1 Introduction
Interest in long carbon chains continues as their possible occurrence in cryogenic matrices or in the diffuse stellar medium becomes apparent. Several theoretical treatments of these species are available at varying degrees of sophistication. The study of compounds containing carbon chains end-capped by redox-active transition metal moieties continues on account of their potential as models for molecular wires [1], which involves an understanding of ways in which electrons (or holes) might transfer from one end to the other through the carbon atoms of the chain [2,3]. In addition, the synthetic challenges of making such complexes, with either even- or odd-numbered chains, or with different end-caps, provide when successful, fascinating and useful additions to the chemist's armoury [4].
Electronically, the structures of the M–C2x–M complexes have been shown to encompass arrangements which, neglecting any radical contributions, may be written in valence-bond terms as A, B or C (Fig. 1). The major difference between these forms is found in the mode of attachment to the metal–ligand fragment, which is by M–C single, M C double or M≡C triple bonds, respectively. In consequence, the carbon–carbon bonds in the Cx chain have varying multiplicities. The redox properties of some complexes, e.g., those with Mn, Fe, Ru, Os or Pt endgroups, have been shown to provide routes to complexes of types B and C, examples being the species [{Ru(PP)2Cp’}2(μ-C4)]n+ [n = 0–4; PP = (PPh3)2, dppe; Cp’ = Cp, Cp*] [5]. The conversion of diyndiyl complexes {Cp(OC)3M}–C≡C–C≡C–{M’(CO)3Cp} (M, M’ = Mo, W) to the ethyndiyl-bridged carbyne clusters {Cp(OC)8Co2M}≡C–C≡C–C≡{Co2M’(CO)8Cp} upon treatment with Co2(CO)8 provides a second example of electronic redistribution within the M–C2x–M framework [6].
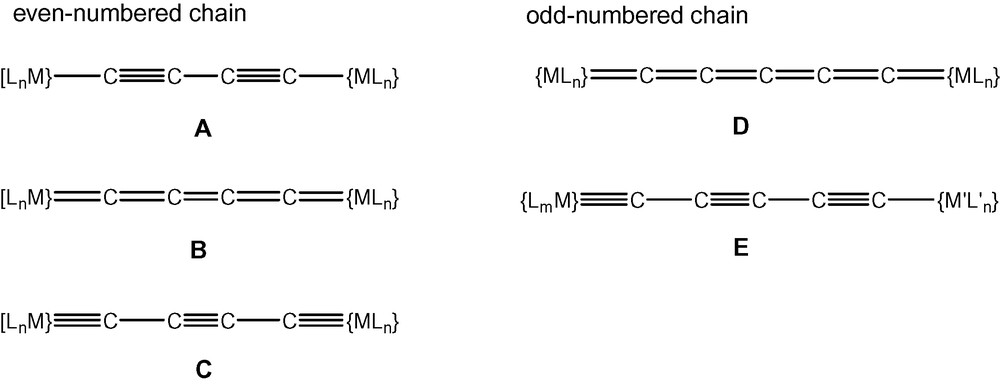
A variety of methods is available for the generation of compounds containing long carbon chains linking transition metal–ligand fragments, some of which are redox-active: several complexes containing chains with ten or more carbon atoms have been described [7–14]. Among these, the elegant studies of Gladysz et al. have resulted in the syntheses of {Re(NO)(PPh3)Cp*}2(μ-C2x) (x = 5–10) [7] and more recently, the series trans,trans-{Pt(C6F5)[P(tol)3]2}2(μ-C2x) (x = 5–8, 10, 12, 14), the C28 derivative containing the longest C(sp) chain so far characterised in this type of complex [12a].
Synthetic approaches to these intriguing compounds are iterative and generally result in compounds with an even-numbered C2x chain because common synthons are based on the C2 unit. Alternatively, a facile approach to lengthening the chain is by coupling two singly metal-capped poly-ynes, but even with odd-numbered chains as precursors, the final products contain an even number of carbon atoms in the bridge.
Consideration of the structures of compounds containing odd-numbered carbon chains shows that all require at least one multiple M–C bond, i.e., the M C double bond at each end of the chain in the metallacumulene D, or the M≡C triple bond at one end of the poly-ynylcarbyne derivative E (Fig. 1). Consequently, synthetic routes to these compounds are more limited than those available for types A–C, the availability of suitable bis-carbene complexes, for example, being presently limited to the Group 6 complexes prepared by Templeton et al. [15]. Several halocarbyne derivatives are known, however, and these compounds are relatively easily converted to compounds containing odd-numbered carbon chains end-capped (necessarily) by two different metal-ligand groups. Exchange of the carbyne halogen for alkynyl groups is easily accomplished by reactions with terminal alkynes in the presence of amines, or by reactions with lithiated alkynes.
We have recently described a new methodology whereby complexes containing carbon chains end-capped by two metal centres may be obtained by elimination of phosphine-gold(I) halides in reactions between alkynyl- or poly-ynyl-gold(I) phosphine complexes and compounds containing C(sp)-halogen bonds [9b]. The reactions, which are related to the well-known Sonogashira reaction, proceed under mild conditions and in the absence of base (which has been shown to react readily with CCo3 clusters, for example). We have used this approach to generate carbon chain complexes containing up to 26 atoms which may have two metal-ligand end-caps, either identical or different, and which may have even- or odd-numbers of carbon atoms, according to the nature of the end-caps [11a,16,17]. We have sought to apply this reaction to the synthesis of specific complexes and this note describes some novel chemistry found when we targeted a complex containing a C19, chosen to honour Pr Pierre Dixneuf, whose work in the area of carbon-rich compounds is well-known [18]. To the best of our knowledge, there is no previous example of a C19 complex [the longest odd-numbered carbon chains yet described are in {Cp(OC)3W}(μ:μ3-C≡CC≡CC){Co3(μ-dppm)n(CO)9–2n} (n = 0 [19], 1 [16]), {Cp*(dppe)Ru}{μ:μ3-(C≡C)xC}{Co3(μ-dppm)(CO)7} (x = 2 [19], 4 [9b]) and Os3(μ-H)3{μ3–C(C≡C)3Fc}(CO)7 [17], which contain C5, C7 or C9 chains].
We have explored a variation of these reactions which allows linking of two phosphine-gold(I) derivatives of metalla-poly-ynes, which may be the same or different, with diiodopoly-ynes [20]. This route affords complexes containing satisfyingly long carbon chains with each end capped. If a mixture of precursors is used, coupling may proceed to give any or all of several products, as found earlier when different Re complexes were oxidatively coupled to produce all possible products, some of which could be obtained preferentially by suitable tweaking of the conditions [7b,21]. The disadvantage of obtaining a mixture of complexes can be addressed if efficient separation methods are available. As shown below, high yields overall of several products can be obtained.
An alternative route to compounds containing long many-atom chains is by coupling with the aid of a second type of atom. Thus, by taking advantage of the linear sp hybridisation shown by two-coordinate Au(I) or Hg(II), it is possible to construct complexes containing chains of carbon atoms linked by single atoms of these elements. Previously, we have described the syntheses of complexes such as Hg{C≡CC≡C[MLn]}2 [MLn = Ru(dppe)Cp*, CCo3(μ-dppm)(CO)7], containing nine- and 11-atom chains respectively [11b,22]. Below we describe an extension to an analogous tricobalt cluster complex containing the 19-atom C9–Hg–C9 chain.
2 Results and discussion
2.1 A complex containing a C19 bridging ligand
Our approach has been to use double eliminations which would be possible between appropriate known poly-ynyl-gold(I) complexes and the recently described di-iodo-poly-ynes, I(C≡C)xI (x = 3 or 4) [20,23]. Use of I(C≡C)ySiMe3 gives products containing y extra C2 units, the iodo-poly-ynylsilanes also being readily available [24]. A sequence of reactions which is generally applicable consists of chain extension by coupling a poly-ynyl-gold(I) phosphine complex R(C≡C)xAu(PPh3) 1 with I(C≡C)ySiMe3 to give R(C≡C)x+ySiMe3 2. In turn, this complex may be converted to the corresponding gold(I) derivative R(C≡C)x+yAu(PPh3) 3. Either 2 or 3 may be employed in a similar sequence to generate further examples of complexes containing longer chains. We have found that this sequence is convenient for the synthesis of derivatives in which R = W(CO)3Cp or CCo3(μ-dppm)(CO)7, for example (Scheme 1).
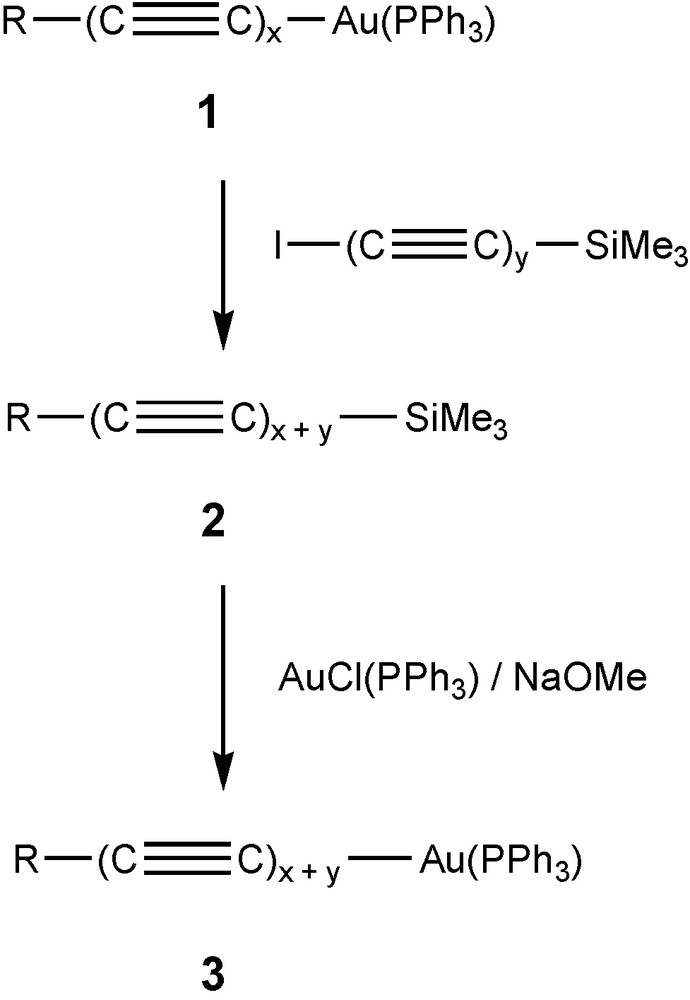
Based on these reactions, we considered that three-component reactions between the diiodopoly-ynes I(C≡C)nI (n = 3 or 4) and two different metal-capped poly-yne reagents might produce the desired products. For example, the reaction between 1,6-di-iodohexatriyne and an equimolar mixture of the two gold-containing precursors W{C≡CC≡CAu(PPh3)}(CO)3Cp 4 [16] and Co3{μ3-C(C≡C)4Au(PPh3)}(μ-dppm)(CO)7 5 [11b] offered the possibility of a (C4 + C6 + C9) = C19 synthesis (Scheme 2). In fact, we have found that this reaction, which was carried out in thf, afforded several compounds, which included both hetero- and homocoupled products either with or without the C6 fragment from the hexatriyne. These were easily separated by preparative t.l.c. on silica gel, and we have identified the following complexes: [W]–C13–[Co3] (6), [W]–C19–[Co3] (7), [Co3]–C18–[Co3] (8) and [Co3]–C24–[Co3] (9) (where [W] = W(CO)3Cp, [Co3] = Co3(μ-dppm)(CO)7). In independent experiments, we have shown that the relatively unstable [W]–C8–[W] and [W]–C14–[W] complexes are also likely to have been formed, although we have not unequivocally isolated and characterised these species from the mixed reaction.
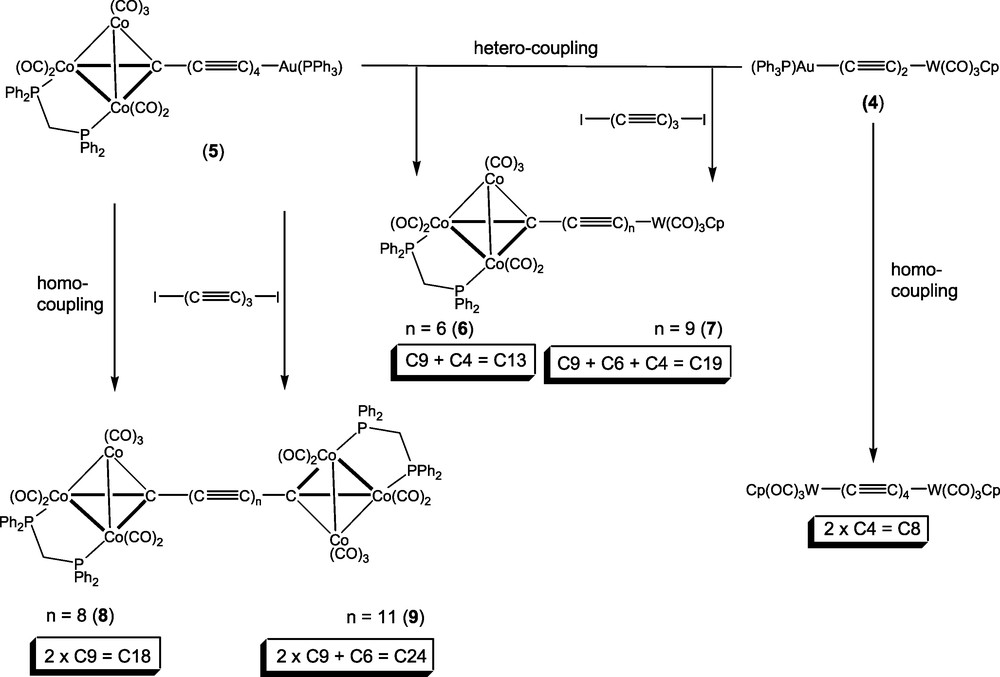
The complexes have been identified by the usual spectroscopic methods but not so far by X-ray crystal structural studies, as they form fine fibres unsuitable even for synchrotron studies. In particular, the IR ν(CO) spectra are essentially identical, being composed of either Co3(CO)7 or of superimposed W(CO)3 + Co3(CO)7 patterns. The anticipated ν(C≡C) absorptions are all very weak and appear as either one or two broad bands at ca 2100 cm-1. We have been unable to obtain 13C NMR spectra which show resonances of the chain carbon atoms, although we would not expect to find the Co3C signal as a result of broadening by the 57Co quadrupole [25–27]. The presence of appropriate M+, [M + Na]+ and / or [M + OMe]– ions in the positive and negative ion ES–MS spectra, together with elemental analytical results, confirm the compositions of these materials. Interestingly, complexes with longer carbon chains are more soluble but less stable than analogous compounds containing shorter chains.
The homometallic products 8 and 9 were characterised from their ES–MS, with negative ions at m/z 1729 and 1833, respectively, assigned to [M − H]–, and a positive ion at m/z 1753 (for 8), assigned to [M + Na]+. Complex 8 was identical with a sample previously prepared [11b]. Their formation suggests that an alternative course of the reaction between the alkynyl-gold(I) complex and the di-iodo-poly-yne is that of oxidative homocoupling, perhaps of radical species derived by initial elimination of the Au(PPh3) group as the iodide, or by oxidation of intermediate palladium complexes [28]. In both 8 and 9, the IR ν(CO) spectra were characteristic of the Co3(CO)7 cluster fragments, while other spectroscopic and analytical data are also consistent with the above formulations.
2.2 A complex containing the 19-atom C9–Hg–C9 chain
We have also utilised coupling of carbon chains through mercury atoms, the well-established linear geometry of the Hg(II) atom providing a facile means of doubling the chain length of precusors by incorporation of a heteroatom, here Hg, in the centre. Earlier, we showed that the reactions between Hg(OAc)2 and Co3{μ3-C(C≡C)nSiMe3}(μ-dppm)(CO)7 (n = 1, 2) in the presence of NaOMe afforded Hg{(C≡C)nC[Co3(μ-dppm)(CO)7]}2, containing 7- and 11-atom chains, respectively, which are end-capped by the Co3 clusters. Extension of this methodology to Co3{μ3–C(C≡C)4SiMe3}(μ-dppm)(CO)7 afforded Hg{(C≡C)4C[Co3(μ-dppm)(CO)7]}2 10 (Scheme 3), which contains a 19-atom C9–Hg–C9 chain linking the two Co3 clusters. Conventional work-up using preparative t.l.c. gave 10 as a brown powder in 25% yield. Characterisation was by ES–MS which contain [M + Na]+ and [M – H]– in the positive and negative ion mode spectra, respectively, supported by microanalysis. Terminal ν(CO) bands were found in the IR spectrum between 2068 and 1957 cm-1, with the w-m-vs pattern found previously for the Co3(μ-dppm)(CO)7 fragment. These were accompanied by weaker absorptions at lower frequencies and two very weak ν(C≡C) bands at 2135 and 2119 cm-1. The 1H and 31P NMR spectra contained resonances arising from the dppm ligands, the single 31P resonance confirming that the molecule is centrosymmetric. Low solubility precluded our obtaining a 13C NMR spectrum.
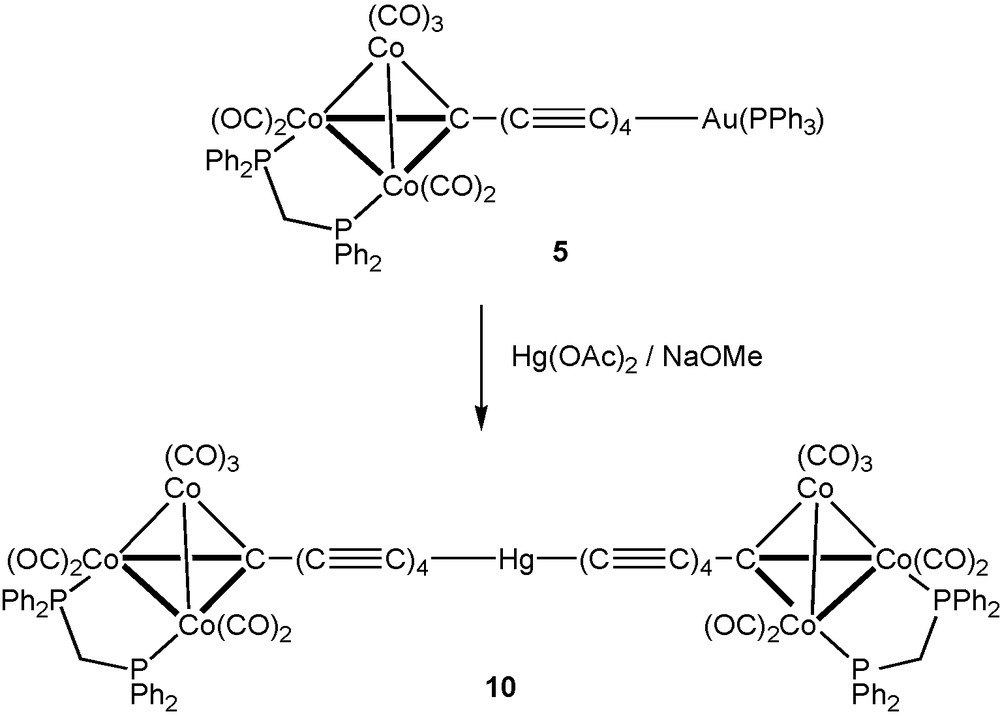
In summary, we have prepared and characterised two novel complexes 7 and 10 containing 19-atom chains bridging transition metal–ligand fragments. The first was obtained from a three-component reaction in which 1,6-diiodohexatriyne is coupled with phosphine-gold(I) complexes 4 and 5. Several other products, containing Cx chains (x = 13, 18, 24) were also formed. Two shorter chain complexes 6 and 8 are derived from “oxidative” coupling of one Co3–C9 with one W–C4, or of two Co3–C9 fragments without involvement of the hexatriyne, whereas the longer carbon chains in 7 and 9 have incorporated one C6 moiety from the triyne. Although the reactions are complex in the sense that coupling between any two of the components has occurred, as well as the desired three-component coupling, it is gratifying that the compounds formed in highest yield are the two heterocoupled complexes 6 and 7, again showing that the gold halide-elimination reaction that we have found has wide applicability.
In 6 and 7, the end-caps are different, with a W(CO)3Cp group being attached by a W–C single bond at one end, and a Co3 cluster interacting with the other terminal C atom through all three Co atoms. In 10, the C9–Hg–C9 chain is symmetrically end-capped by two Co3(μ-dppm)(CO)7 clusters.
3 Experimental
3.1 General experimental conditions
All reactions were carried out under dry, high purity nitrogen using standard Schlenk techniques. Common solvents were dried, distilled under nitrogen and degassed before use.
3.2 Instrumentation
Infrared spectra: a Bruker IFS28 FT-IR spectrometer. Spectra in CH2Cl2 were obtained using a 0.5 mm path-length solution cell with NaCl windows. NMR spectra: Bruker AM300WB or ACP300 instruments (1H at 300.13 MHz, 31P at 121.503 MHz). Samples were dissolved in C6D6, unless otherwise stated, contained in 5 mm sample tubes. Chemical shifts are given in ppm relative to internal tetramethylsilane for 1H and NMR spectra and external H3PO4 for 31P NMR spectra. ES mass spectra: VG Platform II or Bruker MicroTOF. Solutions in MeOH containing NaOMe as an aid to ionisation [29] were directly infused into the instrument. Elemental analyses were performed at CMAS, Belmont, Australia.
3.3 Reagents
The compounds I(C≡C)nI (n = 3, 4) [20], IC≡CC≡CSiMe3 [24], W{C≡CC≡CAu(PPh3)}(CO)3Cp [16] and Co3{μ3–C(C≡C)4Au(PPh3)}(μ-dppm)(CO)7 [11b] were made by the cited methods.
3.4 Syntheses of complexes containing 19-atom chains
3.4.1 C19. Reaction of I(C≡C)3I, W{C≡CC≡CAu(PPh3)}(CO)3Cp 4 and Co3{μ3-C(C≡C)4Au(PPh3)}(μ-dppm)(CO)7 5
A mixture of W{C≡CC≡CAu(PPh3)}(CO)3Cp 4 (31.7 mg, 0.038 mmol), Co3{μ3-C(C≡C)4Au(PPh3)}(μ-dppm)(CO)7 5 (50 mg, 0.038 mmol), I(C≡C)3I (12.4 mg, 0.038 mmol), CuI (1 mg, 0.005 mmol) and Pd(PPh3)4 (5 mg, 0.004 mmol) in thf (20 ml) was stirred at –50 °C for 30 min, after which it was allowed to warm to r.t., stirring being continued for a further 2 h. The dark red solution was evaporated in vacuum and the residue was dissolved in dichloromethane and the products were separated by preparative t.l.c. (silica gel, dichloromethane / hexane 2/3). Several coloured bands developed:
- • Band 1 (yellow, Rf 0.79) contained AuI(PPh3) (20.2 mg, identified by comparison with an authentic sample) and other yellow products, which were not investigated further;
- • Band 2 (purple, Rf 0.63) gave {Co3(μ-dppm)(CO)7}2{μ3:μ3–C(C≡C)11C} (9) as a dark purple solid (3.4 mg, 5%). IR / cm–1: ν(C≡C) 2170w, 2122w; ν(CO) 2090w, 2064 s, 2017vs, 1976 (sh), 1935 s (br). ES–MS (negative ion, m/z): 1833, [M + OMe]–; 1805, [M + OMe – CO]–; 1801, [M – H]–. This complex is not stable in solution and decomposes on the plates;
- • Band 3 (dark red, Rf 0.60) afforded {Co3(μ-dppm)(CO)7}2{μ3:μ3–C(C≡C)8C} (8) (4.1 mg, 6%), identified by comparison with an authentic sample [11b];
- • Band 4 (orange, Rf 0.28), contained {Co3(μ-dppm)(CO)7}{μ3:μ-C(C≡C)9}{W(CO)3Cp} (7) as a brown solid (11.2 mg, 22%). Anal. Found: C, 53.74; H, 2.13. Calcd (C59H27Co3O10P2W): C, 53.72; H, 2.05%; M, 1318. IR / cm-1: ν(C≡C) 2160w, 2119w; ν(CO) 2096vw, 2063 s, 2042m, 2016vs, 1987m, 1966m (br), 1912w (br). 1H NMR: δ 3.03, 4.02 (2 × m, 2 × 1H, CH2), 4.26 (s, 5H, Cp), 6.73–7.42 (m, 20H, Ph). 31P NMR: δ 34.76 [s (br), dppm]. ES–MS (negative ion, m/z): 1349, [M + OMe]–; 1317, [M – H]–; (positive ion, m/z): 1341, [M + Na]+;
- • Band 5 (yellow, Rf 0.23), afforded {Co3(μ-dppm)(CO)7}{μ–C(C≡C)6}{W(CO)3Cp} (6) as a brown powder (15.1 mg, 32%). Anal. Found: C, 50.95; H, 2.09. Calcd (C53H27Co3O10P2W): C, 51.04; H, 2.17%; M, 1246. IR / cm-1: ν(C≡C) 2147vw, 2126w; ν(CO) 2080vw, 2062vs, 2041 s, 2016vs, 1989m (br), 1962 s (br). 1H NMR: δ 2.98, 3.92 (2 × m, 2 × 1H, CH2), 4.27 (s, 5H, Cp), 6.75–7.40 (m, 20H, Ph). 31P NMR: δ 34.82 [s (br), dppm]. ES–MS (negative ion, m/z): 1277, [M + OMe]–; 1245, [M – H]–; (positive ion, m/z): 1269, [M + Na]+.
Further t.l.c. of the reaction mixture afforded a yellow band (Rf 0.21) which travelled with some decomposition to a brown “tail”. IR / cm-1: ν(C≡C) 2138w, 2115vw; ν(CO) 2041 s, 1989w, 1956vs (br). The t.l.c. behaviour and IR spectrum are consistent with this material being {W(CO)3Cp}2(μ-C8) [30].
3.4.2 C9HgC9. Hg{(C≡C)4C[Co3(μ-dppm)(CO)7]}2 10
NaOMe was added to a solution of Co3{μ3–C(C≡C)4SiMe3}(μ-dppm)(CO)7 (25 mg, 0.026 mmol) and Hg(OAc)2 (4.3 mg, 0.013 mmol) in thf / MeOH (1/2). After stirring 1 h at r.t. reaction was complete. Work-up by preparative t.l.c. (acetone-hexane, 3/7) afforded a dark red band (Rf 0.30) containing {Co3(μ-dppm)(CO)7}2(μ-C18) 8 (2.1 mg, 9%), identified by comparison with an authentic sample [11b]. A brown band (Rf 0.26) yielded Hg{(C≡C)4C[Co3(μ-dppm)(CO)7]}2 10 (6.4 mg, 24.5%) as a brown powder. Anal. Found: C, 50.89; H, 2.35. Calcd (C82H44Co6HgO14P4): C, 51.00; H, 2.30; M, 1930. IR (CH2Cl2, cm-1): ν(C≡C) 2135vw, 2119vw; ν(CO) 2068w, 2045m, 2017vs, 1989 (sh), 1977 (sh), 1957 (sh). 1H NMR (CDCl3): δ 3.66, 4.24 (2 × m, 4H, CH2), 7.27–7.65 (m, 40H, Ph). 31P NMR (CDCl3): δ 35.0 [s (br), dppm]. ES–MS (positive ion, m/z): 1954.733 (calcd 1954.729), [M + Na]+; (negative ion, MeOH, m/z): 1930.736 (calcd 1930.733), [M − H]−.
Acknowledgements
We thank the ARC for support of this work.