1 Introduction
Cleaning of building surfaces (glass, wall and aluminum cladding) is generally done by using detergents accompanied by scrubbing, wiping and high-pressure water jet. This type of work is labor-intensive, troublesome, costly and consumes a huge amount of energy. Hence, a self-cleaning building surface is highly desirable.
Titanium dioxide (TiO2) is a semiconductor with a band gap energy Eg = 3.2 eV. If this material is irradiated with photons of the energy > 3.2 eV (wavelength λ < 388 nm), an electron is excited from the valence band to the conduction band, leaving a positively charged hole in the valence band. These photoexcited holes and electrons tend to move to the surface to induce oxidation–reduction. For example, the positive hole oxidizes a water molecule (H2O) to a hydroxyl radical (OH•), and the negative electron reduces oxygen to a superoxide anion (O2–) and hydrogen peroxide (H2O2) [2]. As OH•, H2O2 and O2– are very reactive, they trigger further chemical reactions in their vicinity. Based on these phenomena, numerous applications have been proposed, for example, treatment of skin tumors or skin disease [3], killing of bacteria [4], de-odorization [5], anti-fogging [1] and self-cleaning of glass [6]. Among them, self-cleaning for building surface is one of the most attractive applications. In the past, much work has been performed on inorganic substrates such as glass and ceramic tiles due to photocatalytic TiO2's strong reactivity. If photocatalytic TiO2 is directly coated on an organic paint surface, it attacks the organic paint surface resulting in ‘paint-chalking’ [7,8]. Although work on coating TiO2 onto PET film and PMMA was attempted [9], the application is still very limited since paint is commonly used on building surfaces. In order to widen its application, further research on applying TiO2 coating onto a painted surface is needed.
Here, we designed a way to apply reactive photocatalytic TiO2 to a sol–gel coating in order to achieve a self-cleaning effect for a building. Sol–gel is a process that uses chemical precursors to produce ceramic and glass coatings at low temperatures [10]. These coatings are inert and unaffected by exposure to UV light. Fluorocarbon paint {–(CH2CF2)n–} was chosen for investigation because it is widely used in the high-rise buildings and vehicle industries.
2 Results and discussion
2.1 Synthesis of sol–gel binder
Tetraethoxysilicate (TEOS) and 3-glycidoxypropyl-trimethoxysilane (GPTS) as precursors and itaconic acid as a catalyst were added into a mixture of water and 1-methoxy 2-propanol (MP) for hydrolysis. Separately, GPTS modified silica particles were prepared by adding a few drops of GPTS to colloidal silica suspensions (Ludox™40; 140 m2/g, 10–20 nm in diameter). These two solutions were mixed and stirred for at least 1 week before use. The molar ratio for TEOS/GPTS/SiO2/itaconic acid/MP/H2O is 1.63:1:3.95:0.39:6.41:39.48. At pH 3–4, the epoxy groups of GPTS are opened. The methoxyl groups of TEOS and GPTS are hydrolyzed to form silanol groups (Si–OH). These silanols then condense with each other to produce dimers and larger oligomers [11,12]. Concurrently, hydrolyzed GPTS molecules adsorbed on the surface of silica particle either react with each other (by hydrogen bonding or through condensation reaction) or with hydrolyzed TEOS/GPTS molecules or oligomers in solution to produce a polymeric siloxane network (see Fig. 1b).
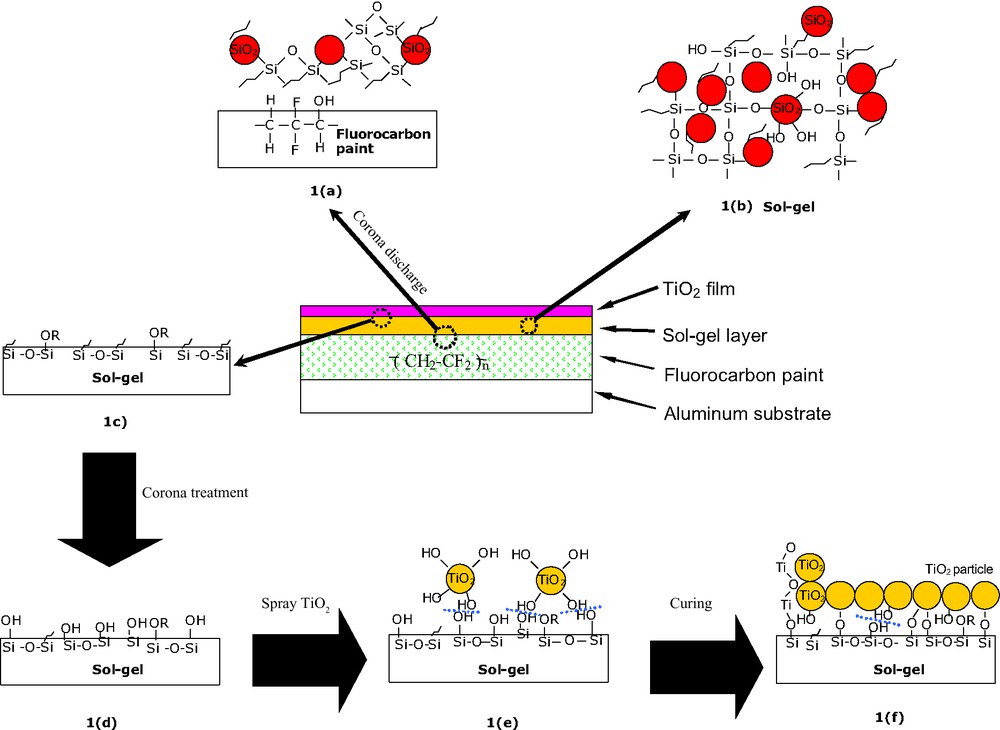
Schematic illustration for TiO2 sol–gel coating in this study. Since corona discharge treatment was used to remove the organic substance before spraying TiO2, the TiO2 particles were probably mainly in direct contact with the silica and siloxane networks.
= The opened epoxy ring on hydrolyzed GPTS.
2.2 Synthesis of TiO2 sol–gel coating onto a paint surface
To form a coating, the fluorocarbon painted substrate was firstly surface-treated with a corona discharge (10 kV, 500 W; SIMTech), and then sprayed with the above sol–gel solution. The spray gun was swept over the painted substrate at 20 m/min, at a distance of 60 cm and a spray rate of 20 ml/min. The required coating thickness was achieved by repeated spraying. After drying at 80 °C for 10 min and corona treatment again, the TiO2 suspension was sprayed on (Anatase form; 10 nm in diameter; dispersed in water at 2%; w/w). Finally, the coatings were heated at 80 °C for 30 min to condense the silanol groups and to fix the TiO2 coating. X-Ray Diffraction (XRD) analysis (Rigaku-Rint 2000 series) showed that there was a relatively stronger signal at a Bragg angle of 25–32° for the final coating (TiO2 sol–gel), showing the TiO2 to be in its anatase form. Compared with previous attempts, where cracking was always a serious problem during drying/calcination [8], the final coating obtained in the present study was crack-free under optical microscopic observation (× 100) (see Fig. 2A). The coating thickness for the sol–gel and TiO2 layer in this case, as measured by scanning electron microscopy (SEM), are 2 and 0.5 μm, respectively (Fig. 3A). As shown in Fig. 3B, energy dispersive X-ray (EDX) line scans of the cross-section (from a→b) confirm the presence of Ti followed by that of Si. Quantitatively, the coating adhesion was tested using the ASTM standard tape test, where no de-lamination or removal was observed. The coating hardness showed at 2H, which is equivalent to the paint's original values (see Table 1).
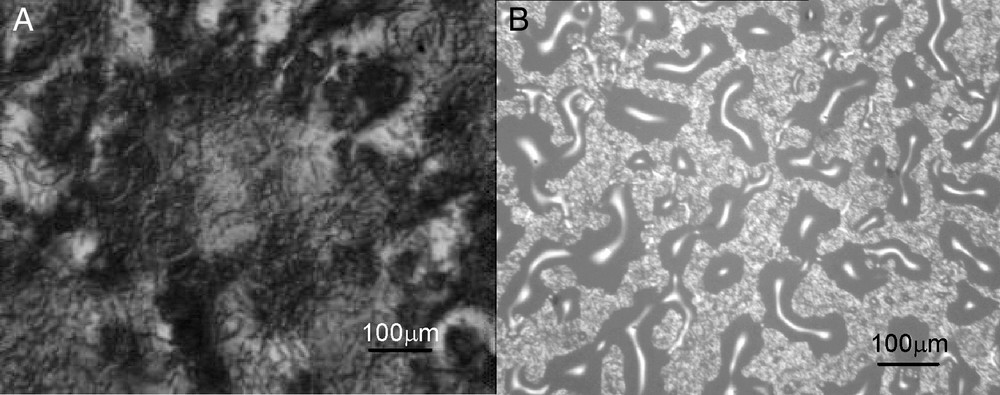
Optical micrographs (100 ×) for various coatings. (A): TiO2 sol–gel coating; (B): TiO2 coating was directly sprayed onto fluorocarbon paint surface without sol–gel binder.

SEM and EDX analysis of cross-section of the hybrid coating system. (A) Scanning electron micrograph of the cross-section of the TiO2 sol–gel coating. (B) EDX elemental line scans for Si and Ti along the line A B in A.
Coating properties after Xenon UV accelerated testing
Coating types | Cracka | Yellowingb ΔE | Gloss c retention | Adhesiond | Hardnesse |
No treatment | None | 1.4 | 99.9% | 5B | 2H |
Sol–gel | None | 1.9 | 102.8% | 5B | 4H |
TiO2f | Crack | 3.3 | 27.6% | Chalking | Unable to measure due to chalking |
TiO2 sol–gel | None | 3.3 | 98.2% | 5B | H |
a Cracks were monitored at suitable intervals using optical microscopy and photographed.
b Results were recorded by Datacolor Microflash Portable Color Spectrophotometer based on the average of six replicates showing coefficients of standard variation. ΔE = (ΔL2 + Δa2 + Δb2)1/2; L, a, b refer to the test values. The measurement angle is 10°.
c Results were generated from Erichsen Pico-Glossmaster 500 based on the average of six replicates. Gloss retention = Gloss before test/Gloss after test.
d According to ASTM D3363-00: 10 horizontal and vertical cuts are made in the coating using a sharp cutter. The resulting cross cut area was covered with an adhesive tape, which is subsequently removed. Adherence is determined by percentage of removed of coating. For examples, 0% → 5B; less than 5% → 4B; 5–15% → 3B; 15–35% → 2B; 35–65% → 1B; Greater than 65 % → 0B.
e According to ASTM D3359-97: The pencil is held firmly against the coating at 45° angle and pushed away from the operator in a 6.5-mm stroke. The hardness of the pencil that will not scratch the coating represents the value of its scratch hardness.
f Xenon UV Accelerated Testing stopped at 40 h because of cracking and lost of gloss.
Compared to those with a sol–gel layer, direct coating of TiO2 onto the paint surface exhibited poor scratch resistance and adhesion. Furthermore, the coating is not continuous (see Fig. 2B), indicating that the sol–gel layer indeed enhances coating adhesion to the painted surface. This phenomenon agrees well with other research reports [13,14], where GPTS served as an adhesion promoter for aluminum and plastic substrates. A possible structure for the TiO2 sol–gel coating in this study is proposed in Fig. 1. The excellent adhesion is likely attributable to the corona treatment and the chemical interaction between the opened epoxy rings from hydrolyzed GPTS and the polymer substrate (1a). Corona treatment increased the surface tension of the coating [15], to permit sufficient wetting (θ: 70° → 50°) of the sol–gel thus enabling direct coating. Without this treatment, wettability is poor and direct coating is not possible. Upon heating at 80 °C, hydrolyzed GPTS and TEOS condensed to form a strong sol–gel layer (Fig. 1b). The residual organic groups from the GPTS and TEOS tend to move to the surface due to its lower surface energy (Fig. 1c) [16]. Upon the second corona treatment, θ was reduced from 50° to 12°, indicating that the surface energy became significantly higher. This is probably because most of the organic groups were decomposed and additional hydroxyl groups were generated during this process, as shown in Fig. 1d. These hydroxyl groups reacted with the TiO2 surface through condensation to form a Si–O–Ti bond or by hydrogen bonding to surface hydroxyls as shown in Fig. 1e and f. Surprisingly, the θ value was reduced almost to zero for the final TiO2 sol–gel coating even without solar irradiation (θ gradually increased in dark conditions, but it quickly dropped again upon solar irradiation, see more in Fig. 5). In contrast to a previous report [1], the components of the sol–gel coating enhance this super-hydrophilic effect (details will be published in another paper). More importantly, compared with the thermal treatment in the range of 400–500 °C to obtain a hard TiO2 film in the past [5], the method used here is suitable for polymeric substrates, and more convenient to implement.
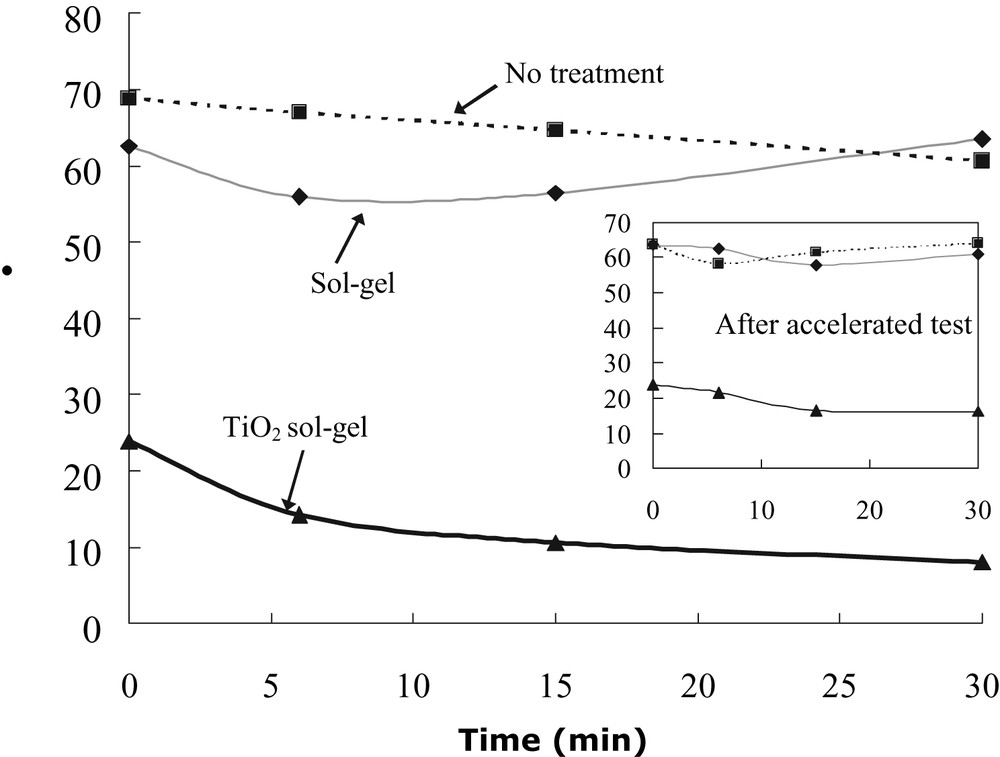
Variation of θ value as a function of solar irradiation for (a): ■ ----- No treatment; (b): ♦ ----- Sol–gel coating; (c) ▲ ------TiO2 sol–gel coating. The inset diagram shows results after Xenon UV Accelerated Testing.
2.3 Self-cleaning effect for the TiO2 sol–gel coating (durability and effectiveness)
To study the long term durability and effectiveness of the TiO2 sol–gel coating, Xenon UV Accelerated Testing was used. This test simulates natural sunlight and rainfall under accelerated conditions (UV A: 85 W/m2; humidity: 75 ± 5%). The exposed samples were examined for damage such as cracks, color changes, and gloss loss, and changes in the self-cleaning effect were investigated. All samples were simultaneously exposed to sunlight in order to account for natural solar fluctuations. The results are summarized in Table 1 and the insets of Figs. 4 and 5.
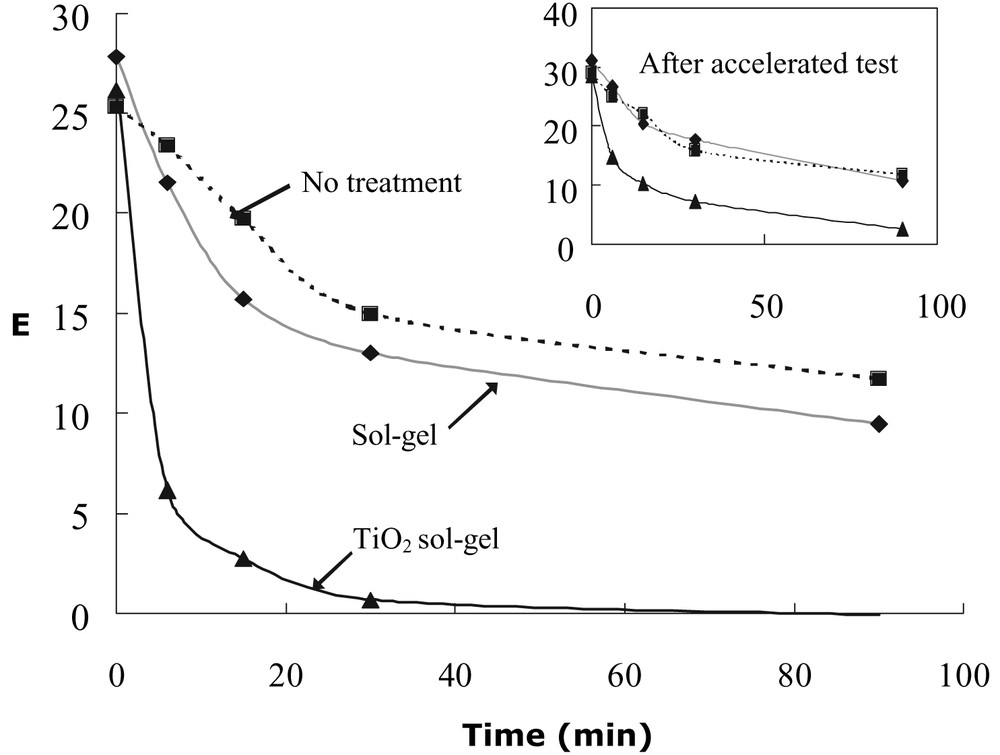
MV, as dirt model, was used to investigate the coating's photocatalytic oxidation ability. Color change (ΔE) is given as a function of time for solar exposure. Coating thickness for TiO2 and sol–gel layer are 0.5 and 2 μm, respectively. The inset diagram shows results after Xenon UV Accelerated Testing.
As seen in Table 1, when the paint was directly coated with TiO2, there were signs of cracks after 40 h of exposure. Gloss retention dropped to 27.6% due to chalking. By contrast, the TiO2 sol–gel layer survived the test for 1000 h (UV dose is roughly equivalent to outdoor exposure for 1.5 years in Singapore, and 3 years in Melbourne) without cracking. There was little change in color (measured as ΔE; see Table 1 for ΔE calculation) or gloss. This indicates that the sol–gel layer is an effective barrier preventing the TiO2 photochemical reaction from attacking the paint surface. Although hardness of the TiO2 sol–gel coating decreased from 2H to H after the accelerated testing, the coating should still be durable enough, as wear is reduced by the decreased maintenance frequency.
The self-cleaning effect was determined by two methods: (1) measurement of the photocatalytic oxidation's ability to destroy a pigment indicator, and (2) measurement of the hydrophilicity, or washing power. An organic pigment, methylene violet (MV; 0.05% aqueous) was used as the dirt indicator. It was sprayed five to seven times on the test samples, followed by direct exposure to sunlight for various durations (11:00–17:00, August 2002; UV with wavelength of 320–400 nm was measured as 2–5 mW/cm2 by using a spectro-radiometer from 4D Controls Ltd. Change in color was then determined through calculation of the ΔE value. The results of the photocatalytic oxidation are shown in Fig. 4, where ΔE is given as a function of time for solar irradiation. For a fresh TiO2 sol–gel coating, there was a rapid decrease in ΔE. For example, 30-min solar irradiation of TiO2 sol–gel samples completely oxidized the MV-indicator, whereas almost half of the original ΔE value still remained on the untreated and sol–gel coated samples. This decrease in ΔE for the untreated and sol–gel samples is attributed to the direct solar UV light effect, but this effect alone is insufficient to remove dirt marks completely. This is especially so when ΔE dropped below 1: MV dirt marks were still discernible even after several days exposure. By contrast, the TiO2 sol–gel coating can generate a surface totally free from dirt marks. It is clear from our data that the TiO2 photocatalytic oxidation is more effective than with solar irradiation alone.
Values of hydrophilicity, measured by water contact angle (θ value), are shown in Fig. 5. As mentioned, θ value for the very fresh TiO2 sol–gel coating was zero. After keeping freshly prepared samples in the dark for 2–3 days, the θ value went up to 23.9°. However, the θ value dropped again simply by solar irradiation. The change in θ value as a function of time is shown in Fig. 5, θ was reduced to 14.2° upon 5 min of solar irradiation, and further decreased to below 10° upon 30 min of irradiation. The coating's θ value was decreased to approximately 2° after exposure to solar irradiation for 2 h, and completely to zero after 1 day outdoor exposure. After the coating was kept in the dark for 12 h, it was confirmed that the θ value could go up 3–5° only. On this basis, we estimate that 6–8 h of solar irradiation per day will be sufficient to maintain the self-cleaning effect through the night. This extremely low θ value makes the surface self-cleaning by the washing effect of rain. By contrast, solar irradiation had little effect on the untreated and sol–gel samples: their θ values still remained at 60–70° levels although they received the same period of solar irradiation. Indeed, the synthesized TiO2 sol–gel coating showed both excellent photocatalytic and hydrophilic effects, whereas a previous study on glass shows that its hydrophilicity was independent of its photocatalytic activity [1].
The long-term self-cleaning effectiveness of the TiO2 sol–gel coating after Xenon UV Accelerated Testing, as measured by its ability to destroy color pigment and reduction of water contact angle upon brief solar exposure, is shown in the insets of Figs. 4 and 5. It can be seen that the long term self-cleaning effectiveness is very close to that obtained before UV accelerated testing. For example, the photocatalytic oxidation effect for the TiO2 sol–gel coating is stronger than those of the untreated and sol–gel samples. The water contact angle, θ, remained at 0° after immediate Xenon UV Accelerated Testing. It went up to 24° after being kept in the dark for 1 week. Upon solar irradiation, θ values were decreased readily, as shown in the inset of Fig. 5. After 30-min of solar irradiation, TiO2 sol–gel coating produced an excellent hydrophilic surface with θ values at 12.7°. The θ values ultimately decreased to zero after sufficient sunlight exposure. By contrast, there was practically no change in the untreated samples and sol–gel samples; θ values still remained in the range of 65–70°. Compared with samples before accelerated testing, it can be seen that the photocatalytic oxidation rate and the θ values decreased somewhat after the accelerated testing. For instance, the θ value for TiO2 sol–gel coating was about 7° higher with similar 30 min sunlight exposure. Research is currently in progress to elucidate and minimize this phenomenon. Nevertheless, the θ value is still much lower compared with those of untreated and sol–gel samples.
Currently, long term field testing of panels with TiO2 sol–gel coatings is on-going. Continuous and sustained self-cleaning effects were realized as seen in Fig. 6 after 10 months of outdoor exposure. A Dirt Collection Index1 was used to express the dirt accumulation level. The lower of Dirt Collection Index, the more dirt is accumulated on the surface. The Dirt Collection Index was greatly decreased from 100 to 95 for the untreated samples, and slightly decreased from 99 to 98 for the TiO2 sol–gel coating over the same period. This data again indicates that the coating is self-cleaning.
3 Conclusions
We have described a new route to form a solar-driven self-cleaning coating on a painted surface, through a combination of corona treatment, sol–gel coating and a photocatalytic TiO2 layer. Using this approach, a strong and crack-free TiO2 sol–gel coating was obtained. The very fresh TiO2 sol–gel coating shows almost zero water contact angle even without exposure to sunlight, and this super-hydrophilic property is retained under sunlight irradiation, differentiating our approach from other study.
In the absence of the binder layer, there was rapid oxidation after only 40-h of Xenon UV accelerated testing; while the hybrid TiO2 sol–gel coating could last for 1000 h without any trace of cracking, and with minimal yellowing and loss of gloss. It was observed that pigment adsorbed on the surface was completely destroyed by only 30-min of solar irradiation. Simultaneously, solar exposure rendered the surface extremely hydrophilic as measured by the drastic reduction of water contact angle (θ) from 70° to 2°. With such a highly hydrophilic property, dirt residues on the surface were readily washed away by rain. Both photocatalytic and hydrophilic effects were retained after the UV accelerated testing, suggesting that the novel coating possesses solar driven, self-cleaning capability. Furthermore, the method developed in this work should be applicable to other organic surface.
Acknowledgements
This work was conducted under support provided by Singapore Agency for Science, Technology and Research (A-STAR) under Grant 172/2/10-11V1.
1 Dirt Collection Index refers to the collection of foreign matter that darkens the surface of exterior exposed coated panels. Lightness readings, L* values, were measured by using a colorimeter or a spectrophotometer before and after exposure According to the Standard Test Method for Quantifying Dirt Collection on Coated Exterior Panels D 3719-95, the Dirt Collection Index, Dc, was computed as:
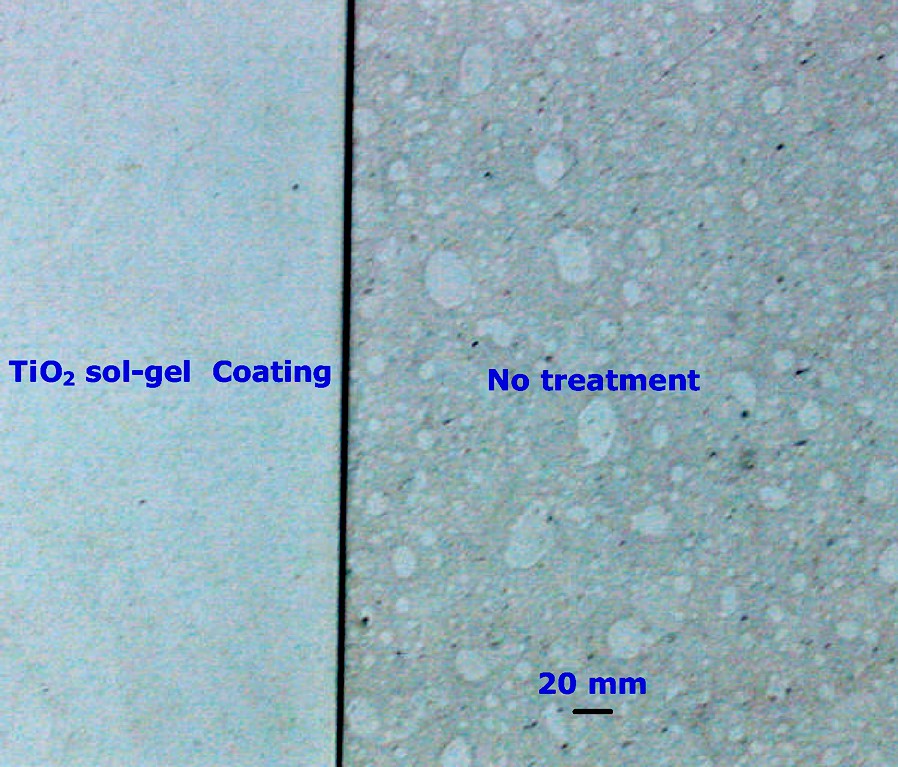
Vous devez vous connecter pour continuer.
S'authentifier