1 Introduction
Oxidation catalysts based on isolated iron active sites, whether mono- or dinuclear, have become a major research interest in the various fields of catalysis (bio- [1,2], homogeneous [3–8] or heterogeneous [9–12]), because they can typically employ alternative oxidation agents such as O2 or N2O in place of alkylhydroperoxide or even H2O2. For instance, the selective oxidation with N2O of benzene into phenol and propene into propene oxide has been reported when Fe-based zeolitic materials [9,10] and a Fe-based silica supported system are used, respectively [13–17]. One of the major debates in this area has been the structure of the active sites, which is still unknown. However, the current models involve typically either isolated mono- or dinuclear Fe systems [18] as in enzymes. In order to evaluate the stability of mono- vs. binuclear surface Fe species, we have therefore studied via Surface Organometallic Chemistry [19] the reaction of analogous mono- and dinuclear perhydrocarbyl Fe(II) complexes [20], namely {[Fe{C6H2(tBu)3}2], (1, C36H58Fe)} [21] and {[Fe{μ1-C6H2(CH3)3}{μ2-C6H2(CH3)3}]2, (2, C36H44Fe2)} [22], with oxide supports {silica (SiO2-(700)), silica–alumina (Al2O3–SiO2-(500)) and alumina (Al2O3-(500))}, which typically gives the corresponding monografted complexes [19].
2 Results and discussion
2.1 Grafting of {[Fe{C6H2(tBu)3}]2, 1} and {[Fe{μ1-C6H2(CH3)3}{μ2-C6H2(CH3)3}]2, 2} on SiO2-(700)
IR spectroscopy. After immersing a silica disk partially dehydroxylated at 700 °C (SiO2-(700)) in a yellow solution of Fe[C6H2(tBu)3]2 (1) in pentane at 25 °C (1.1 mol of 1 per mole of accessible surface silanols), the silica disk turned yellow; it was washed with pentane to remove the excess of the unreacted complex and then dried under vacuum (10−5 Torr, 2 h). Monitoring the reaction by IR spectroscopy showed that the band attributed to isolated silanol groups at 3747 cm−1 disappeared (Fig. 1A), while two new groups of bands appeared in the 3000–2850 cm−1 and 1470–1350 cm−1 regions. These bands are assigned to ν(CH) and δ(CH) vibrations of the alkyls groups, respectively. Additionally, two other bands appeared at 3065 cm−1 and 1593 cm−1, which have been, respectively, assigned to ν(C–H) and ν(CC) vibrations of the aromatic moieties. Similarly, the IR spectra corresponding to the grafting of 2 (Fig. 1B) show that the band attributed to isolated silanols at 3747 cm−1 has totally disappeared, while two groups of bands have appeared in the 3000–2850 cm−1 and 1470–1350 cm−1 regions. These bands are assigned to ν(CH) and δ(CH) vibrations of the residual alkyl groups of the surface of iron complexes, respectively. Two other bands have also appeared at 3025 cm−1 and 1603 cm−1, which are assigned to ν(C–H) and the ν(CC) vibrations of the aromatic moieties, respectively. Both systems display similar IR data, which are in good agreement with a chemical grafting of 1 and 2 on SiO2-(700).
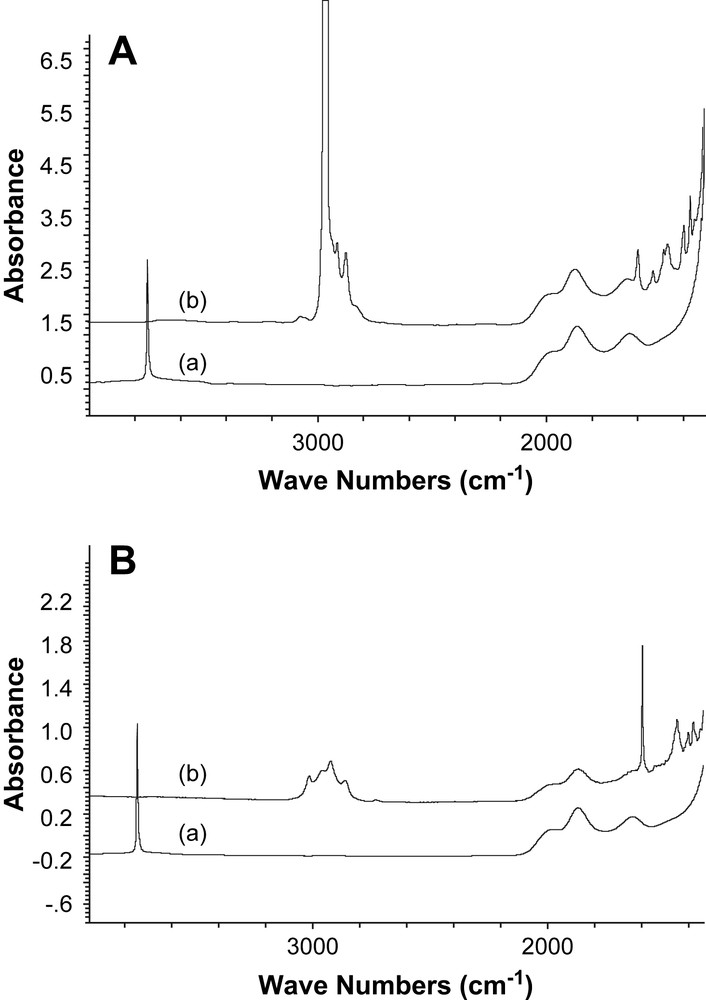
Monitoring by IR spectroscopy of the grafting of 1 (A) and 2 (B) on a silica partially dehydroxylated at 700 °C (SiO2-(700)): (a) SiO2-(700) pellet (50 mg) and (b) after impregnation of 1 or 2 followed by three washings (30 min at 25 °C, in pentane for 1 and in toluene for 2) and a drying step under vacuum (1.32 × 10−3 Pa, 1 h, 25 °C).
Mass balance analysis. Grafting was also performed by impregnation on larger quantities of SiO2-(700) (0.26 mmol OH/g of SiO2-(700)) (Table 1). In the case of 1, iron elemental analysis is ca. 1.20–1.23%wt, which corresponds to 0.21–0.22 mmol Fe/g and an average of 0.88–0.89 Fe/SiOH, in agreement with the consumption of most surface hydroxyls. During grafting, 0.90 ± 0.1 mol of 1,3,5-tris(tertiobutyl)benzene (Mes∗H) per mole of grafted Fe have been released. Moreover, carbon and hydrogen elemental analyses of [1/SiO2-(700)] gave 3.93%wt and 0.56%wt, respectively, which correspond to 15 C/Fe and 25 H/Fe. All these data are consistent with the selective formation of 3 [(SiO){Fe(C18H29)}] as the major surface species, for which 1 Mes∗H evolved during grafting, and 18 C/Fe and 29 H/Fe are expected by elemental analysis (Scheme 1).
Grafting of 1 and 2 on SiO2-(700)
Entry | Precursor | Supporta/mmol | FeR2b/mmol | %wt Fe | Fe/SiOH | RHc/mmol | RH/OHd |
1 | 1 | 0.195 | 0.21 | 1.23 | 0.89 | 0.19 | 0.97 |
2 | 1 | 0.195 | 0.23 | 1.20 | 0.88 | 0.19 | 0.97 |
3 | 2 | 0.105 | 0.11 | 2.33 | 1.75 | 0.096 | 0.92 |
a Number of mmol of OH based on 0.26 mmol of OH/g for SiO2-(700).
b Number of mmol of Fe molecular precursor.
c Number of mmol of RH evolved during grafting.
d Ratio of alkane RH released/surface OH.

Proposed structure of the major grafted surface species resulting for grafting of 1 on SiO2-(700).
In the case of 2, iron elemental analysis on the resulting solid is 2.33%wt, which corresponds to 0.42 mmol Fe/g of silica, i.e. to an average of 1.75 Fe/SiOH. During grafting, 0.57 ± 0.10 mol of mesitylene (MsH) per mole of grafted Fe were released, which corresponds to 1.14 ± 0.20 mol of MsH per mole of grafted 2. Therefore, only one mesityl ligand of 2 has been cleaved during grafting, which is consistent with the formation of [(SiO){Fe2(C9H11)3}] 4 as the major surface species (Scheme 2). The amount of MsH obtained (0.24 mmol/g) and the iron elemental analysis (0.21 mmol of 2/g) show that 0.21–0.24 mmol of surface silanols have been consumed (81–92% of starting silanols based on 0.26 mmol OH/g of SiO2-(700)), which is consistent with what has been observed by in situ IR experiments (vide supra). Carbon and hydrogen elemental analyses of [2/SiO2-(700)] are 5.5%wt and 0.51%wt, respectively, which corresponds to 11 ± 1 C/Fe and 12 ± 1 H/Fe. These data are also consistent with the formation of monosiloxy complexes 4 as major surface species (expected C/Fe and H/Fe ration for 4: 13.5 and 16.5, respectively). Note that the reaction of 2 with a bulky phenol, 2,6-di-tbutyl-4-methylphenol, gives 5 in which only the terminal mesityl ligands have been exchanged (Eq. (1)) [23]. Therefore, we propose that 4a is the most likely surface species upon grafting of 2 on SiO2-(700) (Scheme 2).
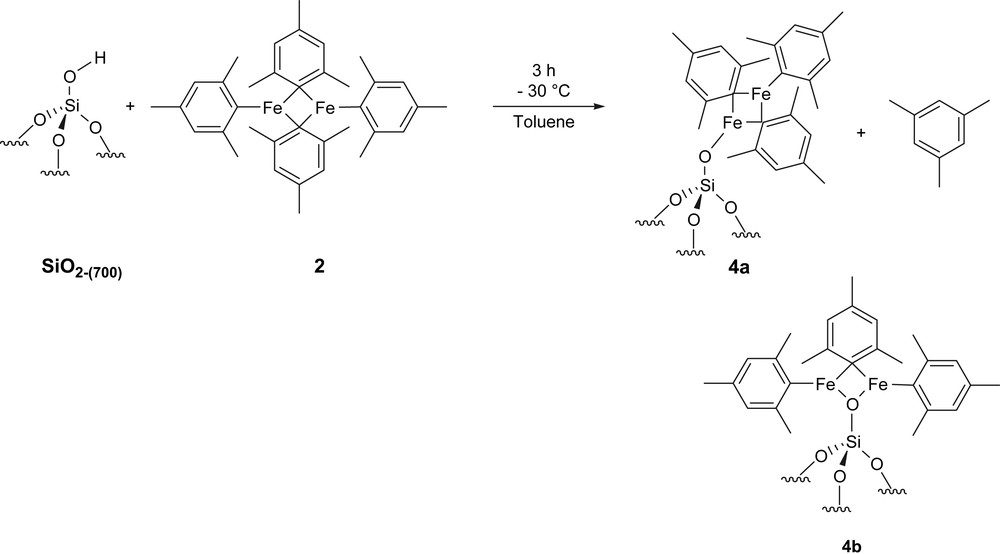
2.2 Grafting of 1 and 2 on SiO2–Al2O3-(500)
First, monitoring the grafting of 1 and 2 by IR spectroscopy shows that it is very similar to what has been observed on silica: most hydroxyl groups reacted and some are not consumed, but interact with adjacent hydrocarbyl ligands (Fig. S1), as evidenced by the broadening and the shift of the hydroxyls to lower wavenumbers [24]. For the grafting of 1 on SiO2–Al2O3-(500) (Table 2, entry 1), iron elemental analysis on the resulted solid is 3.21%wt, which corresponds to ∼0.57 mmol Fe/g, thus showing that there is an average of 0.80 Fe/SiOH. This low ratio is consistent with the presence of remaining OH group, as observed by IR spectroscopy. Additionally, during grafting, 1.0 ± 0.1 mol of 1,3,5-tris(tertiobutyl)benzene (Mes∗H) per mole of grafted Fe have been released. Moreover, carbon (13.5%wt) and hydrogen (1.95%wt) elemental analyses on [1/SiO2–Al2O3-(500)] correspond to 19 C/Fe and 33 H/Fe, respectively. Because hydroxyl groups on silica–alumina are mainly silanols, these data are consistent with the formation of 6 [(SiO){Fe(C18H29)}] as the major surface species, for which as expected 1 Mes∗H/Fe evolved during grafting as well as 18 C/Fe and 29 H/Fe ratio from elemental analysis (Scheme 3a). In the case of 2, iron elemental analysis on the resulting solid is 5.29%wt, which corresponds to ∼0.97 mmol Fe/g, thus showing that there is an average of 1.4 Fe/SiOH (Table 2, entry 2). During grafting, 0.53 ± 0.1 mol of mesitylene (MsH) per mole of grafted Fe have been released. Moreover, carbon elemental analyses of [2/SiO2–Al2O3-(500)] are 16.20%wt, which corresponds to 14.0 C/Fe. All this data are consistent with the selective formation of 7 [(SiO){Fe2(C9H11)3}] as the major surface species, for which as expected 1 MsH evolved during grafting and 13.5 C/Fe from elemental analysis (Scheme 3b).
Grafting of 1 and 2 on SiO2–Al2O3-(500)
Entry | Precursor | Supporta/mmol | FeR2b/mmol | %wt Fe | Fe/SiOH | RHc/mmol | RH/OHd |
1 | 1 | 0.22 | 0.26 | 3.21 | 0.80 | 0.14 | 0.64 |
2 | 2 | 0.22 | 0.26 | 5.29 | 1.4 | 0.13 | 0.59 |
a Number of mmol of OH based on 0.88 mmol of OH/g for SiO2–Al2O3-(500).
b Number of mmol of Fe molecular precursor.
c Number of mmol of RH evolved during grafting.
d Ratio of alkane RH released/surface OH.

Reaction of 1 (a) and 2 (b) with the surface hydroxyls of a silica–alumina surface (MS = Si or Al).
2.3 Grafting of 1 and 2 on Al2O3-(500)
First, monitoring the grafting of 1 and 2 on alumina by IR spectroscopy shows that it is very similar to what has been observed on silica–alumina: some hydroxyl groups react with the Fe molecular complexes, and some are not consumed, but only interact with adjacent hydrocarbyl ligands, as evidenced by the broadening and the shift of the hydroxyls to lower wavenumbers (Fig. S2) [24]. For [1/Al2O3-(500)] (Table 3, entry 1), iron elemental analysis is 1.31%wt, which corresponds to 0.24 mmol Fe/g of Al2O3-(500), i.e. 0.40 Fe/AlOH. This low ratio is consistent with the presence of remaining OH group, as previously observed by IR spectroscopy. During grafting, 1.1 ± 0.1 mol of Mes∗H per mole of grafted Fe is formed. From carbon (5.06%wt) and hydrogen (0.68%wt) elemental analyses, there are 18.0 C/Fe and 29.0 H/Fe on [1/Al2O3-(500)]. These data are consistent with the formation of the surface species 8 [(AlSO){Fe(C18H29)}] (AlS = surface aluminium atom) as the major surface species (expected C/Fe and H/Fe ratio for 8: 18.0 and 29.0, respectively, Scheme 4a). For [2/Al2O3-(500)] (Table 3, entry 2), iron elemental analysis is 2.65%wt, which corresponds to 0.47 mmol Fe/g of Al2O3-(500), i.e. 0.80 Fe/AlOH. During grafting, 0.53 ± 0.1 mol of MsH per mole of grafted Fe is formed. From carbon elemental analysis (7.40%wt C), there are 13.0 C/Fe on [2/Al2O3-(500)]. These data are consistent with the formation of 9 [(AlSO){Fe2(C9H11)3}] as the major surface species (Scheme 4b; expected C/Fe ratio for 9: 13.5).
Grafting of 1 and 2 on Al2O3-(500)
Entry | Precursor | Supporta/mmol | FeR2b/mmol | %wt Fe | Fe/SiOH | RHc/mmol | RH/OHd |
1 | 1 | 0.13 | 0.25 | 1.31 | 0.40 | 0.052 | 0.40 |
2 | 2 | 0.17 | 0.20 | 2.65 | 0.80 | 0.063 | 0.37 |
a Number of mmol of OH based on 0.66 mmol of OH/g for Al2O3-(500).
b Number of mmol of Fe molecular precursor.
c Number of mmol of RH evolved during grafting.
d Ratio of alkane RH released/surface OH.
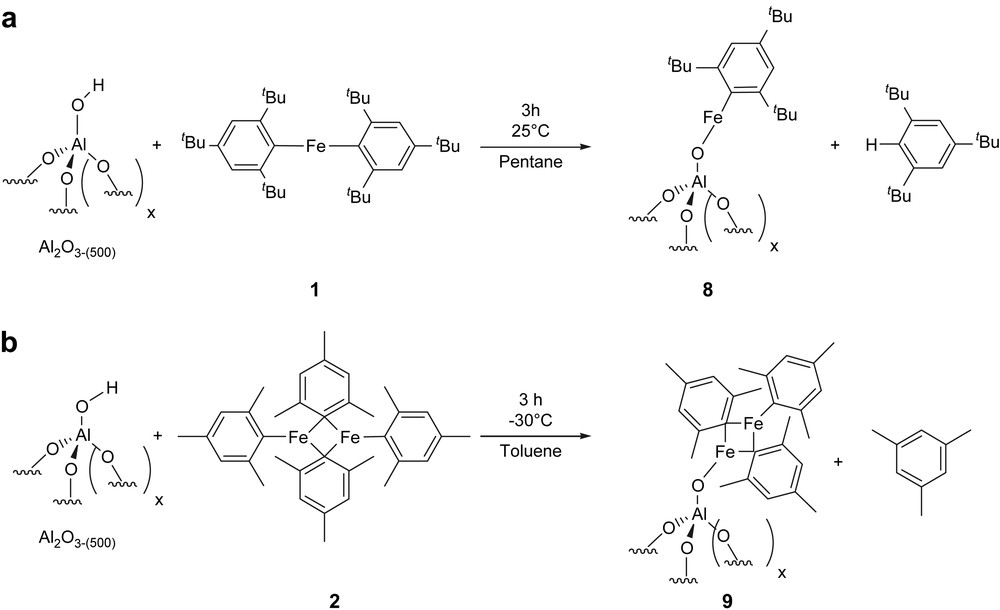
Reaction of 1 (a) and 2 (b) with the surface hydroxyls of an alumina surface (x + 2 = number of O around Al).
3 Conclusion
Independently of the oxide supports, grafting of the mononuclear perhydrocarbyl iron(II) complex generates a mononuclear complex, while the dinuclear perhydrocarbyl iron(II) complex yields a dinuclear complex as evidenced by elemental analysis and IR spectroscopy. This is in sharp contrast with what has been observed for the grafting of a molecular siloxy dinuclear Fe(II) complexes [{Fe(OSi(OtBu)3)2}2], which yields the corresponding monosiloxy mononuclear complexes [11,12]. Overall, the integrity of the structure of the molecular complexes is conserved, replacing only one alkyl group by a siloxy surface species, as already observed for other organometallic complexes [19]. Further studies are underway to obtain more structural information on these systems; this involves for instance the development of NMR spectroscopic techniques for paramagnetic materials [25].
4 Experimental procedures
4.1 General procedure
All experiments were carried out by using standard air-free methodology under argon using either glove boxes or Schlenk lines. Silica was purchased from Degussa (Aerosil, 200 m2/g). Silica–alumina was obtained from Akzo Nobel (25% alumina) and had a specific area of 478 m2/g. γ-Alumina was obtained from Degussa (162 m2/g). Toluene was distilled over Na/K amalgam and stored under argon over 3-Å molecular sieves. Ether, dioxane and THF were distilled over sodium/benzophenone mixture and stored over 3-Å molecular sieves. All solvents were degassed by four freeze–pump–thaw cycles. The complexes [Fe{C6H2(tBu)3}]2 (1) [21] and [Fe{μ1-C6H2(CH3)3}{μ2-C6H2(CH3)3}]2 (2) [22] were prepared according to the literature procedures. Gas-phase analysis was performed on a Hewlett-Packard 5890 series II gas chromatography apparatus equipped with a flame ionisation detector and an Al2O3/KCl on fused silica column (50 m × 0.32 mm). Infrared spectra were recorded on a Nicolet 550-FT by using an infrared cell equipped with CaF2 windows, allowing in situ studies. Typically 16 scans were accumulated for each spectrum (resolution, 2 cm−1). Elemental analysis was performed at the CNRS Central Analysis Service in Solaize (France) and at the Laboratory of Organometallic Synthesis and Electrosynthesis in Dijon (France).
4.2 Preparation of silica partially dehydroxylated at 700 °C, SiO2-(700)
Silica was partially treated under vacuum (1.32 × 10−3 Pa) at 500 °C for 12 h and at 700 °C for 4 h in order to obtain SiO2-(700). After this treatment, the specific area was about 200 m2/g with an OH density of 0.7 OH/nm2 (0.26 mmol OH/g).
4.3 Reaction of 1 with SiO2-(700) (monitoring by IR spectroscopy): representative procedure
The grafting of 1 on a silica pellet was performed using the following experimental procedure. In an IR-cell, 50 mg of silica were pressed into a 18-mm self-supporting disk, put into a sealed glass high-vacuum reactor equipped with CaF2 windows, and partially dehydroxylated under vacuum at 700 °C. The silica disk was then immersed in a pentane solution of 1 (1.67 mg/mL) at 25 °C for 3 h followed by three pentane washings and a drying step under dynamic vacuum at room temperature. An IR spectrum was recorded after each step.
4.4 Reaction of 1 with SiO2-(700) by impregnation in pentane [1/SiO2-(700)]: representative procedure
A mixture of SiO2-(700) (750 mg, 0.195 mmol OH) and 1 (110 mg, 0.21 mmol) in pentane (10 mL) was stirred at 25 °C for 3 h. After filtration, the solid was washed three times with pentane, and the solvent was analysed in order to quantify 2,4,6-tritertiobutylbenzene evolved during grafting. The resulting yellow powder was dried under vacuum (1.32 × 10−3 Pa, 2 h) to yield 650 mg of solid 3. Analysis by gas chromatography indicated the formation of 0.19 mmol of 2,4,6-tritertiobutylbenzene during the grafting. Elemental analysis of 3: Fe 1.23%wt, C 3.93%wt, H 0.56%wt.
4.5 Reaction of 2 with SiO2-(700) (monitoring by IR spectroscopy): representative procedure
The grafting of 2 on a silica pellet was performed using the following experimental procedure. In an IR cell, 50 mg of silica were pressed into a 18-mm self-supporting disk, put into a sealed glass high-vacuum reactor equipped with CaF2 windows, and partially dehydroxylated under vacuum at 700 °C. The silica disk was then immersed in a toluene solution of 2 (1.67 mg/mL) at −30 °C for 24 h followed by three toluene washings and a drying step under dynamic vacuum at room temperature. An IR spectrum was recorded after each step.
4.6 Reaction of 2 with SiO2-(700) by impregnation in toluene [2/SiO2-(700)]: representative procedure
Silica (400 mg, 0.105 mmol OH) was treated at 25 °C with a solution of 2 (155 mg, 0.26 mmol) in toluene (8 mL) under an inert atmosphere. The reaction mixture was cooled at −30 °C, stirred at this temperature for 3 h and then kept for 24 h at −30 °C without stirring. After filtration, the solid was washed three times with toluene, and the solvent was condensed into another reactor in order to quantify mesitylene evolved during grafting. The resulting red powder was dried under vacuum (1.32 × 10−3 Pa). Analysis by gas chromatography indicated the formation of 0.096 mmol of mesitylene during the grafting. Elemental analysis of 4: Fe 2.33%wt, C 5.5%wt, H 0.51%wt.
4.7 Preparation of silica alumina
Silica–alumina was calcined at 500 °C under N2/O2 flow for 24 h and heated at 500 °C under high vacuum (1.32 × 10−3 Pa) for 24 h to give a white solid having a specific area of 390 m2/g and containing 1.4 OH/nm2 (0.88 mmol OH/g).
4.8 Reaction of 1 with SiO2–Al2O3-(500) (monitoring by IR spectroscopy)
This was performed as described above for the grafting of 1 on silica, but calcination at 500 °C under N2/O2 flow overnight was carried out before a treatment under vacuum (500 °C, 12 h).
4.9 Reaction of 1 with SiO2–Al2O3-(500) by impregnation in pentane [1/SiO2–Al2O3-(500)]: representative procedure
A mixture of SiO2–Al2O3-(500) (250 mg, 0. 22 mmol OH) and 1 (144 mg, 0.26 mmol) in pentane (10 mL) was stirred at 25 °C for 3 h. After filtration, the solid was washed three times with pentane, and the solvent was analysed in order to quantify 2,4,6-tritertiobutylbenzene evolved during grafting. The resulting yellow powder was dried under vacuum (1.32 × 10−3 Pa, 1 h, 25 °C) to yield 300 mg of solid 6. Analysis by gas chromatography indicated the formation of 0.14 mmol of 2,4,6-tritertiobutylbenzene during the grafting. Elemental analysis of 6: Fe 3.21%wt, C 13.50%wt, H 1.95%wt.
4.10 Reaction of 2 with SiO2-Al2O3-(500) (monitoring by IR spectroscopy)
This was performed as described above for the grafting of 1 on silica–alumina.
4.11 Reaction of 2 with SiO2–Al2O3-(500) by impregnation in toluene [2/SiO2–Al2O3-(500)]: representative procedure
A mixture of SiO2–Al2O3-(500) (250 mg, 0.22 mmol OH) and 2 (155 mg, 0.26 mmol) in toluene (10 mL) was stirred at −30 °C for 24 h. After filtration, the solid was washed three times with toluene, and the solvent was analysed in order to quantify mesitylene evolved during grafting. The resulting red powder was dried under vacuum (1.32 × 10−3 Pa, 1 h, 25 °C) to yield 300 mg of solid 7. Analysis by gas chromatography indicated the formation of 0.13 mmol of mesitylene during grafting. Elemental analysis of 7: Fe 5.29%wt, C 16.2%wt.
4.12 Preparation of alumina
γ-Alumina was calcined at 500 °C under N2/O2 flow overnight and then heated at 500 °C under high vacuum (10−5 Torr) for 12 h to give a white solid having specific area of 100 m2/g and containing 4.0 OH/nm2 (0.66 mmol OH/g). 27Al CP/MAS solid state NMR: δ = 4 and 62 ppm, in agreement with 3 to 1 ratio of octahedral and tetrahedral aluminium sites. Powder X-ray diffraction is in agreement with a γ-alumina phase.
4.13 Reaction of 1 with Al2O3-(500) (monitoring by IR spectroscopy)
This was performed as described above for the grafting of 1 on silica–alumina, but calcination at 500 °C under N2/O2 flow overnight was carried out before a treatment under vacuum (500 °C, 12 h).
4.14 Reaction of 1 with Al2O3-(500) by impregnation in pentane [1/Al2O3-(500)]: representative procedure
A mixture of Al2O3-(500) (200 mg, 0.13 mmol) and 1 (135 mg, 0.248 mmol) in pentane (10 mL) was stirred at 25 °C for 3 h. After filtration, the solid was washed three times with pentane, and the solvent was analysed in order to quantify 2,4,6-tritertiobutylbenzene evolved during grafting. The resulting yellow powder was dried under vacuum (1.32 × 10−3 Pa, 1 h, 25 °C) to yield 300 mg of solid 8. Analysis by gas chromatography indicated the formation of 0.052 mmol of 2,4,6-tritertiobutylbenzene during the grafting. Elemental analysis of 8: Fe 1.31%wt, C 5.06%wt, H 0.68%wt.
4.15 Reaction of 2 with Al2O3-(500) (monitoring by IR spectroscopy)
This was performed as described above for the grafting of 2 on silica–alumina.
4.16 Reaction of 2 with Al2O3-(500) by impregnation in toluene [2/Al2O3-(500)]: representative procedure
A mixture of Al2O3-(500) (250 mg, 0.17 mmol OH) and 2 (116 mg, 0.197 mmol) and in toluene (10 mL) was stirred at −30 °C for 24 h. After filtration, the solid was washed three times with toluene, and the solvent was analysed in order to quantify mesitylene evolved during grafting. The resulting red powder was dried under vacuum (1.32 × 10−3 Pa, 1 h, 25 °C) to yield 310 mg of solid 9. Analysis by gas chromatography indicated the formation of 0.063 mmol of mesitylene during grafting. Elemental analysis of 9: Fe 2.65%wt, C 7.4%wt.
Acknowledgement
We are all indebted to the CNRS and ESCPE Lyon for financial supports.