1 Introduction
Many types of photocatalytic reactors have been proposed for water purification. However, most of reactors use UV lamp that consume an electric power. Activation of photocatalyst by sunlight can decrease the cost for the system. Although some of photocatalytic reactor using sunlight for activation of photocatalyst has been developed, they also require the electricity for circulation of water by pump and so on [1]. The purpose of this research is a development of drinking water purification system using less electricity. This system basically aims at the utilization in isolated islands, mountain huts, and inconvenient places in developing countries. Especially, it is expected that this system will be helpful in developing countries located in tropical or sub-tropical area since enough sunlight can be obtained. Using this water purification system, a lot of people can get purified drinking water and hence can prevent suffering from diseases and improve their health.
So far, using optical fiber coated with TiO2 has carried out a number of researches on water purification by using optical fiber coated with TiO2. Two types of optical fiber were used as a substrate for TiO2 coating. One is quartz fiber, and the other is the polymer optical fiber [2]. The quartz fiber is very stable and the transparency of UV light is high, however, it has a costly problem. Typical polymer optical fiber is made from polymetylmethacrylate (PMMA) resin. This is not so expensive compared with quartz fiber [3], however, this organic fiber is not stable against photocatalytic oxidation.
In this research, we used optical wave-guide rod instead of optical fiber to avoid for mentioned costly and instability problems for optical fibers [5]. Transparent TiO2 thin film was coated outside of Pyrex glass tube, and the optical wave-guide rod made from PMMA was inserted into this photocatalytic tube. Pollutants are oxidized at the interface between photocatalytic surface and aqueous phase by UV light irradiation of photocatalytic layer from the backside. Here, we report some results about the removal rate of water soluble VOC by the reactor using wave-guide type photocatalytic rods. Final destination of this research is not only removal of organic water pollutant but also sterilization of bacteria in drinking water.
2 Experimental
2.1 Preparation of wave-guide type photocatalytic rod with TiO2
A glass tube with transparent TiO2 was prepared by dip-coating method [4]. Tetraisopropyl orthotitanate (TPOT), α-terpineol (TPO), 2-propanol (PrOH), diethyleneglycol monoethyl ether ((ethoxyethoxy)ethanol, EEE) were used to make a solution for dip-coating. EEE was used as ligand for TPOT. TPOT (100 ml), TPO (350 ml), PrOH (250 ml), and EEE (100 ml) were well mixed using a magnetic stirrer before use.
A Pyrex glass tube (external diameter = 9.5 mmϕ, internal diameter = 6.5 mmϕ, length = 150 mm) was dip-coated as follows. Withdrawing speed was 1.5 mm/s, and dip-coating process was carried out under room temperature and ambient air. After that, this glass tube was heated at 450 °C for 1 h to form anatase TiO2 on the surface. To examine the effect of TiO2 film thickness, four kinds of glass tube were prepared with different coating times, 3, 6, 9, and 12. Twelve times coating means repeating 12 times, dip-coating and calcination process. TiO2 film thickness on the glass tube was estimated from field emission scanning electron microscope (FE-SEM) images taken using Hitachi S-4700.
We used transparent acrylic acid resin rod (diameter = 6 mm, length = 150 mm) as a wave-guide for the incident light. Two types of acrylic wave-guide rods were prepared for the purpose of increasing seep out of the light. One of the wave-guide rod had 50 mm of frosted part at the edge, and the other had a screw-cut by stump out using a die.
The wave-guide type photocatalytic rod was prepared by inserting the above wave-guide rod into the photocatalytic tube coated with TiO2. Sixteen photocatalytic rods were set at vertical position on a Teflon block plinth (50 × 50 × 10 mm) and a photocatalytic unit was constructed. The image of the unit is shown in Fig. 1.
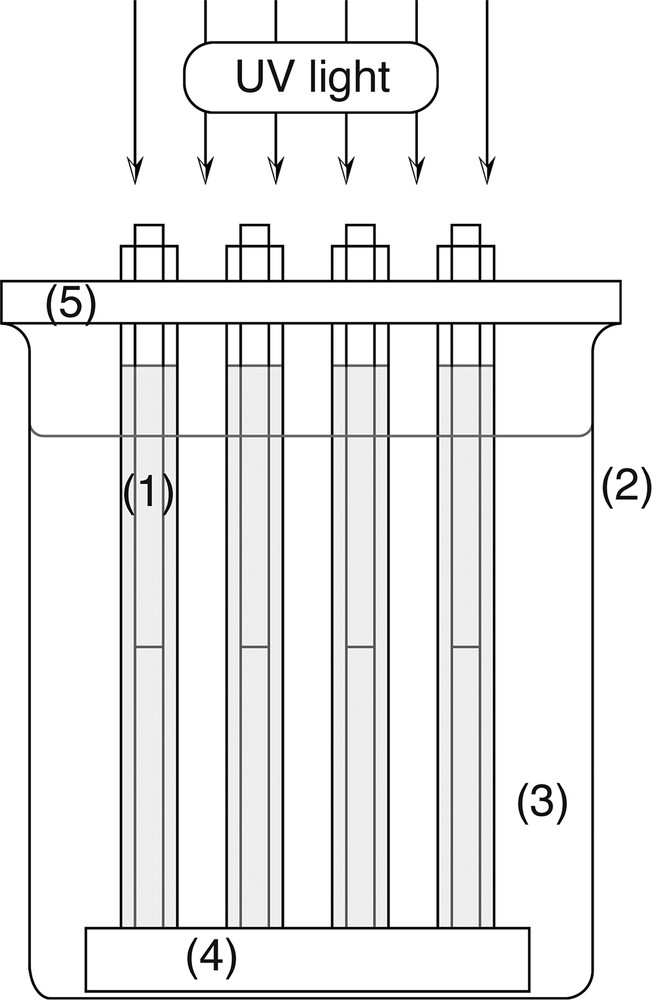
Image of photocatalytic reactor using wave-guide rods. (1): photocatalytic rod, (2): 500 ml beaker, (3): polluted water, (4): Teflon plinth, (5): Teflon cap.
2.2 Measurement of UV light intensity from wave-guide type photocatalytic rod
The efficiency of water purification depends on UV energy used for oxidation reaction. UV light transmitted inside of the wave-guide mainly seeps out from the frosted or screw-cut section and irradiates the TiO2 layer from the backside. To obtain an information about suitable TiO2 film thickness, we measured the intensity of UV light transmitted from the side of each wave-guide inserted photocatalytic rod in the atmosphere. The measurement of the UV intensity emitted from wave-guide type photocatalytic rod was carried out using Fuji Xerox UV Caremate Pro.
2.3 Photocatalytic reactions
The unit of wave-guide type photocatalytic rods prepared in Section 1 was set on a 500 ml glass beaker and used for photocatalytic decomposition of pollutants in a model of polluted solution (500 ml) in a beaker. Toluene and phenol were added as contaminants for a model solution, and the initial concentration was kept at 100 ppm. Top of beaker was sealed with Teflon plate to avoid direct irradiation of water by the UV light source. Irradiation of light was carried out using high-pressure mercury lamp (500 W). The experimental apparatus used in this research is shown in Fig. 2.
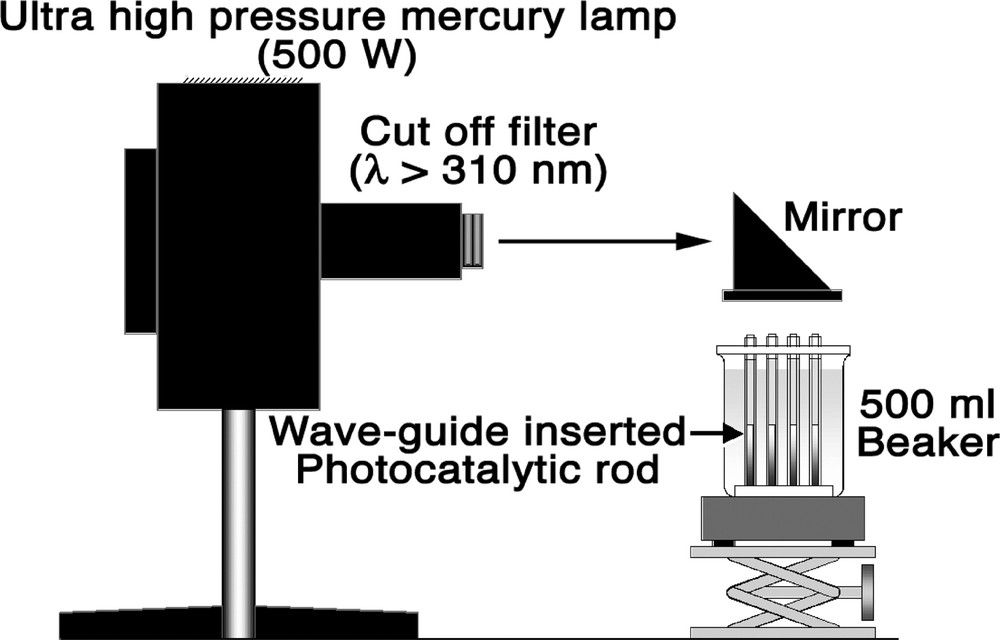
Experimental apparatus for photocatalytic reaction.
Concentration of contaminants and intermediates formed by oxidation reactions was analyzed using HPLC equipped with Shimadzu LC10AC gradient system and GC/MS (Shimadzu GCMS-QP2010).
3 Results and discussion
The method of TiO2 coating was already reported [4]. For the TiO2 coated glass tubes, optical measurement of film thickness was difficult because the shape of TiO2 layer was cylindrical. Therefore, film thickness was estimated by observation of cross section of the glass tube by using FE-SEM. Fig. 3 shows the SEM image of the cross section of the TiO2 coated photocatalytic rods. As shown in Fig. 3, the thickness of TiO2 layer linearly increased in proportion to the number of dip-coating. Estimated film thickness of the TiO2 layer with 3, 6, 9, and 12 time coating were around 0.25, 0.50, 0.75, and 1.00 μm, respectively.
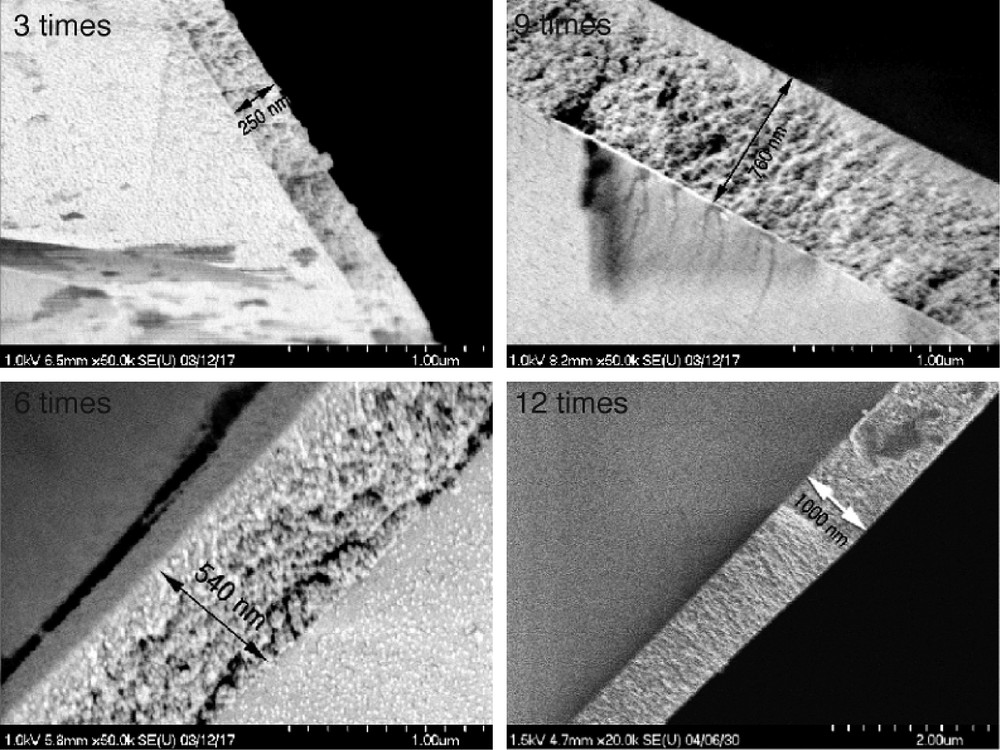
FE-SEM image of the cross section of a glass tube with TiO2 coating.
The thickness of TiO2 layer linearly increases with increasing dip-coating times.
Fig. 4 shows the intensity of UV light seeped out from the photocatalytic rod. 10 cm on a glass tube was coated by TiO2 thin film. As shown in Fig. 4, seeping out of light from the top 5 cm of the photocatalytic rod is very weak because introduced light is totally reflected at the wall of the wave guide. Introduced UV light into wave-guide rod is seeped out from the bottom 5 cm of photocatalytic rod. Furthermore, UV intensity depends on a difference process of seep-out region between frosted and screw-cut, and film thickness of TiO2. Maximum UV emission intensity from screw-cut wave-guide inserted photocatalytic rod was around five times of frosted one. Emission from seep out area is depend on a roughness of wave-guide surface. This result shows that an important factor for this system is how to seep out the introduced light to wave-guide rod. Seeping out UV intensity from wave-guide inserted photocatalytic rod decreased with the increase of film thickness as shown in Fig. 4. This result means that absorbance of UV light by TiO2 layer increases with increasing TiO2 film thickness.
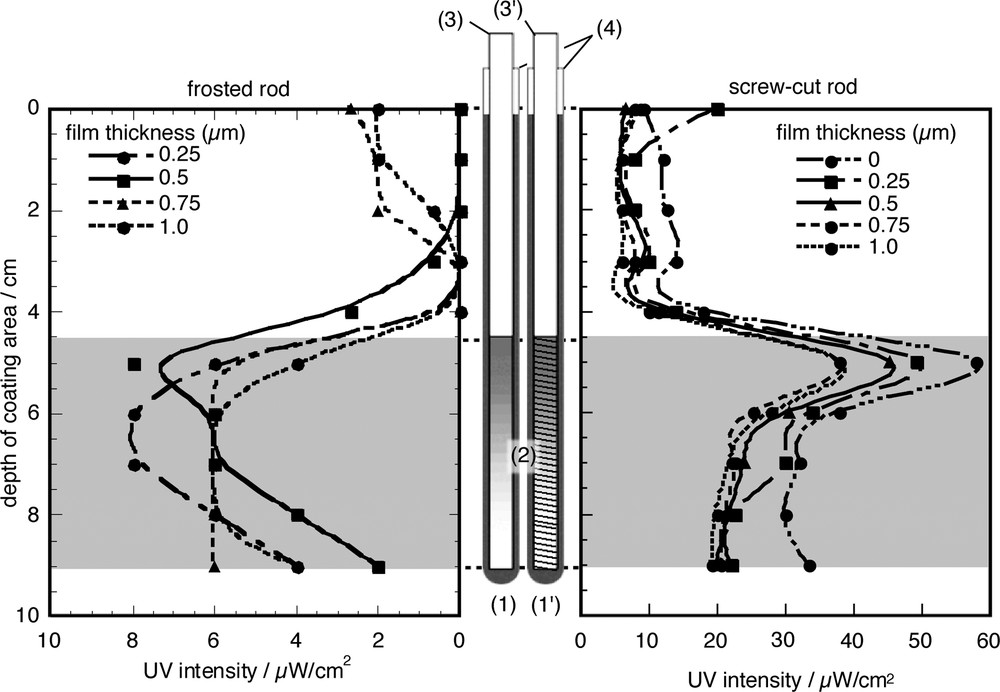
Dependence of coating times on UV intensity seeped out from the photocatalytic rod. Left side shows the light intensity from frosted wave-guide inserted photocatalytic tube. Right side shows the light intensity from screw-cut wave guide inserted photocatalytic tube. (1) Shows the image of the frosted wave-guide inserted photocatalytic tube. (1′) Shows the image of the screw-cut wave-guide inserted photocatalytic tube. (2) Shows the seep-out region of UV light from frosted or screw-cut processed on wave-guide rod. (3) Shows the frosted wave-guide rod. (3′) Shows the screw-cut wave-guide rod. (4) Shows the photocatalytic tube. Masquer
Dependence of coating times on UV intensity seeped out from the photocatalytic rod. Left side shows the light intensity from frosted wave-guide inserted photocatalytic tube. Right side shows the light intensity from screw-cut wave guide inserted photocatalytic tube. (1) Shows ... Lire la suite
Fig. 5 shows a relationship between TiO2 film thickness and penetration of UV light into TiO2 layer. In this experiment, UV irradiation of TiO2 photocatalyst is backside direction of coating. Therefore, TiO2 film thickness is also important factor for reveal of photocatalytic activity. When TiO2 film thickness is too thin (case (1)), photocatalytic activity is low because absolute amount of photocatalyst usable for photodegradation is small. Similarly, photocatalytic activity may decrease when TiO2 film thickness is too thick (case (3)). Inside of TiO2 layer absorbs UV irradiation, and the UV light approached to the photocatalytic area in TiO2 layer must be decreased, as a result, photocatalytic activity is decreased. At the case (2), excitation light is strong at the photoreaction area. In addition, the amount of photocatalyst is optimum. Therefore, it is expected that photocatalytic activity is high on the case (2).
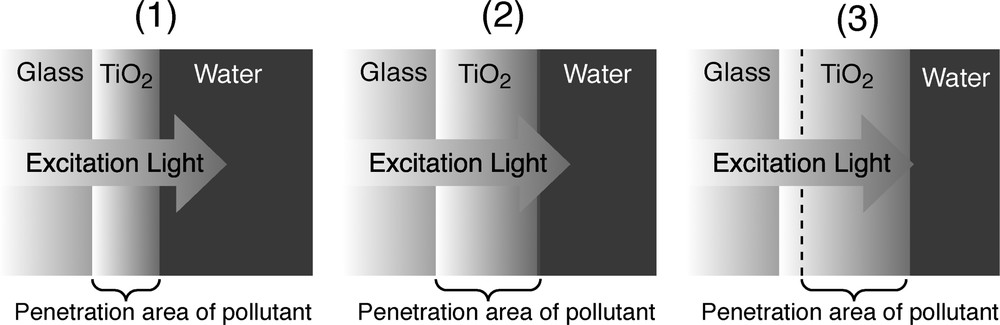
Relationship between TiO2 film thickness and the light penetration into TiO2 layer from backside. (1) Too thin TiO2 layer, (2) optimum TiO2 layer, (3) too thick TiO2 layer. TiO2 layer has a porous structure, and reactants penetrate and disperse to inside of the TiO2 layer.
Fig. 6 shows the result of photocatalytic degradation of 100 ppm toluene in aqueous phase using wave-guide type (with screw-cut rod) photoreactor. Toluene easily evaporates from aqueous phase, therefore, the changes in toluene concentration by evaporation was obtained and compensated for the true amount of degraded toluene by photocatalyst. CO2 and H2O have been reported as the final products of the photocatalytic degradation of toluene [5]. In case of gas phase reaction, benzyl alcohol and benzaldehyde were observed as adsorbed intermediate species on a surface of photocatalyst [5]. Although these two intermediate species are soluble in water, we could not detect them in aqueous phase. On the other hand, we found small amount of p-cresol in aqueous phase. The mechanism for following photodegradation of toluene has been proposed (Schema 1).
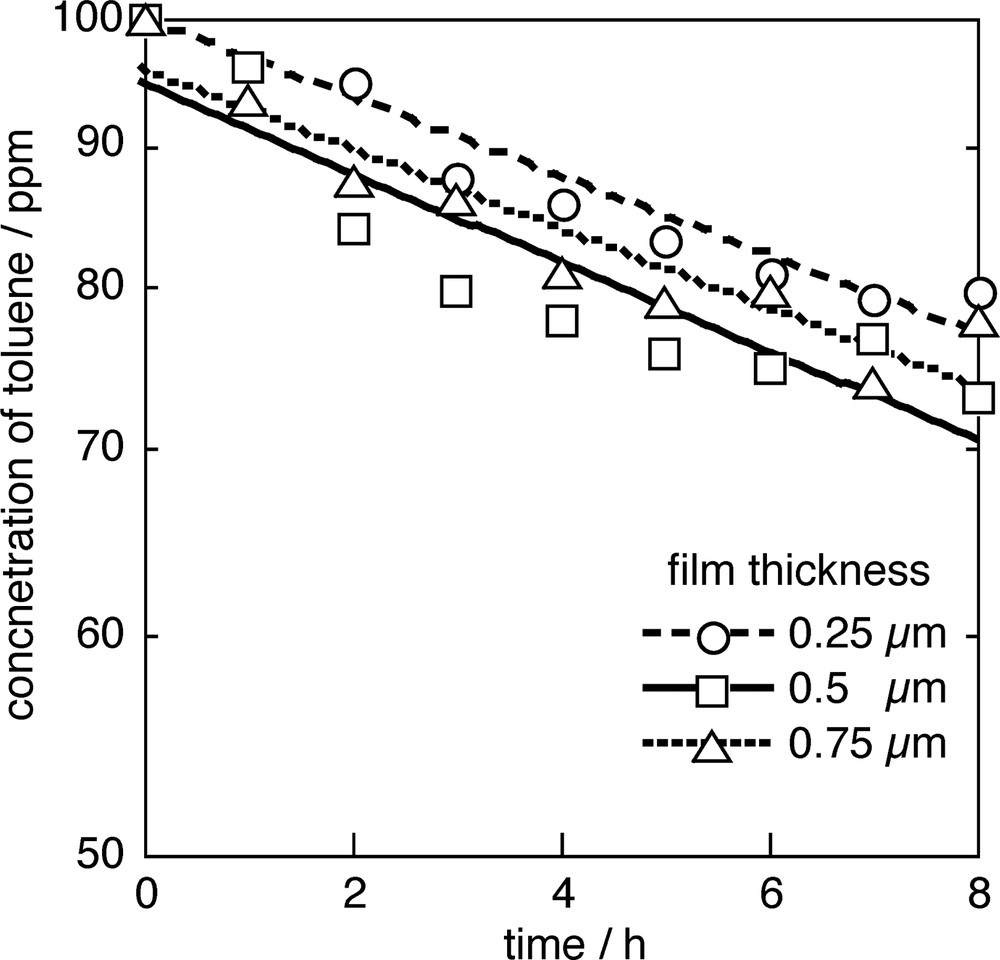
Photocatalytic degradation of toluene (100 ppm). The reaction rate depend on a thickness of the TiO2 layer.
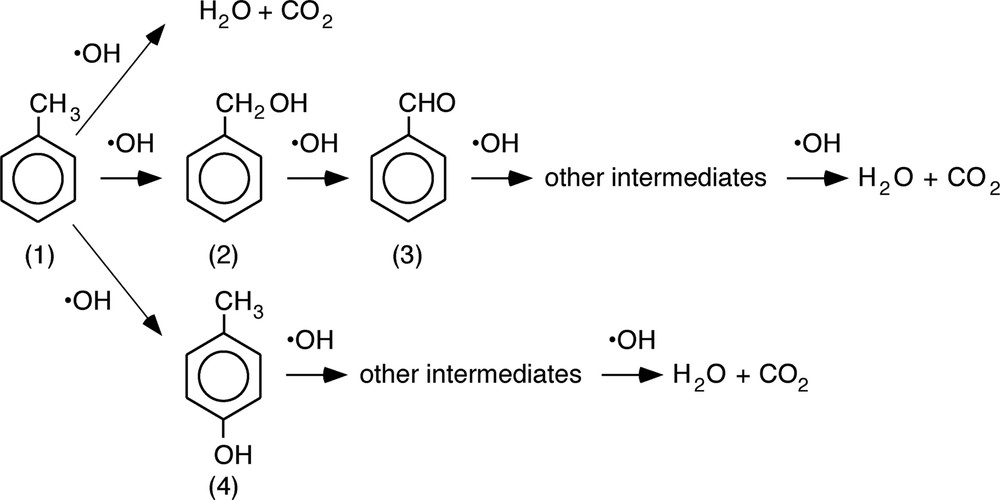
Proposed degradation pathways of toluene. (1): toluene, (2): benzyl alcohol, (3): benzaldehyde, (4):p-cresol.
OH radical plays an important role for photo-oxidation of toluene and further reaction of intermediates. Many researchers reported that benzyl alcohol (2) and benzaldehyde (3) were formed with photocatalytic oxidation of toluene in gas phase [5]. On the other hand, Marci et al. reported that p-cresol (4) was found in aqueous phase [6]. They supposed that high concentration of water would favor the attack of OH radical to the 4-position of toluene molecule. This result indicates that addition reaction of hydroxyl group to benzene ring easily progress compared with oxidation of methyl group under water rich condition. Therefore, benzaldehyde and benzyl alcohol were scarcely observed in aqueous phase in our study.
As shown in Fig. 6, 0.5 μm thick photocatalytic rod gives the highest efficiency. Namely, 0.5 μm of TiO2 film thickness is suitable for the utilization of wave-guide type photocatalytic rod. This result is agree with forecast in Fig. 5. Film thickness of 0.5 μm corresponds to the case (2) in Fig. 5, which gives optimum penetration of light for photodegradation using the wave-guide type photocatalytic rods.
Phenol is well known environmental contaminant, and it is contained in the waste water from industries. Photodegradation of phenol in aqueous phase has been reported by many researchers. The possible pathway for the photodecomposition of phenol has been reported as follows: (Schema 2).

Proposed degradation pathways of phenol. (5): phenol, (6): catecol, (7): resorcinol, (8): hydroquinone, (9): p-benzoquinone, (10): pyrogallol, (11): phloroglucinol dihydrate, (12): phloroglucinol.
In the case of this photoreactor system, the concentration of phenol did not change with increasing time without UV irradiation.
Fig. 7 shows the result of photodegradation of phenol using the wave-guide type rods. In this experiment, we tested both frosted and screw-cut wave-guide rods. As shown in Fig. 7, concentration of phenol in water decreased with increasing UV irradiation time. The degradation rate depends on the shape of wave-guide and the thickness of TiO2 layer. Any big difference in the degradation rates was observed between frosted and screw-cut wave-guide made with 0.75 μm TiO2 coatings. While degradation rate for screw-cut wave-guide was higher than that of frosted one in case 0.5 and 1.0 μm coated rods were used. Screw-cut wave-guide may allow higher transmittance of light and gives similar penetration of light to the case (2) in Fig. 5. More experiments are needed to reveal why 0.75 μm TiO2 coating gave the best result in case of phenol.

Photocatalytic degradation of phenol (100 ppm) using photocatalytic rods with different thickness of TiO2 layer (0.5, 0.75, 1.0 μm) and different kind of wave-guide rods (screw-cut: S, frosted: F).
In our experiment, we detected few ppm level of some intermediate species formed by photodecomposition of phenol [7]. Judging from the result of HPLC analysis, main products were hydroquinone (8) in Schema 2. In addition, trace level of catecol (6), resorcinol (7), and p-benzoquinone (9) was observed by GC/MS analysis. Trihydroxybenzene isomers (10) and (11) were also observed under trace level. However, phloroglucinol (12) was not observed in any analysis method. It is considered that compound (12) is unstable in an aqueous phase. Detection of these trihydroxybenzenes formed in the aqueous system was very difficult since the concentration of dihydroxybenzenes derived from phenol was also very low.
We conclude that total amount of intermediates can be decreased by use of the wave-guide type photocatalytic rods reported here. Photocatalyst on the glass tube seems to depress the dispersion of intermediates to the aqueous phase. Our result shows that the photoreactor using wave-guide rod inserted photocatalytic tube is more suitable than conventional ones for the water purification. We will continue further experiments for practical application of this system with solar light. Measurement of sterilization effect will be also conducted.