1 Introduction
The design of molecules which could be utilised for information processing and data storage is one of the main challenges in present material science. Molecules suitable for information technology applications should ideally exhibit bistability, which may be defined as a property of a molecular system that allows it to exist in two different electronic states over a certain range of external perturbation. Among potentially suited classes of molecules, spin transition (ST) iron(II) complexes have been studied extensively, in particular because of their well-differentiable magnetic signal discriminating the diamagnetic low-spin (LS; S = 0) and the paramagnetic high-spin (HS; S = 2) states. The ST event can be driven by external triggers such as temperature, pressure or light irradiation [1]; external parameters which are of interest for applications. Thus, iron(II) ST compounds possessing high transition temperatures (T1/2), ideally around room temperature, have drawn much attention due to their potential as active components in molecular devices [2,3]. The potential of ST systems for data storage has been emphasised by several reports [2]. For successful implementation of ST compounds into information processing devices, a thermally induced spin state transition should occur (i) at or around room temperature, (ii) with a high degree of abruptness and (iii) with a large magnetic hysteresis loop [2]. Recently, it has been shown how targeted substitution in the 4-position of the bispyrazolylpyridine motif can steer the spin transition of the respective Fe(II) complexes to regions above room temperature exhibiting a good degree of steepness [4] and acceptable widths of the magnetic hysteresis loops [5].
In continuation of this work and in view of the continuing interest in room temperature ST in iron(II) compounds [4–7], we report herein on the synthesis, structural and magnetic characterisation of a series of four iron(II) bispyrazolylpyridine compounds of the general formula [FeII(L1–4)2](ClO4)2·nCH3CN (1–4) with L being 4-iodo-2,6-bis(pyrazol-1-yl)pyridine (L1), 4-(4′-hydroxyphenyl)-2,6-bis(pyrazol-1-yl)-pyridine (L2), 4-(4′-trimethylsilylethynyl)-2,6-bis(pyrazol-1-yl)pyridine (L3) and 4-ethynyl-2,6-bis(pyrazol-1-yl)pyridine (L4); n = 0 for 1, 2, and 4; n = 2 for 3.
2 Experimental
2.1 General
All chemicals were purchased and used as received. The solvents diisopropyl ether, acetonitrile, hexane, ethyl acetate were used without any further purification. 1H and 13C NMR spectra were recorded in a Bruker DPX 300 spectrometer with solvent protons as an internal standard. Elemental analyses were carried out on a Vario MICRO cube. MALDI-TOF MS data were acquired on a Voyager-DE PRO Bio spectrometry workstation.
2.2 Magnetic susceptibility measurement
Temperature dependent static susceptibilities of the complexes (15–20 mg) were recorded for cooling and heating cycles (2 K min−1) using a MPMS-5S (Quantum Design) SQUID magnetometer over a temperature range 4.5–380 K in a homogeneous 0.1 Tesla external magnetic field. Gelatine capsules were used as sample containers for measurements. The very small diamagnetic contribution of the gelatine capsule had a negligible contribution to the overall magnetisation, which was dominated by the sample. The diamagnetic corrections of the molar magnetic susceptibilities were applied using Pascal's constants.
2.3 Single crystal diffraction
Single crystal X-ray diffraction data were collected on a STOE IPDS II diffractometer with graphite monochromated Mo Kα radiation (0.71073 Å). The structures were solved by direct methods (SHELX-97). Refinement was performed with anisotropic temperature factors for all non-hydrogen atoms (disordered atoms were refined isotropically) [12,13].
2.4 Synthesis of the ligands
The ligands 4-iodo-2,6-bis(pyrazol-1-yl)pyridine (L1), 4-(4′-trimethylsilylethynyl)-2,6-bis(pyrazol-1-yl)pyridine (L3) and 4-ethynyl-2,6-bis(pyrazol-1-yl)pyridine (L4) were synthesised following reported protocols [4,6].
2.4.1 Synthesis of 4-(4′-hydroxyphenyl)-2,6-bis(pyrazol-1-yl)-pyridine (L2)
4-Iodo-2,6-bis(pyrazol-1-yl)pyridine (L1) (0.67 g, 2 mmol) and 4-hydroxy phenyl boronic acid (0.29 g, 2.1 mmol) and Pd(0)(PPh3)4 (10 mol.%) were suspended in N2 bubbled solvents of methanol and toluene (1:1, 100 ml) and 2 M Na2CO3 (8 ml). The mixture was heated to 70 °C for 2 days under nitrogen atmosphere. The crude reaction mixture was poured into ice water (500 ml) and extracted with dichloromethane. The separated organic layer was dried over MgSO4 and the solvent removed. The solid residue was purified by column chromatography on silica gel with hexane/ethyl acetate (10:1) as the eluent obtaining the ligand (L2) as a white powder 0.41 g (68% yield). 1H NMR (DMSO-d6; δ): 9.27 (s, 1H, OH); 8.34 (d, 2H, Pz-H5, J = 4 Hz); 7.71 (s, 2H, Py); 7.46 (m, 2H, Pz-H4); 7.38 (d, 2H, Har, J = 9 Hz); 6.64 (d, 2H, Har, J = 9 Hz); 6.22 (d, 2H, –H3, J = 5 Hz). 13C NMR (DMSO-d6; δ): 159.25; 153.51; 142.34; 128.31; 127.49; 126.94; 116.48; 108.14; 106.09. MALDI-TOF (matrix 2,5-dihydroxybenzoic-acid) m/z: found 303.80 (M+, 100%), calcd. for C17H13N5O (M+) 303.11. Elemental analysis: calcd. for C17H13N5O: C 67.32%; H 4.32%; N23.09%, found: C 67.39%; H4.53%; N23.00%.
2.5 Synthesis of the compounds 1–4
All the compounds 1–4 were prepared by using the following general method. In a 100 ml Schlenk tube, a solution of the ligand L (0.33 mmol) and Fe(ClO4)2·6H2O (0.165 mmol) in acetonitrile (60 ml) was heated at 80 °C for about 5 h under N2. The reaction mixture was cooled and filtered. The single crystals were grown by diffusing diisopropyl ether to the above solution under N2 at room temperature in several days.
Caution. Although we have experienced no difficulties in handling these complexes, metal-organic perchlorates are potentially explosive and should be handled with care in small quantities.
2.5.1 Compound 1
Calcd. for C22H16Cl2FeI2N10O8: C 28.44%; H 1.74%; N 15.08%. Found: C 28.41%; H 1.82; N 15.02%. Yield = 79%, formula weight = 929.00, MALDI-TOF (matrix glycerol anhydrous) m/z: found 727.90 ([FeII(L1)2]2+, 20%), calculated for C22H16FeI2N10, 730.1, crystal colour: brown, T/K = 180, crystal system: orthorhombic, space group: Pbcn, a/Å = 16.988(3), b/Å = 11.010(2), c/Å = 15.999(3), V/Å3 = 2992.5(10), Z; ρcalc/g cm−3 = 4; 2.062, μ(Mo Kα)/mm−1 = 2.810, F(000) = 1792, crystal size/mm = 0.34 × 0.33 × 0.30, θ range for the data collection/° = 2.20–25.68, final R indices[I > 2σ(I)]: R1 = 0.0273; wR2 = 0.0669, R indices (all data): R1 = 0.0287; wR2 = 0.0678, extinction coefficient = 0.0053(3), GOF on F2 = 1.139, CCDC reference number: 682057.
2.5.2 Compound 2
Calcd. for C34H26Cl2FeN10O10: C 47.41%; H 3.04%; N 16.26%. Found: C 47.14%; H 3.27%; N 16.05. Yield = 85%, formula weight = 861.40, MALDI-TOF (matrix glycerol anhydrous) m/z: found 660.61 ([FeII(L2)2]2+, 90%), calculated for C34H26 FeN10O2, 662.5, crystal colour: brown, T/K = 180, crystal system: orthorhombic, space group: Pcca, a/Å = 22.345(5), b/Å = 10.753(2), c/Å = 14.579(3), V/Å3 = 3503.1(12), Z; ρcalc/g cm−3 = 4; 1.633, μ(Mo Kα)/mm−1 = 0.659, F(000) = 1760, crystal size/mm = 0.37 × 0.36 × 0.24, θ range for the data collection/° = 2.52–25.65, final R indices[I > 2σ(I)]: R1 = 0.0456; wR2 = 0.1136, R indices (all data): R1 = 0.0475; wR2 = 0.1149, extinction coefficient = 0.0106(16), GOF on F2 = 1.105; the perchlorate anions are disordered, CCDC reference number: 682058.
2.5.3 Compound 3
Calcd. for C36H40Cl2FeN12O8Si2: C 45.92%; H 4.58%; N 17.37%. Found: C 45.24%; H 4.27%; N 17.31%. Yield = 75%, formula weight = 951.73, crystal colour: dark orange, T/K = 180, crystal system: monoclinic, space group: C2/c, a/Å = 23.575(5), b/Å = 12.106(2), c/Å = 17.743(4), β/° = 115.68(39), V/Å3 = 4563.8(16), Z; ρcalc/g cm−3 = 4; 1.385, μ(Mo Kα)/mm−1 = 0.561, F(000) = 1968, crystal size/mm = 0.40 × 0.38 × 0.35, θ range for the data collection/° = 2.54–25.63, final R indices[I > 2σ(I)]: R1 = 0.0629; wR2 = 0.1553, R indices (all data): R1 = 0.0680; wR2 = 0.1591, extinction coefficient = 0.0036(12), GOF on F2 = 1.043, the perchlorate anions and the acetonitrile molecules are disordered, CCDC reference number: 682059.
T/K = 298, crystal colour: light orange, λ/Å = 0.71073, crystal system: monoclinic, space group: C2/c, a/Å = 23.629(5), b/Å = 12.322(3), c/Å = 17.845(4), β/° = 115.15(3), V/Å3 = 4703.3(16), Z; ρcalc/g cm−3 = 4; 1.344, μ(Mo Kα)/mm−1 = 0.544, F(000) = 1968, crystal size/mm = 0.38 × 0.12 × 0.10, θ range for the data collection/° = 1.90–25.63, final R indices[I > 2σ(I)]: R1 = 0.0558; wR2 = 0.1660, R indices (all data): R1 = 0.0656; wR2 = 0.1733, GOF on F2 = 1.042.
2.5.4 Compound 4
Calcd. for C26H18Cl2FeN10O8: C 43.06%; H 2.05%; N 19.31%. Found: C 43.19%; H 2.5%; N 19.24%. Yield = 80%, formula weight = 725.23, MALDI-TOF (matrix glycerol anhydrous) m/z: found 623.82 ([FeII(L1)2]+(ClO4), 15%), calculated for C26H18ClFeN10O4, 625.78, crystal colour: red, T/K = 180, crystal system: tetragonal, space group: I41/a, a/Å = b/Å = 9.6248(14), c/Å = 29.593(6), V/Å3 = 2741.4(8), Z; ρcalc/g cm−3 = 4; 1.757, μ(Mo Kα)/mm−1 = 0.819, F(000) = 1472, crystal size/mm = 0.25 × 0.22 × 0.20, θ range for the data collection/° = 2.23–25.63, final R indices[I > 2σ(I)]: R1 = 0.0558; wR2 = 0.1660, R indices (all data): R1 = 0.0558; wR2 = 0.1660, extinction coefficient = 0.0026(6), GOF on F2 = 1.120, CCDC reference number 682060.
3 Results
3.1 Synthesis
The ligands L1, L3 and L4 were synthesised following literature procedures [4,6]. Ligand L2, 4-(4′-hydroxyphenyl)-2,6-bis(pyrazol-1-yl)-pyridine was obtained as a white powder in 68% yield via a Pd-catalyzed Suzuki cross-coupling reaction from ligand L1, 4-iodo-2,6-bis(pyrazol-1-yl)pyridine, with 4-hydroxy phenyl boronic acid (Scheme 1).

Reagents and conditions: (i) Pd(0)(PPh3)4, 2 M Na2CO3, toluene, MeOH; (ii) Pd(0)(PPh3)4, CuI, Et2NH; (iii) K2CO3, MeOH; (iv) Fe(ClO4)2·xH2O, CH3CN.
The compounds (1–4) were prepared by the reaction of two equivalents of the respective ligand L with one equivalent of iron(II) perchlorate in acetonitrile at 80 °C under inert atmosphere of N2. Single crystals suitable for X-ray diffractions were grown by diffusing diisopropyl ether into the acetonitrile solution of the complexes under N2 at room temperature for several days. Compounds 1 and 2 crystallise as brown blocks while the colour of compound 3 is light orange. The compound 4 crystallises as dark red blocks. All the crystals were found to be stable at room temperature without decomposition and loss of solvent molecules.
3.2 Solid state structures of the compounds 1–4
Selected Fe–N bond lengths of the complex dications in (1–4) at different temperatures are given in Table 1. The X-ray diffraction studies of single crystals of [FeII(L1)2](ClO4)2 (1) reveal the orthorhombic space group Pbcn exhibiting a unit cell volume of 2992.5(10) Å3 (a = 16.988(3) Å, b = 11.010(2) Å and c = 15.999(3) Å). Four formula units are included in the unit cell and no solvent molecules are present in the crystal lattice. Fig. 1a shows the molecular structure of the dication [FeII(L1)2]2+ in 1. The coordination environment in the iron(II) metal ion can be described as a distorted octahedron. The complex exhibits at 180 K Fe(II)–N bond lengths varying from 1.899(2) to 1.968(2) Å (Table 1).
Selected bond distances and angles of the coordination polyhedra of the complex dications in 1–4
1 | 2 | 3 | 3 | 4 | |
180 K | 180 K | 180 K | 298 K | 180 K | |
Fe1–N1 | 1.968(2) | 1.964(2) | 2.106(3) | 2.176(3) | 1.972(15) |
Fe1–N3 | 1.899(2) | 1.900(2) | 2.054(3) | 2.123(2) | 1.881(2) |
Fe1–N5 | 1.962(2) | 1.976(2) | 2.104(3) | 2.176(3) | – |
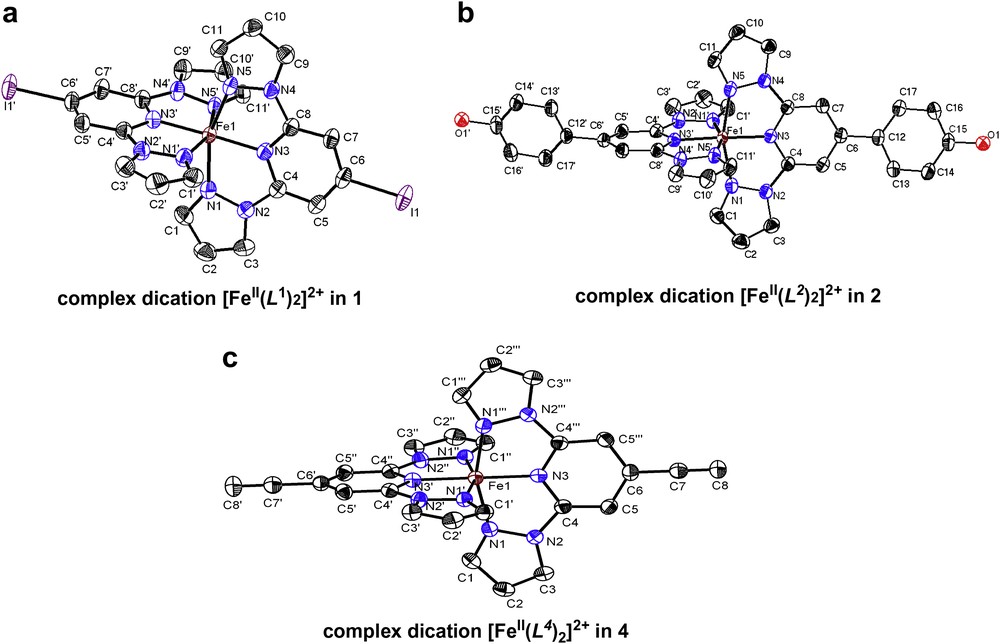
(a–c) Representation of the ORTEP views of the dications [FeII(Lx)2]2+ in 1, 2 and 4. The thermal ellipsoids represent the 50% probability level. All molecular structures were determined at 180 K. Anions and hydrogen atoms were omitted by clarity.
X-ray diffraction studies on single crystals of [FeII(L2)2](ClO4)2 (2) at 180 K show the orthorhombic group Pcca symmetry exhibiting a unit cell volume of 3503.1(12) Å3 (a = 22.345(5) Å, b = 10.753(2) Å and c = 14.579(3) Å). Four formula units can be found within the unit cell. The molecular structure of the dication [FeII(L2)2]2+ is shown in Fig. 1b. The hydroxyl group is protonated, but the respective OH groups do not form any intermolecular contact with neighboring hydrogen bond acceptors or donors. The coordination environment in the iron(II) metal ion can be described as a tetragonally distorted octahedron. The complex cation exhibits Fe(II)–N bond lengths varying from 1.900(2) to 1.976(2) Å at 180 K (Table 1).
For [FeII(L3)2](ClO4)2·2CH3CN (3), X-ray diffraction data were collected at both 298 K as well as 180 K using two different crystals. In both cases, single crystal X-ray structure analysis reveals the monoclinic space group C2/c. At 180 K compound 3 contains two non-bonded CH3CN solvent molecules per complex molecule, which were also detected in the crystal lattice at 293 K. The cell volume and unit cell dimensions show slight variations at the two different temperatures. At 180 K, the cell volume is 4563.8(16) Å3 and increases to 4703.3(16) Å3 at 298 K. The corresponding unit cell parameters are a = 23.575(5) Å, b = 12.106(2) Å, and c = 17.743(4) Å at 180 K and a = 23.629(5) Å, b = 12.322(3) Å, and c = 17.845(4) Å at 298 K. Fig. 2a depicts the molecular structure of the dication [Fe(L3)2]2+ in 3. At 180 K, the Fe(II)–N bond lengths range from 2.054(3) to 2.106(3) Å, while at room temperature the Fe(II)–N bond lengths range from 2.123(2) to 2.176(3) Å (Table 1). At both temperatures, the coordination environment in the iron(II) metal ion can be described as a distorted octahedral. Moreover, the temperature dependence of the colour change of one crystal of complex 3 was investigated by decreasing the temperature slowly from 298 to 180 K, the initially light orange colour of a crystal of complex 3 changes successively to dark orange without loosing crystallinity (Fig. 2b).
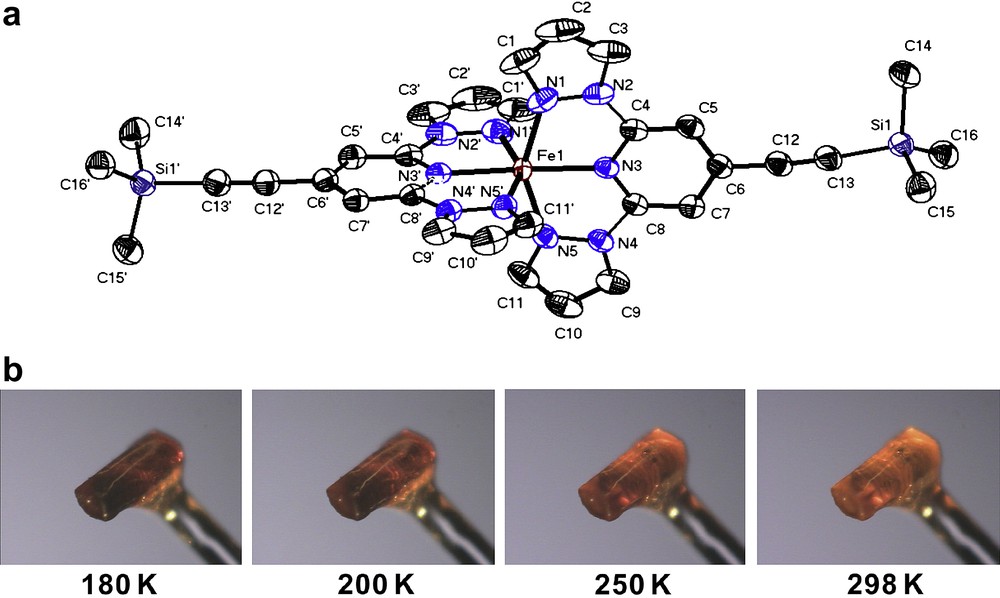
(a) ORTEP plot of the complex dication [FeII(L3)2]2+ in 3·2CH3CN at 180 K (50% probability ellipsoids). The molecular structure was determined at 180 K. Anions, solvent molecules and hydrogen atoms were omitted for clarity. (b) Photographs of a single crystal of complex 3·2CH3CN showing its thermochromism at four different temperatures.
The single crystals of [FeII(L4)2](ClO4)2 (4) are red and the X-ray diffraction studies reveal the tetragonal space group I41/a at 180 K. The compound shows a unit cell volume of 2741.4(8) Å3 (a = b = 9.6248(14) Å, and c = 29.593(6) Å) including four formula units per unit cell. No solvent molecules were detected in the crystal lattice. Fig. 1c shows the molecular structure of the complex dication [FeII(L4)2]2+ in 4. The coordination environment in the iron(II) metal ion can be described as a distorted octahedron. The complex exhibits Fe(II)–N bond lengths varying from 1.881(2) to 1.972(15) Å at 180 K (Table 1).
3.3 Temperature dependent magnetic properties
The temperature dependent magnetic susceptibilities of the compounds 1–4 were performed for both heating (↑) and cooling cycles (↓) in the temperature range 4.5–380 K. Field dependent magnetisation measurement was also carried out for complex 3.
The product of the molar magnetic susceptibility and temperature (χT) of the complex [FeII(L1)2](ClO4)2 (1) is 3.49 emu K mol−1 at 380 K, which is slightly higher than the value expected for a HS state (S = 2) of an iron(II) ion (Fig. 3a). On cooling, (χT) starts to decrease very abruptly at 340 K and reaches a plateau at 260 K approaching a low temperature value of 0.045 emu K mol−1. This value can be attributed to the iron(II) LS (S = 0) state. Measurements were performed in both heating (↑) and cooling (↓) cycles. It is interesting to note that the compound 1 is one rare example of Fe(II) complexes exhibiting spin transition above room temperature with a T1/2 of 333 K [5a].

The solid state χT vs. T plots of compound 1 (left) and compound 2 (right) exhibiting T1/2 value above room temperature.
The temperature dependent magnetic measurement of complex [FeII(L2)2](ClO4)2 (2) is shown in Fig. 3b. At 380 K, the value of χT is 3.01 emu K mol−1, which is in accordance with the value for a high-spin (S = 2) state iron(II) metal ion. On cooling, χT starts to decrease at 360 K relatively smoothly before reaching at 200 K a plateau close to zero a value which corresponds to the LS state (S = 0) of an iron(II) metal ion. Meanwhile, the measurements performed in both heating (↑) and cooling (↓) cycles have revealed that there is no indication of hysteresis as a result of intermolecular cooperativity in the solid state. In this case also, it is interesting to note that the ST temperature is close to room temperature [8] with a T1/2 = 281 K.
For the complex [FeII(L3)2](ClO4)2·2CH3CN (3), the product of the molar magnetic susceptibility and temperature (χT) is 3.03 emu K mol−1 at 380 K (Fig. 4a), which is in accordance to the value expected for a HS state (S = 2) of an iron(II) ion. On cooling the χT value remains constant at this value over the temperature range from 380 to 300 K. Spin transition occurs between 300 and 125 K reaching a second plateau of 1.76 emu K mol−1 around 140 K. This value coincides with a situation where half of the iron(II) centres in the crystal lattice have undergone spin transition, while the other half remain in the paramagnetic HS state. Field dependent magnetisation measurements at 4.2 K exhibit magnetic saturation at around 2 μB mol−1 (Fig. 4b). The χT in temperature range 120–5 K reveals a strong increase, in particular below 50 K. Below 10 K, χT versus T plot drops strongly due to the zero field splitting of Fe(II) HS metal ions. Measurements were performed in both heating (↑) and cooling (↓) cycles and have revealed that there is no evidence for thermal hysteresis. It is also interesting to note that the compound does not seem to loose any solvent molecules in the lattice even at 380 K unlike other compounds of the iron(II) bispyrazolylpyridine family [5b].
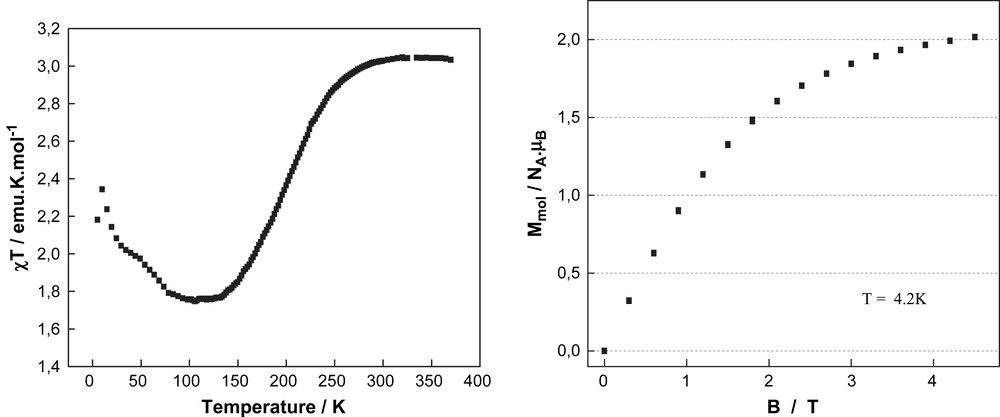
(a) The solid state χT vs. T plot and (b) field vs. magnetisation plot (right) of compound 3.
In the case of complex [FeII(L4)2](ClO4)2 (4), variable temperature magnetic susceptibility measurements show that the complex remains completely low spin in the whole temperature range from 5 to 380 K. This is surprising, since the structurally very close compounds 3 and 4 are only distinguished by the replacement of the trimethylsilyl group by the less bulky proton.
4 Discussion
The single crystal diffraction and magnetic studies of compounds 1 and 2 reveal for both compounds very similar structural and magnetic behaviour. At 180 K, the averaged Fe(II)–N bond lengths are close to 1.90 Å for the central pyridine rings and between 1.96 and 1.98 Å for the peripheral pyrazolyl rings (Table 1). Such bond length values are typical for iron(II) metal ions in the LS state, [1b] which is also confirmed by the magnetic investigations with χT values close to 0 emu K mol−1 below 180 K. Both complexes exhibit drastic changes of χT with increasing temperatures: While compound 2 reveals a smooth, almost gradual transition between 200 and 380 K, compound 1 exhibits a relatively steep increase of χT between 330 and 370 K. At 380 K, both complexes reach magnetic saturation of the χT at values typical for the HS state with S = 2. Noticeably, both compounds show spin transition above room temperature, a region extremely interesting for applications.
On comparing the electronic effects of the substituents in the 4-position of the bispyrazolylpyridine moiety, the iodo group of compound 1 exercises a slightly negative, while the hydroxyl phenyl group a slightly positive inductive effect. Interestingly, the analogous, in the 4-position unsubstituted bispyrazolylpyridine iron(II) perchlorate compound remains fully high-spin between 300 and 5 K [10]. Although comparability is given by the same type of counteranions and the absence of lattice solvent molecules, the scattering of these results is not fully explicable on the only base of ligand field strength discussions. It has been previously shown for the class of iron(II) bispyrazolylpyridine compounds that slight packing effects in the crystal lattice, varying positions and even different disordered schemes of anions are strong enough to alter considerably the ST behaviour [5b]. In accordance with the absence of intermolecular contacts in the X-ray diffraction studies, no hysteresis was observed in the solid state magnetic studies of 1 and 2. The importance of such contacts for the evolution of intermolecular cooperativity, expressed by the appearance of hysteresis loops with up to 8 K width, has been shown previously for further members of the bispyrazolylpyridine iron(II) compound family [4,5a].
The spin transition behaviour of compound 3 turned out to be more complex. The presence of a spin transition above 180 K was evidenced by the distinct thermochromism going from dark to pale orange on heating to 298 K (Fig. 3b). At 298 and 180 K, the X-ray single crystal structure of compound 3 shows the inclusion of two acetonitrile solvent molecules into the crystal lattice. The averaged Fe–N bond distances correspond with 2.12 Å for the central pyridine and 2.18 Å for the pyrazol groups of the ligand L3 indicate HS state character of the coordinated iron(II) metal ion at 298 K. This result is confirmed by the magnetic studies showing a χT value close to 3 emu K mol−1, which is in accordance to the value expected for an all HS situation at 298 K and higher temperatures (Fig. 4a). However, the χT value starts to decrease drastically at temperatures below 260 K in order to reach a plateau between 140 and 125 K with a χT value of 1.76 emu K mol−1. This value can only be interpreted by a magnetic situation where only half of the involved iron(II) metal ions have undergone transition into the LS (S = 0) state, while the remaining iron(II) centres are supposed to be kept in the HS state (S = 2). Such 50%/50% HS/LS situation at low temperatures was further confirmed by field dependent magnetisation measurements at 4.2 K where saturation shows up at around 2 μB mol−1; half of the value expected for four electrons per molecule (Fig. 4b). Accordingly, the X-ray diffraction studies at 180 K reveal in terms of Fe(II)–N bond lengths a situation (d(Fe–Npyridine) = 2.05 Å; d(Fe–Npyrazol) = 2.11 Å), which can be also attributed to an admixture of LS and HS bond lengths. Below 125 K the χT value shows first a slight increase, and then a strongly pronounced increase in the temperature range 50-5K, which can be attributed to a successive inset of weak ferromagnetic coupling of the remaining iron(II) HS centres. Cohabitation of spin transition and ferromagnetic coupling is rather unusual, but was recently reported [9]. Below 10 K, χT drops strongly due to the zero field splitting of Fe(II) HS metal ions. Magnetic measurements performed in both heating (↑) and cooling (↓) cycles revealed absence of magnetic hysteresis so demonstrating absence of intermolecular cooperativity in the solid state.
Compound 4 does not show any evidence for spin transition and the iron(II) centres and remains completely diamagnetic in the temperature range of 5–380 K. In accordance, the crystal structure at 180 K exhibits Fe–N bond distances typical for the iron(II) LS state (Fig. 1, Table 1). In comparison with compound 3, not only the steric bulk of the trimethylsilyl group but also the inclusion of solvent molecules leads obviously to this drastically changed ST behaviour; proving high sensitivity of the spin transition to subtle changes in the molecular environment.
5 Conclusions
We have characterised structurally and magnetically four iron(II) bispyrazolylpyridine compounds bearing different substituents in the 4-position. Variable temperature magnetic studies reveal that the substituents in the 4-position of the bispyrazolylpyridine ligand have a pronounced effect on the ST properties of the complexes. In view of applications, the access to spin transition iron(II) complexes showing transition temperatures close to ambient temperature is of pivotal importance for their use as active units in device architectures [11]. Out of the four studied compounds herein, two show spin transition above or close to room temperature, which shows how controlled molecular design can achieve attractive working parameters. The still missing hysteretic behaviour can be reintroduced by increasing the cooperativity between the ST centres, e.g. by incorporating additional interlinking paramagnetic centres using polydentate ligands or by favoring weak hydrogen or π–π bonds in the crystal lattices. The construction of such multinuclear ST architectures is under progress [4,5].
Acknowledgements
The authors thank the Deutsche Forschungsgemeinschaft (DFG) for financial support under CFN (Center for Functional Nanostructures – Project E3). We are also thankful to Prof. Horst Hahn for providing the necessary instrumental facilities.