1 Introduction
Virgin olive oils play an important role in the food industry and are important in human nutrition for a variety of reasons. Lipids are the main sources of energy for the human body compared with their weight. Moreover, olive oils are becoming increasingly important as suppliers of fat-soluble vitamins and polyphenols as antioxidants. They are also an important source of essential unsaturated fatty acids, which cannot be synthesized by the human body. Virgin olive oils are extracted from olive fruits by using only physical methods, which include crushing of olives, malaxation of resulting pastes and separation of the oily phase. Because of its location in mesocarp of cells and the use of purely mechanical pieces of apparatus for its extraction, virgin olive oil does not require further treatment before its consumption. Therefore, it preserves a great number of volatile compounds, responsible for the aroma and also the presence of antioxidant compounds which inhibit the lipid autoxidation (nonenzymatic oxidation by dioxygen).
Provence, the most important area of France for olive oil production, is part of the Mediterranean basin where the climate and soil encourage production of olive fruits and consequently virgin olive oil at high quality with lots of volatile and non-volatile compounds of interest. The extraction procedure is of great importance to produce olive oil rich in secondary metabolites [1–4]. The choice of the technique is the result of a compromise between efficiency and reproducibility of extraction, ease of procedure, together with considerations of cost, time, degree of automation and safety.
The objective of this work was to investigate the effects of the crushing systems, stone mill and hammer crusher, on the chemical changes of virgin olive oil considering quality parameters such as: free acidity, peroxide value, conjugated dienes concentration, fatty acid composition, volatile compounds, and polyphenolic compounds with regard to their antioxidant potentialities. The chemical analysis studies were supplemented by scanning electron micrographs to shed light on the physical action of the two crushing systems.
2 Materials and methods
2.1 Sample collection and reagents
In these investigations, oil was extracted from olives Aglandau (Vaucluse, France) collected from local fields (Domus Claudia, Hameau de st Veran, France) during the harvesting period for oil production (1st and 2nd of December 2007). 1000 kg of olives were harvested and processed in a small scale mill (Le petit Moulin, Travaillan, France) on the 3rd of December 2007.
Acetic acid, chloroform, sodium thiosulfate, ethanol 96%, phenolphthalein, BF3–methanol and n-heptane used were of analytical grade; n-hexane and Folin-Ciocalteu reagent were of UV-grade; and methanol, water and n-hexane used for HPLC were of HPLC grade. All these solvents were supplied by VWR International (Darmstadt, Germany).
Standards of gallic acid, vanillic acid, p-coumaric acid, ferulic acid and trans-cinnamic acid were from Alfa-Aesar (Karlsruhe, Germany) and those of tyrosol, luteolin and apigenin were from Extrasynthèse (Genay, France).
2.2 Oil processing
2.2.1 The stone mill
The stone mill is still the most common way of crushing olives in the south of France even if only small scale mills can use it due to the longer time of grinding compared to more modern crushers (Fig. 1). In the mill of this study the two granite stones used to grind the olives were 23 cm wide and turned at 17 rpm. The maximum charge at one time was 250 kg of olive and the crushing time was about 35 min at room temperature (20 °C).

Olive oil processes.
2.2.2 The hammer crusher
Hammer crushing is used in almost all the large scale mills. The olives were introduced into a metal container where a six arm hammer was rotating at a speed of 2890 rpm (Fig. 1). Once properly crushed at room temperature, the ground olives fell through a 0.7 cm grid before going into the malaxation compartment.
2.2.3 Other steps
Malaxation was performed in a metal container with a no-end screw without any heating of the olive paste. This step lasted for 25 min until a good coalescence of the olive oil droplets was achieved. Then the olive paste was placed on fiber disks which are stacked on top of each other and further it was placed into the press where a pressure of 400 bar was applied. This allows the liquid/solid separation and the liquid fraction goes through a centrifuge at a speed of almost 7000 rpm for 10 min. Olive oil obtained was immediately frozen and kept at −18 °C prior to analyses.
2.3 Olive oil analyses
Peroxide values (PVs) were determined according to the AOCS official method Cd 8b-90 [5]. 5 g of olive oil extract was dissolved in a 3:2 acetic acid–chloroform solution and then 0.5 ml of saturated KI solution was added. After 1 min of continuous shaking, 30 ml of distilled water was added to the flasks and the peroxides were titrated with 0.1 N sodium thiosulfate. 0.5 ml of starch solution (5 g/L) was added in order to better see the end point of the titration.
The Free Fatty Acids were determined according to the AOCS official method Ca 5a-40 [6]. For 56.4 g of olive oil, 50 ml of ethanol 96% and 2 ml of phenolphthalein were added and carefully homogenized. The mixture was then titrated with standard 0.1 N sodium hydroxide solution until permanent pink color was seen.
The conjugated dienes were evaluated according to the AOCS official method Cd 7-58 [7]. A 2 g/l solution of olive oil dissolved in n-Hexane was prepared and absorbance was measured at 232 nm. UV–visible spectra were recorded using a HP 8452 diode-array spectrometer equipped with a quartz cell (optical pathlength: 1 cm) thermostated by a water bath.
Total phenolic content of the olive oil extracts was determined using the Folin-Ciocalteu assay [8]. 30 g of olive oil was dissolved in 30 ml n-Hexane. Polyphenols were extracted 3 times with 10 ml of a methanol/water solution (60/40, w/w). The three extracts were combined in a 100 ml flask and the flask was filled with water to the 100 ml mark. To 5 ml of this extract, 2.5 ml of Folin-Ciocalteu reagent was added and the mixture was allowed to stand for 3 min before adding 1 ml of saturated Na2CO3 solution and then completed to 50 ml. Absorbance was measured at 750 nm after 1 h. The amount of polyphenols was calculated using a calibration curve prepared with caffeic acid. For each extract, the analyses were performed in triplicate.
The antioxidant activity of olive oils was determined using the photochemiluminescence (PCL) in which the photochemical generation of free radicals is combined with the sensitive detection by using chemiluminescence. This reaction is induced by optical excitation of a photosensitizer S which results in the generation of the superoxide radical O2− [9]. The free radicals are visualised with the chemiluminescent detection reagent luminol. It works as photosensitizer as well as oxygen radical detection reagent. The olive oils were measured in the Photochem® with the ACL kit (AnalytikJena, Jena, Germany). A 2.2 ml portion of reagent 1 (solvent and dilution reagent), 200 μl of reagent 2 (buffer solution), 25 μl of reagent 3 (photosensitizer), and 100 μl of standard (trolox reagent in reagent 1) or diluted sample (olive oil in methanol) solution were mixed and measured. A light emission curve was recorded over 180 s, using inhibition as the parameter to evaluate antioxidant potential. The antioxidant capacity was then determined by using the integral under the curve and was expressed as mmol/l of trolox per litre of oil. For each extract, the analyses were performed in triplicate.
2.4 Preparation of fatty acid methyl ester derivatives
Fatty acid methyl esters (FAMEs) were prepared according to the AOCS Official Method Ce 2-66 [10]. Oil samples were filtered through a 0.2 μm cellulose regenerated filter (Alltech Associates, Deerfield, IL, USA) and then injected into the gas chromatography capillary. For each oil sample, analyses were performed in triplicate after derivation procedure and mean values are reported.
2.5 Chromatographic analysis
2.5.1 Gas chromatography coupled with mass spectrometry (GC/MS) analysis
FAMEs were separated, quantified and identified by gas chromatography coupled with mass spectrometry (GC/MS). Analyses were performed by using a Shimadzu QP2010 (Kyoto, Japan) gas chromatography. The instrument was equipped with a CP-Wax capillary column 30 m × 0.32 mm × 0.5 μm (Varian) and the velocity of the carrier gas (He) was at 47 cm/s. Injections of 2 μl of the various samples were carried out with a split mode (ratio 1:15) and the injector temperature was set at 250 °C. Oven temperature was initially 60 °C for 1 min and then progressed at a rate of 20 °C/min from 60 °C to 180 °C and then increased from 180 to 230 °C at a rate of 4 °C/min. The temperature was then held during 15 min at 230 °C. The mass spectra were recorded at 3 scan/s from 50 to 400 amu and the ionisation mode was electronic impact at 70 eV. Identification of common fatty acids was performed using the NIST'98 [US National Institute of Standards and Technology (NIST), Gaithersburg, MD, USA] mass spectral database.
2.5.2 Headspace-solid phase micro-extraction/gas chromatography/mass spectrometry (HS-SPME/GC/MS)
The volatiles of the olive oils were estimated using HS-SPME/GC/MS. 4 g of olive oil was put in a 20 ml vial and pre-incubated at 35 °C for 15 min. The volatile compounds contained in the headspace of the vials were then absorbed on a SPME fiber for 30 min under stirring of the oil. The fiber used for absorption and desorption was an SPME fiber 75 μm carboxen–PDMS (polydimethylsiloxane) and the volatile compounds were separated with GC/MS Shimadzu QP2010 (Kyoto, Japan) equipped with AOC 5000 auto-injector. The separation occurred on a capillary column CP WAX 52 CB (10 m × 0.1 mm). The separation was performed as follows: initial oven temperature at 35 °C held for 3 min, then rise at a rate of 5 °C/min until 110 °C followed by an increase at a rate of 10 °C/min until 230 °C and then it was held for the last 5 min of analysis. The mass spectra were recorded at 3 scan/s from 50 to 400 amu and the ionisation mode was electronic impact at 70 eV. The volatile compounds were identified by comparison of their mass spectra and retention times with those of authentic reference compounds. When standards were not available, the tentative identification of compounds was performed using the NIST'98 [US National Institute of Standards and Technology (NIST), Gaithersburg, MD, USA] mass spectral library by comparison of their retention index with those of the literature.
2.6 High performance liquid chromatography analyses
HPLC analyses were carried out at 35 °C on a Waters (Mildford, MA, USA) apparatus equipped with a Model 600 pump and a Model 600 gradient controller, to which were connected a Model 717 autosampler and a Model 996 photodiode-array detector. Separation was achieved on a 5 μm Purospher Star C18 250 × 4.6 mm column with a 5 μm Purospher Star C18 guard column (Merck, Germany). The solvent system used was a gradient of A (H2O/0.05% HCO2H) and B (MeCN). The best separation was obtained with the following gradient: at 0 min 80% A/20% B; at 5 min 60% A/40% B; at 40 min 20% A/80% B; at 45 min 0% A/100% B, at 45 min 0% A/100% B. The solvent flow rate was 1 ml/min. The volume injected was 20 μl. The spectroscopic detection was performed in the range 200–800 nm with a resolution of 1.2 nm. Detection was performed at 280 nm, with the exception of the luteolin and apigenin, which was recorded at 340 nm. The concentrations of polyphenols in the olive oil extracts were estimated from calibration curves (peak area vs. concentration) constructed with tyrosol (R2 = 0.9986), gallic acid (R2 = 0.9965), vanillic acid (R2 = 0.9985), p-coumaric acid (R2 = 0.9986), ferulic acid (R2 = 0.9942), (trans-cinnamic acid) (R2 = 0.9958), apigenin (R2 = 0.9933) and luteolin (R2 = 0.9958), respectively. For each extract, the analyses were performed in triplicate. All runs were acquired and processed using the Empower software (Waters).
2.7 Scanning electron microscopy
Olive fragments were collected at important steps of the olive process: just after crushing and malaxation. After being carefully collected, the samples were put in glutaraldehyde 4% (v/v) in cacodylate sodium buffer in order to fix the structures and were kept in the fridge before being post-fixated with osmium tetroxide (diluted to 1% (v/v)). Samples were then rinsed in water baths followed by dehydration using increasing ethanol baths (30%, 50%, 70%, 90%, and 100%). The last step of the dehydration process was a hexamethyl disilazane bath until full evaporation and the samples were then gold plated before being observed by scanning electron microscopy.
2.8 Statistical analysis
Data represent the mean value of triplicate analysis. The significance of differences at 5% level (or α = 0.05) among means was determined by using the Student test.
3 Results
3.1 Quality parameters
To evaluate the chemical changes in virgin olive oils as a function of crushing systems, several parameters have been determined. Peroxide values, free fatty acids, conjugated dienes percents, total phenolic content and antioxidant activities are presented in Table 1. Preliminary studies on the two different oils showed that the two processes lead the oils to be very little oxidized with low peroxide values compared to most of the previous studies [11,12] and also very low acidity (Table 1). Rotondi et al. [11] have found a high positive correlation between low acidity and a long shelf life of olive oil.
Physicochemical characteristics.
Parameter | Stone mill | Hammer crusher |
Peroxyde value (meq O2/kg) | 5.79 ± 0.21 | 5.40 ± 0.01 |
Free Fatty acids (% oleic acid) | 0.15 ± 0.02 | 0.18 ± 0.01 |
Conjugated dienes (%) | 0.067 ± 0.008 | 0.064 ± 0.004 |
Total phenolics (mg CAE/kg oil) | 416 ± 27 | 563 ± 39 |
Photochem value (mmol trolox/L oil) | 1.608 ± 0.10 | 2.778 ± 0.23 |
No significant differences (α = 0.05) were found in treatments for acidity and peroxide values, and absorbance UV at 232 nm.
The total phenolic contents of the two olive oils were the first step in the polyphenols characterization and were determined as caffeic acid equivalent (CAE). As shown in Table 1 the total phenolic content was highly correlated to the process used. The highest value was found in olive oil issued from the hammer crusher (563 compared to 416 mg CAE/kg, significantly different (α = 0.05)) which may point up a better extraction of polyphenols from the olive fruit when using a hammer. This observation is in accordance with previous works [13,14] that attribute this higher value to the more destructive processes that are the metallic crushers compared to the stone mill.
The antioxidant activities determined by using the photochemiluminescence also show higher values in olive oils obtained from a hammer crusher than those obtained from the stone mill. The correlation between total polyphenolic and antioxidant activity has already been studied widely [15,16].
3.2 Fatty acid composition
The crushing process did not greatly affect the fatty acid composition of the oil (Table 2). This may be due to the high content in olive oils of minor compounds, such as polyphenols, that protect the fatty acids from oxidation or degradation. The main fatty acids are oleic, palmitic, linoleic and stearic respectively as previously reported (Fig. 2). A good ratio between oleic and linoleic acids was found which has already been correlated to a long shelf life of an olive oil [11,12]. Similar ratios were also found in another publication on olive oils from the same area of France [17].
Fatty acid composition (% total fatty acids ± standard deviation).
Fatty acids | Retention time (min) | Stone mill (%) | Hammer crusher (%) |
C14:0 | 9.730 | traces | traces |
C15:0 | 10.850 | traces | traces |
C16:0 | 12.180 | 11.76 ± 0.37 | 12.32 ± 0.27 |
C16:1 | 12.604 | 0.88 ± 0.02 | 1.12 ± 0.26 |
C17:0 | 13.676 | 0.16 ± 0.04 | 0.12 ± 0.05 |
C17:1 | 14.039 | 0.30 ± 0.09 | 0.29 ± 0.11 |
C18:0 | 15.299 | 2.59 ± 0.18 | 2.78 ± 0.08 |
C18:1 | 15.755 | 73.12 ± 0.69 | 73.28 ± 0.42 |
C18:2 | 16.426 | 9.64 ± 0.06 | 9.05 ± 0.15 |
C18:3 | 17.488 | 0.56 ± 0.01 | 0.51 ± 0.06 |
C19:0 | 16.894 | traces | traces |
C20:0 | 18.615 | 0.46 ± 0.03 | 0.28 ± 0.07 |
C20:1 | 18.995 | 0.17 ± 0.13 | 0.07 ± 0.05 |
C21:0 | 20.436 | traces | traces |
C22:0 | 22.597 | 0.06 ± 0.05 | 0.04 ± 0.02 |
C23:0 | 25.314 | traces | traces |
C24:0 | 28.741 | 0.07 ± 0.01 | 0.03 ± 0.01 |
SFA | 15.32 ± 0.13 | 15.69 ± 0.10 | |
MUFA | 74.48 ± 0.23 | 74.76 ± 0.17 | |
PUFA | 10.20 ± 0.04 | 9.56 ± 0.07 | |
C18:1/C18:2 | 7.59 | 8.10 |

GC/MS chromatogram of a fatty acids profile of olive oil.
3.3 Volatile compounds profiles
Table 3 summarises the results obtained by HS-SPME/GC/MS analysis (Fig. 3). The main families of compounds found are alcohols and aldehydes, mostly with a 5 and 6 carbon chains. In fact, the main compounds are hexenal and hexanal which exhibit the “green”, “freshly cut grass” and “sweet” smells in the oil [18,19]. The total C6 compounds represent about 70% of the total volatile compounds and are issued from the lypoxygenase degradation of polyunsaturated fatty acids. C5 aldehydes and alcohols also contribute to the positive attribute of an olive oil by providing “fruity” note, while C5 ketones (especially penten-3-one) bring pungent sensation and is correlated to bitterness [20]. The small amount of esters also may have a positive sensory characteristic on the oil in most cases because it provides a good fruity perception [19]. During settling of the olive oil, the thin layer of wastewater at the bottom of the bottle has probably induced a little fermentation which explains the presence of ethyl acetate and ethanol in some sample. The amount of these volatiles might not be very high in the oil but as they are very volatile, they were easily absorbed on the fiber during the HS-SPME phase which induced quite high values in the final results.
Volatile compounds composition as determined by SPME fiber (% total volatiles ± standard deviation).
Volatile compounds | RI sample | RI standard | RI literature | Stone mill (%) | Hammer crusher (%) |
Ethyl acetate | 920 | 893a | 1.3 ± 0.13 | 2.1 ± 0.13 | |
3-Pentanone | 975 | 980a | 3.1 ± 0.01 | 5.1 ± 0.30 | |
1-Penten-3-one | 1012 | 1012 | 1.7 ± 0.08 | 3.9 ± 0.05 | |
Hexanal | 1069 | 1073 | 15.8 ± 0.99 | 11.1 ± 0.39 | |
(Z)-3-Hexenal | 1134 | 1132b | 2.0 ± 0.41 | 2.1 ± 0.14 | |
1-Penten-3-ol | 1153 | 1154 | 3.4 ± 0.38 | 4.5 ± 0.28 | |
(E)-2-Hexenal | 1208 | 1209 | 31.5 ± 0.80 | 25.1 ± 1.51 | |
(E)-3-Hexenyl acetate | 1313 | 1308b | 4.8 ± 0.22 | 4.2 ± 0.05 | |
(E)-2-Pentenol | 1317 | 1335a | 2.9 ± 0.25 | 3.2 ± 0.03 | |
Hexanol. | 1348 | 1351b | 3.6 ± 0.06 | 3.9 ± 0.02 | |
(Z)-Hex-3-en-1-ol. | 1378 | 1378 | 9.2 ± 0.08 | 9.3 ± 0.98 | |
(E)-2-Hexen-1-ol | 1400 | 1400b | 2.3 ± 0.36 | 1.9 ± 0.06 |
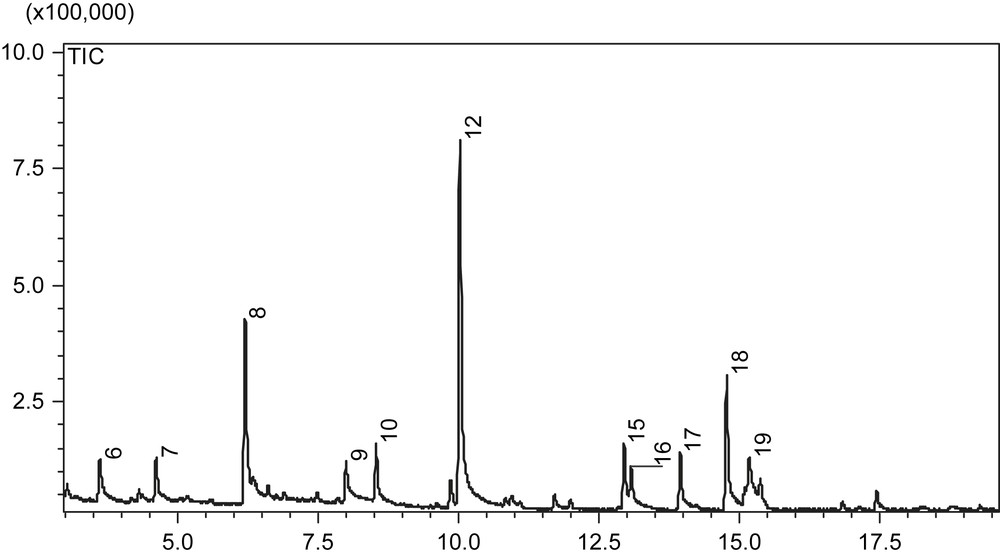
GC/MS chromatogram of a volatile compounds profile in olive oil. Peak numbers of the main compounds: (6)3-pentanone; (7)1-penten-3-one; (8)hexanal; (9)(Z)-3-hexenal; (10)1-penten-3-ol; (12)(E)-2-hexenal; (15)(E)-3-hexenyl acetate; (16)(E)-2-pentenol; (17)hexanol; (18)(Z)-hex-3-en-1-ol; (19)(E)-2-hexen-1-ol.
3.4 Polyphenolic composition
To complete the study, the changes in the amount of individual polyphenols were considered. Only simple polyphenols and flavonoids are reported here. The tentative of identification in both types of olive oil extracts was carried out by HPLC/DAD (Fig. 4). The presence of polyphenols was determined using comparison of retention time and the UV-spectrum of the authentic standard. Thus, tyrosol, gallic acid, vanillic acid, p-coumaric acid, ferulic acid, trans-cinnamic acid as simple phenols whereas, apigenin and luteolin as flavonoïds, were easily identified and quantified thanks to the existence of commercially available standards (Fig. 5). The biophenolic composition, retention time and maximum absorbance of olive oil obtained with the two milling processes are given in Table 4. The amounts of polyphenols were quite similar within each sample, with only the vanilic acid from the stone milling process showing a slightly higher content (Table 4). The results show also that luteolin and tyrosol were the most abundant polyphenolic compounds in olive oil.
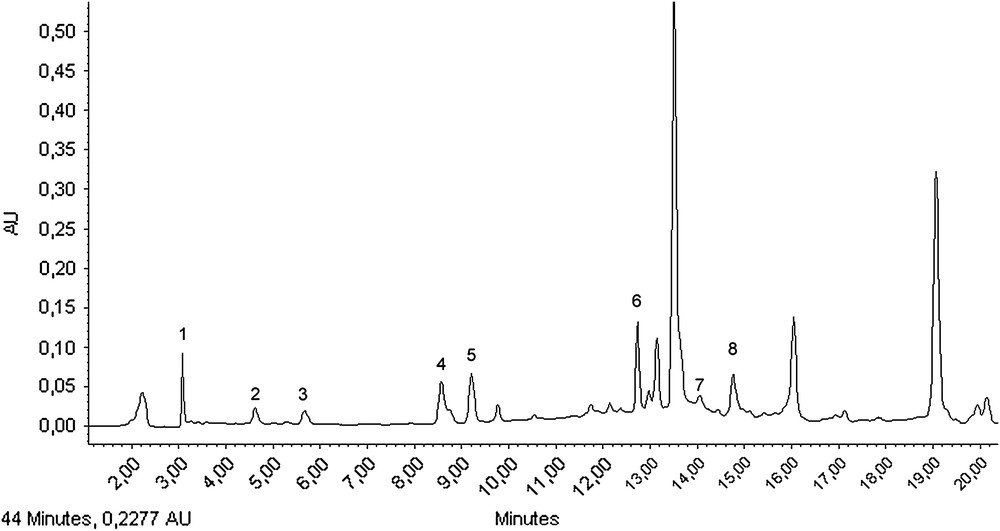
HPLC-DAD chromatogram of phenolic composition of olive oil. (1) Gallic acid; (2) tyrosol; (3) vanillic acid; (4) p-coumaric acid; (5) ferulic acid; (6) luteolin; (7) trans-cinnamic acid; (8) apigenin.

Polyphenolic compounds identified in olive oils.
Retention time (Rt), λmax (nm) and quantification (mg/kg) of polyphenolic compounds identified in the olive oils.
No. | Compounds | Rt (min) | λmax (nm) | Stone (mg/kg) | Hammer (mg/kg) |
1 | Gallic acid | 3.1 | 226–272 | 1.60 ± 0.20 | 1.32 ± 0.14 |
2 | Tyrosol | 4.6 | 225–277 | 2.99 ± 0.17 | 3.00 ± 0.54 |
3 | Vanillic acid | 5.6 | 261, 292 | 1.83 ± 0.12 | 1.27 ± 0.12 |
4 | p-Coumaric acid | 8.4 | 230, 310 | 2.25 ± 0.16 | 1.97 ± 0.42 |
5 | Ferulic acid | 9.2 | 235, 323 | 1.95 ± 0.12 | 1.62 ± 0.22 |
6 | Luteolin | 12.7 | 253, 348 | 4.66 ± 0.25 | 4.20 ± 0.31 |
7 | trans-Cinnamic acid | 14.1 | 215, 277 | 0.12 ± 0.01 | 0.11 ± 0.01 |
8 | Apigenin | 14.7 | 224, 267, 337 | 1.64 ± 0.17 | 1.61 ± 0.14 |
3.5 Scanning electron microscopy
Olive fragments collected at important steps of the olive process (crushing and malaxation) were observed by SEM (Fig. 6) in order to see the impact of the crushing process at the cellular scale. The most important criteria were the inter-cellular structure of the olive and the presence or absence of olive oil droplets. As shown in Fig. 6a the inter-cellular structure of the olive shelf remained in its native form after being crushed by a hammer. The hammer had only cut and opened the olive fruit but without destroying the inter-cellular structure (the cellular organisation can easily be seen). In the middle of picture Fig. 6a an olive oil droplet releasing from the cell matricia could be observed. Once released, the droplets had a spherical appearance in SEM. Contrary to what was observed in the hammer crushing process, the granite milling destroyed the inter-cellular structure as it is highlighted by Fig. 6b. In this picture it can be seen that the different layers of olive cells have sustained a compression and it is easily understandable that some olive oil droplets could be retained within these layers, which can explain the lower yield usually observed while using this type of process.

(a) SEM scan at 10 kV of the olive fragment after being crushed by a hammer, magnification ×2000 on original picture scale, 124 μm total picture width. (b) SEM scan at 10 kV of the olive fragment after being crushed by a stone, magnification ×4000 on original picture scale, 62 μm total picture width. (c) SEM scan at 5 kV of the olive fragment after the malaxation step, magnification ×150 on the original picture scale, 1680 μm total picture width.
From the malaxation step to the end of the process, no significant difference was observed when comparing the olive fragments issued from one process or the other. Fig. 6c showed that the olive oil droplet coalescence happened during malaxation, when the olive cells have already been emptied from their intra-cellular content.
This study showed that the type of crushing could influence what happened on a cellular scale: the higher production yields usually observed after a hammer crushing can be explained both by better cell cuts and by the olive oil droplets that could be retained within the olive cell layers when using a granite stone.
4 Conclusion
For the first time, olive fruits were studied by scanning electron microscopy and the olive oils tested for their composition as a function of crushing systems, i.e. stone mill and hammer crusher. From the above, it can be concluded that the hammer crusher appeared to give high quality of olive oil rich in phenolic compounds known as antioxidants.
In general, the scanning electronic microscopy observations indicated two distinct extraction mechanisms for the two olive oil processes: the first one involved diffusion of the oil across the opened cells which have been clearly cut (hammer crushers), and the second one involved exudation of oil from broken and damaged cell walls due to a strong mechanical effect (stone mill).
Acknowledgments
The authors acknowledge the region P.A.C.A. for the financial support, the association FEMOS and ENIGMA S.A.RL. for their financial support and its raw material supply. The authors are thankful to the mill “le petit Moulin” in Travaillan (Vaucluse, France) for its technical assistance. The authors also want to acknowledge Christian Ginies (INRA Avignon, France) for GC/MS analyses.