [24] polyferrocenyl dendrimers except that it is air sensitive due to the nature of the core. As in other polyferrocenyl dendrimers, the apparent equivalence of all the ferrocenyl groups in term of the seemingly unique redox potential allows to use this nanocluster as exo-receptor for the recognition and titration of oxo-anions whose prototype is ATP2–. The sensing property by the 18-silylferrocenyl dendrimer is about the same as with the amidoferrocenyl dendrimers [25], but it is of easier access and presents the advantage that the oxidized ferrocenium form is fully stable, contrary to that of amidoferrocenyl moieties [24,25]. The CVs show a rather nice recognition of ATP2– in spite of the relatively modest wave shift of 90 mV. The advantages of the golden-nanoparticle-cored polyferrocenyl dendrimers, however, are the air stability and their larger size (up to about 360 ferrocenyl groups) allowing a very easy electrode derivatization [24] that does not work at this time precisely because of the reduced size of this cluster-cored dendritic assembly.
4 Concluding Remarks
Three new hexasubstituted octahedral Mo6 clusters with organometallic groups have been synthesized from the precursor [n-Bu4N]2[Mo6Br8(CF3SO3)6] upon prolonged heating. The substitution reactions of the triflate by the functional pyridine- or phenolate ligands were monitored by 1H NMR that showed clean hexasubstitution. The two cluster-cored hexa-pyridine organometallics obtained are light and air sensitive and the 18-Fc dendrimer is air sensitive, which makes their purification, isolation and analysis tedious. Nevertheless, 1H NMR is a convenient method to investigate both their synthesis and the electronic changes occurring upon coordination along the organometallic pyridine rods. The Mo6-cluster-cored 18-silylferrocenyl dendrimers resembles, through its periphery, other polyferrocenyl dendrimers assembled by covalent bonds, coordination to gold nanoparticles and hydrogen bonds and, in particular, it behaves as an exo-receptor allowing to recognize and titrate the biologically important anion ATP2–.
5 Experimental Section
5.1 General data
Reagent-grade tetrahydrofuran (THF) and pentane were predried over Na foil and distilled from sodium-benzophenone anion under argon immediately prior to use. MeOH was distilled from magnesium just before use. All other chemicals were used as received. All manipulations were carried out using Schlenk techniques or in a nitrogen-filled Vacuum Atmosphere dry lab. 1H NMR spectra were recorded at 25 °C with a Bruker AC 300 (300 MHz) spectrometer. 13C NMR spectra were obtained in the pulsed FT mode at 75 MHz with a Brucker AC 300 spectrometer. All chemical shifts are reported in parts per million (δ, ppm) with reference to Me4Si (TMS). The product 1 [21] was synthesized from commercial 4-ethynylpyridine and [RuCp(PPh3)2Cl] according to Bruce's general method of synthesis of the complexes [RuCp(PPh3)2(η1-alcynyl)] [22]. The precursor cluster 3 was synthesized according to reference [12d]. The reaction conditions used for the syntheses of substituted hexa-pyridine complexes were analogous to those reported in Fréchet's article [1].
Cyclic-voltammetric data were recorded with a PAR 273 potentiostat galvanostat. The reference electrode was an Ag quasi-reference electrode (QRE). The QRE potential was calibrated by adding the reference couple [FeCp2*]/[FeCp2*]+ (Cp* = pentamethylcyclopentadienyl). The working electrode (platinum) was treated by immersion in 0.1 M HNO3, and then polished before use and between each recording when necessary.
5.2 Synthesis of [RuCp(PPh3)2(CC-C5H4N)], 1
A methanol solution of 4-ethynylpyridine (0.296 g, 2.07 mmol) and 5 ml of triethylamine was added to a suspension of [RuCp(PPh3)2Cl] (1.00 g, 1.38 mmol) and NH4PF6 (0.449 g, 2.76 mmol) in methanol. The solution was stirred at room temperature for 3 days. The solvent was removed under vacuum and the product was extracted with toluene. The product was precipitated with toluene/pentane. The yellow powder was dried under vacuum. Yield 0.350 g (0.440 mmol) of yellow powder of 1 (32%).
1H NMR (MeOD, 300 MHz), δppm: 8.06 (d, NCH), 7.30 – 7.04 (m, PPh3), 6.80 (d, NCCH), 4.29 (s, Cp). 31P NMR (MeOD, 100 MHz), δppm: 49.60 (PPh3). Infrared: νCC= 2 070 cm–1. MS (MALDI-TOF; m/z), calcd. for C48H39NP2Ru: 792.85, found: 794.24. CV in CH2Cl2: E1/2 = 0.700 V vs. Fc* at 20 °C (partially chemically reversible: ic/ia = 0.5).
5.3 Synthesis of the trinuclear AgRu2 complex, 2
A methanol solution of 1 (0.0530 g, 0.0667 mmol) and AgBF4 (0.007 g, 0.0333 mmol) was stirred at room temperature for 12 h. The solvent was removed under vacuum and the product was precipitated with MeOH/pentane. The orange powder was dried under vacuum. Yield: 0.040 g of 2 as an orange powder (67%). Analysis calcd. for C96H78AgBF4N2P4Ru2: C: 64.76%, H: 4.42%, found: 64.45%, H: 4.13.
1H NMR (MeOD, 300 MHz), δppm: 8.08 (d, NCH), 7.28 – 7.05 (m, PPh3), 6.87 (d, NCCH), 4.33 (s, Cp). Infrared: νCC = 2 016 cm–1. CV in CH2Cl2: E1/2 = 0.940 V vs. Fc* at 20 °C (partially chemically reversible ic/ia = 0.5).
5.4 Synthesis of the hexa-substituted cluster Mo6-Ru6, 4
A THF solution of 1 (0.131 g, 0.165 mmol) and 3 (0.069 g, 0.0265 mmol) was stirred at 60 °C for 7 days. The solvent was removed under vacuum and the product was precipitated with THF/pentane. The red powder was dried under vacuum. Yield 0.115 g of red powder of 4 (65%). Analysis calcd. for Mo6Br8C292H234F12S4O12N6P12Ru6 (%): C 53.39, H 3.39, found: C 52.59, H: 2.97.
1H NMR (MeOD, 300 MHz), δppm: 8.12 (d, NCH), 7.23 – 7.08 (m, PPh3), 7.04 (d, NCCH), 4.42 (s, Cp). 31P NMR (MeOD, 100 MHz), δppm: 49.51(PPh3). Infrared: νCC = 2 018 cm–1. CV in CH2Cl2: E1/2 = 0.650 V vs. Fc* at 20 °C in CH2Cl2 (partially chemically reversible ic/ia = 0.1).
5.5 Synthesis of the hexa-ferrocenyl Mo6Fc6 cluster, 7
A THF solution of 5 (0.054 g, 0.187 mmol) and 3 (0.063 g, 0.029 mmol) was stirred at 60 °C for 7 days. The solvent was removed in vacuum and the product was precipitated with THF/pentane. The red powder was dried under vacuum. Yield 0.073 g of red powder of 7 (63%). 1H NMR (CDCl3, 300 MHz), δppm: 8.64 (d, NCH), 7.51 (d, NCCH), 4.62 and 4.41 (t, FeCH), 4.32 (s, Cp). 13C NMR (CDCl3, 63 MHz), δppm: 69.9 (FeC), 70.2 (Cp), 72.0 (FeC), 76.95 (CC), 125.9 (NCC), 147.5 (NC). Infrared: νCC = 2 181 cm–1. Elemental analysis calcd (%): C 36.03, H 2.22; found (%): C 35.29, H 1.59. CV in CH2Cl2 at 20 °C: E1/2 = 0.800 V vs. [FeCp*2] in CH2Cl2.
5.6 Synthesis of the trinuclear AgFc2 complex, 6
A chloroform-d solution of 5 (0.044 g, 0.0156 mmol) and AgSO3CF3 (0.020 g, 0.0078 mmol) was stirred at room temperature for 24 h. The solvent was removed under vacuum, and the product was washed with ether and dried under vacuum. Yield: 0.061 g of red powder of 6 (95%).
1H NMR (CDCl3, 300 MHz), δppm: 8.54 (d, NCH), 7.41 (d, NCCH), 4.61 and 4.40 (t, FeCH), 4.30 (s, Cp). 13C NMR (CDCl3, 63 MHz), δppm: 71.3 (FeC), 72.2 (Cp), 73.5 (FeC), 77.6 (CC), 126.7 (NCC), 137.2 (NCCC), 148.4 (NC). Infrared: νCC = 2 176 cm–1.
Analysis calcd (%): C 50.57, H 3.15; found (%): C 50.28, H 2.91
5.7 Synthesis of the cluster-cored hexa-tri-silylferrocenylphenolate dendrimer (Bu4N)2[MoBr8(OC6H4C{C3H6SiC2H6Fc}3)6], 9
A 20-ml THF solution of (n-Bu4N)2[MoBr8(CF3SO3)6], (0.019 mmol, 0.050 g) and Na[OC6H4C(C3H6SiC2H6Fc)3] (0.23 mmol, 0.222 g) was magnetically stirred at 60 °C overnight. The solvent was removed under vacuum, and 20 ml of toluene were added. The solution was then stirred at 80 °C for two days, then filtered at room temperature over celite. The volume of the filtrate was reduced to 3-5 ml, and 30 ml of anhydrous pentane was added dropwise. An orange powder precipitated and was re-dissolved with 2-3 ml toluene and re-precipitated with pentane. The compound (Bu4N)2[MoBr8(OC6H4C(C3H6SiC2H6Fc)3)6], 9, is an orange powder. Yield: 57% 0.080 g). 1H NMR (300 MHz, CDCl3): δ 6.85 (m. 12H), 6.45 (m. 12H), 4.12 (s, 12H), 3.91 (s, 30H), 3.86 (s, 12H), 2.72 (m, 16H), 1.40 (m, 36H), 1.07–0.97 (m, 42H), 0.70 (m, 36H), 0.43 (m, 36H), 0.002 (s, 108H). 13C NMR (62.9 MHz, CDCl3) : δ 724.65 (C5H4), 72.28 (C5H4), 69.81 (C5H4), 31.34 (CH2N), 19.73 (CH2), 19.20 (CH2). 15.66 (CH3), -0.08 (CH3). CV of the ferrocenyl system: E1/2 = 0.40 V vs. [FeCp*2] in CH2Cl2 at 20 °C.
Acknowledgment
We are grateful to Dr Jean-Claude Blais (University Paris-6) for recording the MALDI TOF mass spectrum of complex 1. Financial support from the French ‘Ministère de la Recherche’ (ACI Nanostructures 2001 No. N18-01), the ‘Institut universitaire de France’ (IUF), the ‘Centre national de la recherche scientifique’ (CNRS), the Universities Bordeaux-1, Rennes-1 and Funchal (Portugal) and the Fondation Langlois are gratefully acknowledged.