1 Introduction
Accurate enzyme kinetic parameters are highly desirable for metabolic kinetic analysis [1]. Usually purified enzymes are utilized to characterize their kinetic behavior in unnatural buffer systems. Unfortunately, these artificial conditions usually cannot provide correct information on how an enzyme fulfils its physiological role in the living cell [2]. There is plenty of evidence that channeling, i.e. the preferential direct transfer of a product of an enzymatic reaction to the next enzyme, is an important phenomenon that cannot be studied with purified enzymes. Recent protein localization studies in Arabidopsis thaliana showed, e.g. that the dynamic association of glycolytic enzymes with mitochondria is a function of mitochondrial activity thus supporting channeling [3]. Channeling was also shown for glycolysis even in bacteria [4]. Application of cell-free extracts obtained from the water-soluble part of the cell lysate is the classical way for intracellular enzyme study, but the requirement of complete disruption of the cell integrity may result in an inactivation of enzymes and dissociation of enzyme complexes, especially those associated more weakly. In addition, the procedure for purifying enzymes or preparation of cell-free extract is laborious and time consuming [5]. Permeabilization of the cellular membrane without complete cell disintegration promises to solve above mentioned problems to a certain extent. After the treatment of cells with appropriate organic solvents, such as ethanol [6], toluene-ethanol mixtures [7,8], detergents like Triton X-100 [5,9] and other organic chemicals [10,11], the cell membrane becomes permeable allowing small molecules to freely enter and leave the cell while keeping the morphology of the cell intact. This method permits keeping most cellular structures, protein-protein interactions, and most of intracellular enzymes in their original environment which is very important for metabolic processes analysis [12]. Kinetics measured under permeabilization conditions, which is often called in situ, is regarded as an intermediate stage between in vivo and in vitro. Compared to in vitro methods, permeabilized cells still keep their macromolecular structure intact that is important for channeling and protein-protein interaction phenomena.
Many intracellular oxidation-reduction reactions involve the inter-conversion of the oxidized and reduced forms of NAD(P), and for monitoring such reactions, the large absorbance of NAD(P)H at 340 nm can be measured continuously by a spectrophotometer. Normally such spectrophotometric assays need an optically clear reaction system for a detection linear with concentration of NAD(P)H. Therefore, NAD(P)H-dependent enzyme assays in a cell suspension system have not yet been deeply studied.
Whole-cell permeabilization for enzyme activity measurement has been applied in many organisms until now [6,7,9,10,13–15]. Corynebacterium glutamicum is intensively used for the industrial production of amino acids such as glutamate and lysine [16–18]. The method mostly used for enzyme activity measurement in such cells uses cell lysis [19–21]. In vivo activity of the enzyme of the entire metabolic network is determined using various methods of metabolic flux analysis [19,22–30].
In this investigation, we focus on NAD(P)H-dependent enzyme assays in a cell suspension system, in particular on obtaining a rapid, simple permeabilization method in C. glutamicum for potential determination of enzymatic kinetic parameters which are important for metabolic pathway analysis and modeling. The effect of the nonionic surfactant Triton X-100, toluene-ethanol mixtures (TE), CTAB, digitonin and ethanol on the permeability of C. glutamicum cells was examined and four enzyme activities, glucose-6-phosphate dehydrogenase, isocitrate dehydrogenase, 6-phosphogluconate dehydrogenase and malic enzyme, were analyzed as model systems.
2 Materials and methods
2.1 Strain
Corynebacterium glutamicum ATCC 13032 was obtained from the American Type Culture Collection (Manassas, USA).
2.2 Chemicals
Triton X-100 was purchased from Fluka (Buchs, Switzerland). Malate, NADP and glucose-6-phosphate were from Sigma (Steinheim, Germany). All other chemicals were from Sigma (St. Louis, USA), Merck (Darmstadt, Germany) or Fluka (Buchs, Switzerland) and of analytical grade. For all experiments the same batch of chemicals was used.
2.3 Strain cultivation
The cultivation of C. glutamicum ATCC 13032 was the same as described in [28]. Cells in the exponential growth phase (OD660 = 10) were harvested by centrifugation (8500 rpm, 5 min, 4 °C), and then washed by Tris–HCl buffer (100 mM Tris, 200 mM KCl, pH = 7.8) for twice. The pellet was weighted and appropriate volume of Tris–HCl buffer was added and adjusted to the required cell density (mg cell dry weight/ml) for the next cell disruption.
2.4 Preparation of crude extract and permeabilization
2.4.1 Permeabilization
Washed cells were resuspended to a cell density of 10 mg/ml (CDW), 1 ml of cell suspension was transferred into a 1.5 ml Eppendorf tube and a defined amount of permeabilization agent was added to give a desired concentration. After 30 s swirling the cell suspension was incubated for a defined period of time. The final permeabilized cell suspension was directly used for enzyme activity measurements.
2.4.2 Glass beads treatment
Washed cells were resuspended to a cell density of 10 mg/ml(CDW), 400 μl cell suspension and 400 mg glass beads (≤0.25 mm in diameter) were mixed in a 1.5 ml Eppendorf tube and shaken for 15 min (Frequency: 30/s). The supernatant was transferred into another pre-cooled Eppendorf tube and centrifuged at 13000 rpm (15500g) for 15 min. The final supernatant was used for enzyme activity measurement. Samples were kept at 4 °C.
2.5 Enzymes assays
All of the following enzyme activities were measured spectrophotometrically by detecting the change in the absorption of NADPH at 340 nm (ɛ = 6.223 l mmol−1 cm−1) at 30 °C.
2.5.1 Malic enzyme
The 1 ml reaction system contains 890 μl Tris–HCl buffer (100 mM Tris–HCl, 200 mM KCl, pH = 7.8), 10 μl MgCl2 solution (2 mM), 10 μl NADP solution (1 mM) and 50 μl cell extract or permeabilized cell suspension. 40 μl of malate solution was added to start the reaction.
2.5.2 Glucose-6-phosphate dehydrogenase
The 1 ml reaction system contained 880 μl Tris–HCl buffer (100 mM Tris–HCl, 200 mM KCl, pH = 7.8), 10 μl MgCl2 solution (10 mM), 10 μl NADP solution (1 mM) and 50 μl cell extract or permeabilized cell suspension. 50 μl of glucose-6-phosphate was added to start the reaction.
2.5.3 Isocitrate dehydrogenase
The 1 ml reaction system contained 915 μl Tricine–KOH buffer (100 mM Tricine–KOH, pH = 8.0), 10 μl MgCl2 solution (10 mM), 5 μl NADP+ solution (1 mM) and 50 μl cell extract or permeabilized cell suspension. 20 μl of dl-isocitric acid solution was added to start the reaction.
2.5.4 6-Phosphogluconate-dehydrogenase
The 1 ml reaction system contained 910 μl Tris–HCl buffer (100 mM Tris–HCl, pH = 7.5), 10 μl MgCl2 (10 mM), 10 μl NADP (1 mM) and 50 μl cell extract or permeabilized cell suspension. 20 μl of 6-phosphogluconate was added to start the reaction.
2.5.5 Phosphoglucose isomerase
The 1 ml reaction system contained 925 μl Tris–HCl buffer (100 mM Tris–HCl, pH = 7.5), 10 μl MgCl2 (10 mM), 5 μl NADP (0.5 mM), 1 U glucose-6-phosphate dehydrogenase and 50 μl cell extract or permeabilized cell suspension. 10 μl of fructose-6-phosphate was added to start the reaction.
2.5.6 Enzyme activity unit
One unit of enzyme is defined as the amount of enzyme producing 1 μmol NADPH per min under the assay conditions. Enzymatic activities are given per mg of dry cells unless specially defined. All absorbance measurements were carried out in a spectrophotometer at controlled temperature.
2.5.7 Cell density in enzyme assay
Permeabilized cell suspension was diluted 20 times starting usually at 10 mg/ml and yielding 0.5 mg/ml in the reaction cuvette.
3 Results
3.1 NAD(P)H measurement in permeabilized cells
In optically clear solution the measured absorbance is usually linear with concentration following Lambert Beer's law. During activity measurement with permeabilized cells, the absorption of NAD(P)H is measured in a cell suspension disturbing its measurement. It was therefore essential to study whether NAD(P)H could be measured in a permeabilized cell suspension. Various amounts of NADPH were mixed with permeabilized cell suspensions to give final concentrations from 0 to 50 μg/ml. Plots of the absorbance at 340 nm, A340, against NADPH concentration are depicted in Fig. 1. The range of linear correlation decreased with increasing cell concentration (Table 1). The corresponding R2-values are listed in Table 1. A good linear relationship was obtained for a range of NADPH concentration from 1 μg/ml to 20 μg/ml for cell densities below 1 mg/ml (CDW). The highest A340 value in this linear range was 2.77. For cell densities between 1 to 6 mg/ml, the corresponding A340 values were linear at NADPH concentrations from 1 μg/ml to only 10 μg/ml with a highest A340 value of 3.11. A340 values below 2.77 increased linearly with rising NADPH concentration at cell densities below 6 mg/ml. For cell densities higher than 6 mg/ml A340 values were still linear although only in a small range from 1 to 10 μg/ml. It was concluded that cell densities above 6 mg/ml are not suitable for enzyme activity measurements.
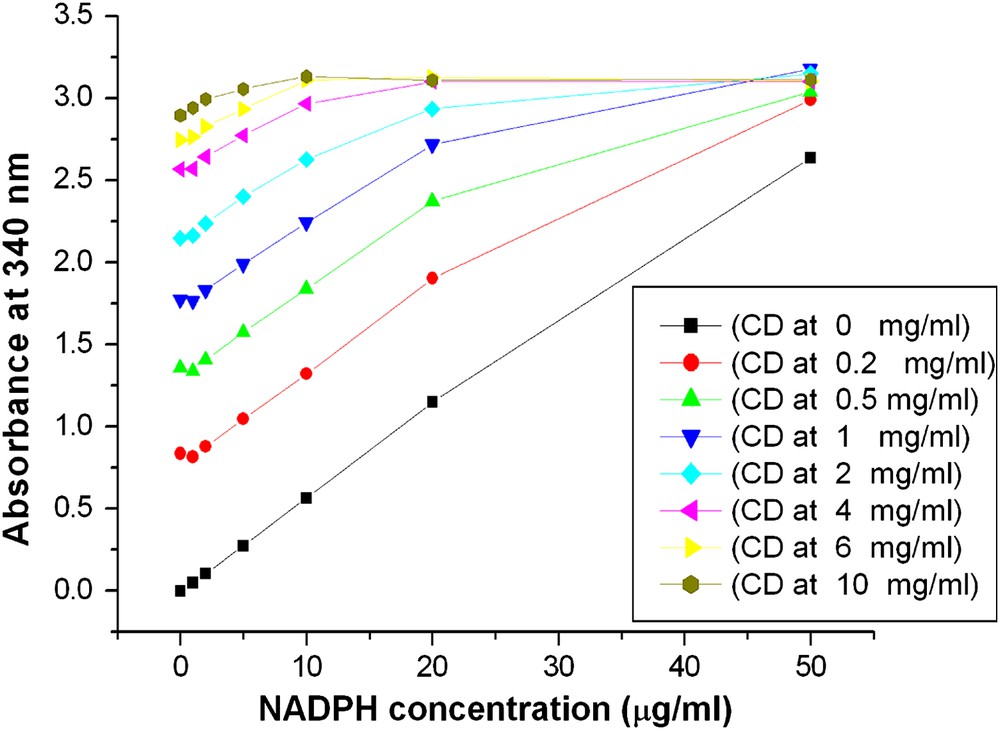
Absorbance and concentration of NADPH at various permeabilized cell densities. Harvested cells were washed 3 times with Tris–HCl buffer (pH = 7.8) and centrifuged. Afterwards the suspension was diluted with the same buffer to desired cell densities, followed by permeabilization with Triton X-100. After permeabilization, cell suspensions were diluted 20 times (the same ratio as in enzyme assay) with buffer to final cell densities (CD) of 0 mg/ml, 0.2 mg/ml 0.5 mg/ml, 1 mg/ml, 2 mg/ml, 4 mg/ml, 6 mg/ml, 10 mg/ml. For each cell density, 8 ml diluted cell suspension was divided evenly into 8 cuvettes and added various amount of NADPH to final concentrations of 0 μg/ml, 1 μg/ml, 2 μg/ml, 5 μg/ml, 10 μg/ml, 20 μg/ml, 50 μg/ml. Absorbance was measured with a spectrophotometer at 340 nm.
Linear dependency of the absorbance at 340 nm, A340, on the concentration of NADPH, CNADPH, at various cell densities as depicted in Fig. 1.
Cell density (mg/ml) | Slope, SL (ml/μg NADPH) | Intercept, IC | R2 | CNADPH, max μg/ml |
0 | 0.053 | 0.0162 | 0.9986 | 20 |
0.2 | 0.0563 | 0.759 | 0.9998 | 20 |
0.5 | 0.054 | 1.2949 | 0.9996 | 20 |
1 | 0.0524 | 1.7216 | 0.9989 | 10 |
2 | 0.0506 | 2.1304 | 0.995 | 10 |
4 | 0.0427 | 2.5464 | 0.9917 | 10 |
6 | 0.0371 | 2.7413 | 0.9935 | 10 |
10 | 0.0171 | 2.9634 | 0.9926 | 10 |
Slopes, SL, and intercepts, IC, of the linear parts of the calibration curves depicted in Fig. 1 and listed in Table 1 depended on cell densities, XCD, in a regular way as is shown in Fig. 2. This is described by the equation
SL = −0.0036 XCD + 0.0568 | (1) |
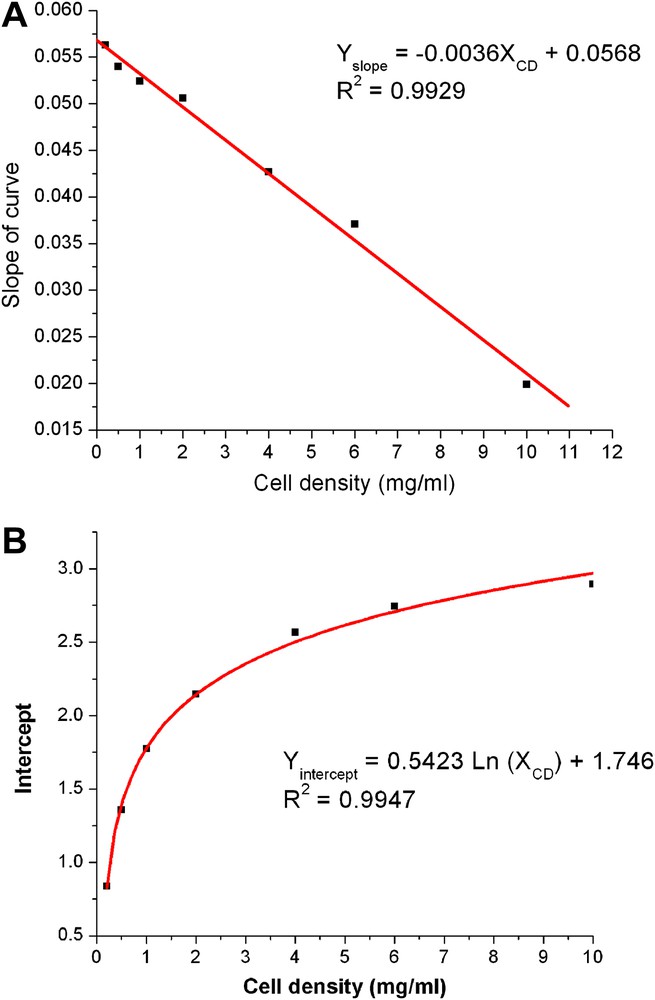
Slopes (A) and intercepts (B) of Table 1 and cell density.
The intercept depended linearly on the logarithm of cell density as given by
IC = 0.5423 ln (XCD) + 1.746 | (2) |
From the above relationships and equations it is possible to determine the NAD(P)H concentration in a cell suspension just by measurement of two different absorptions, A660 and A340, respectively. The measurement of A660 that is linearly correlated with cell density directly yields slope and intercept using Eqs. (1) and (2). Similar to the Lambert-Beer's law, a linear correlation exists between the absorbance at 340 nm, A340, and NADPH concentration, CNADPH
A340 = SL CNADPH + IC | (3) |
Rearrangement of this equation yields
(4) |
3.2 Permeabilization optimization
3.2.1 Selection of agents for permeabilization
Table 2 summarizes effects of treatment using different permeabilization agents on four intracellular enzyme activities: glucose 6-phosphate dehydrogenase, G6PDH, malic enzyme, ME, isocitrate dehydrogenase, IDH, and 6-phospho-gluconate dehydrogenase, 6-PGDH. Untreated cells (control) did not show any activity in contrast to treated cells which show significant variation on different treatments. Except for G6PDH, higher activity was observed in cell-free extracts than in permeabilized cells. For G6PDH and 6GPDH the measured activities were very similar for cell-free extract, Triton X-100 and TE. The activities for ME and particularly IDH were significantly higher in cell-free extracts than in permeabilized cells. CTAB treatment was comparable to other permeabilization methods for ME and 6PGDH. In this study, Triton X-100 and TE were investigated in more detail.
Activity of selected intracellular enzymes of C. glutamicum using enzyme extraction and different methods of permeabilization. All activities are given in mU/mg dry cells.
Enzyme | Control untreated cells | Cell-free extracta | TritonX-100 permeabilized cellsb,g | TE permeabilized cellsc,g | CTAB permeabilized cellsd,g | Digitonin permeabilized cellse,g | Ethanol permeabilized cellsf,g |
Malic enzyme | 0 | 51.1 ± 5.7 | 20.5 ± 1.9 | 41.7 ± 3.6 | 23.7 ± 4.7 | 16.1 ± 3.6 | 1.0 ± 0.3 |
G6PDH | 0 | 22.6 ± 1.5 | 24.1 ± 2.0 | 22.0 ± 2.9 | 5.5 ± 1.8 | 12.1 ± 3.1 | 8.8 ± 0.9 |
IDH | 0 | 304 ± 30 | 109 ± 13 | 96.6 ± 12.4 | - | - | - |
6PGDH | 0 | 36.4 ± 4.4 | 26.5 ± 4.4 | 25.6 ± 5.1 | 24.4 ± 2.4 | - | - |
a Glass-bead treatment followed by direct analysis of enzyme activity in cell-free extract.
b Triton X-100 was added to yield a final concentration of 0.05% and kept at room temperature for 30 min.
c TE was added to a final concentration of 10% and kept at room temperature for 30 min.
d CTAB was added to a final concentration of 0.1% and kept at room temperature for 30 min
e Digitonin was added to a final concentration of 0.1% and kept at room temperature for 30 min.
f Ethanol was added to a final concentration of 40% and kept at room temperature for 30 min.
g In all cases of permeabilization enzyme activity was directly measured in the resulting cell suspension.
3.2.2 Effect of cell density on permeabilization
Washed cells were diluted to final cell densities between 2 mg/ml and 20 mg/ml (CDW). Fig. 3A shows that malic enzyme and G6PDH activities increased linearly in Triton X-100 permeabilized cells with cell densities up to 10 mg/ml. The same trend was observed with ME activity in TE permeabilized cells as depicted in Fig. 3B. It was reported that permeabilization depends primarily on the ratio of the permeabilization agent to cell number rather than its concentration [10]. This means that at higher cell density a higher agent concentration is required which may cause problems in following analysis steps. On the other hand, a relatively high protein concentration and therefore high cell concentration is required for a sensitive activity measurement. 10 mg/ml was identified as the optimal cell density for permeabilization in this study.
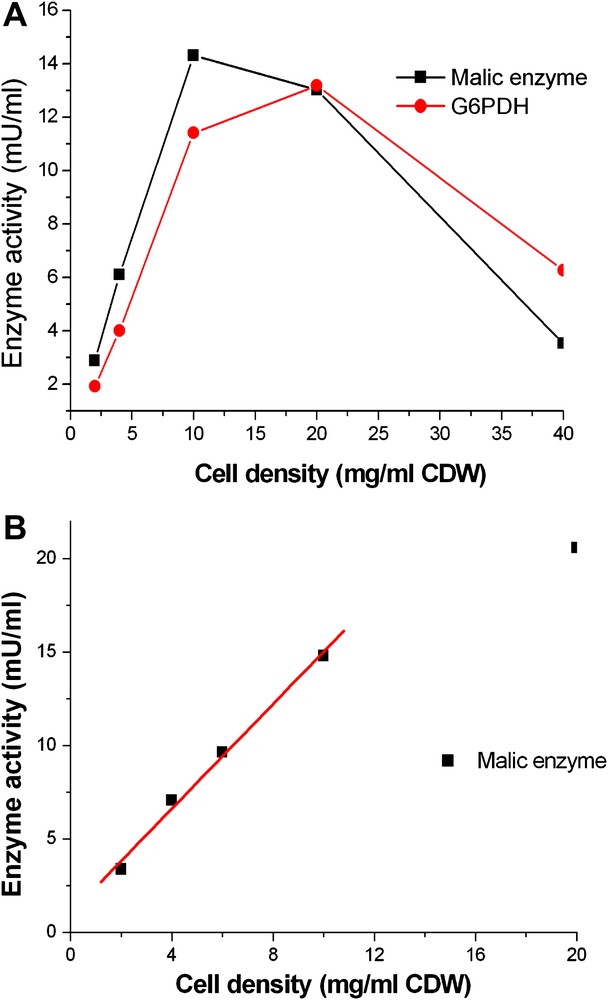
Influence of cell density on permeabilization in C. glutamicum. Enzyme activities were defined as mU per 1 ml reaction system in a cuvette. A: Washed cells were resuspended in Tris–HCl buffer at various densities from 2 to 40 mg/ml (CDW). Triton X-100 was added to each cell density and adjusted to the same final concentration of 0.05% and then incubated at room temperature for 30 min. B: Washed cells were resuspended in Tris–HCl buffer at different densities from 2 to 20 mg/ml (CDW). TE was added to each cell density and adjusted to the same final concentration of 10% and then incubated at room temperature for 30 min.
3.2.3 Effect of agent concentration for permeabilization
Fig. 4A shows the effect of Triton X-100 concentration on enzyme activity. The level of both enzyme activities of permeabilized cells increased significantly but not linearly with the Triton X-100 concentration from 0.02% to 0.05%. Higher Triton X-100 concentration did not result in any further increase for both enzymes. In contrast relatively high TE concentration was necessary for permeabilization to get highest enzyme activities (Fig. 4B). For malic enzyme activity a concentration higher than 20% was required for the highest activity, but for G6PDH, the highest activity was found at a TE concentration of 10% and the activity decreased at higher concentrations than 10%.
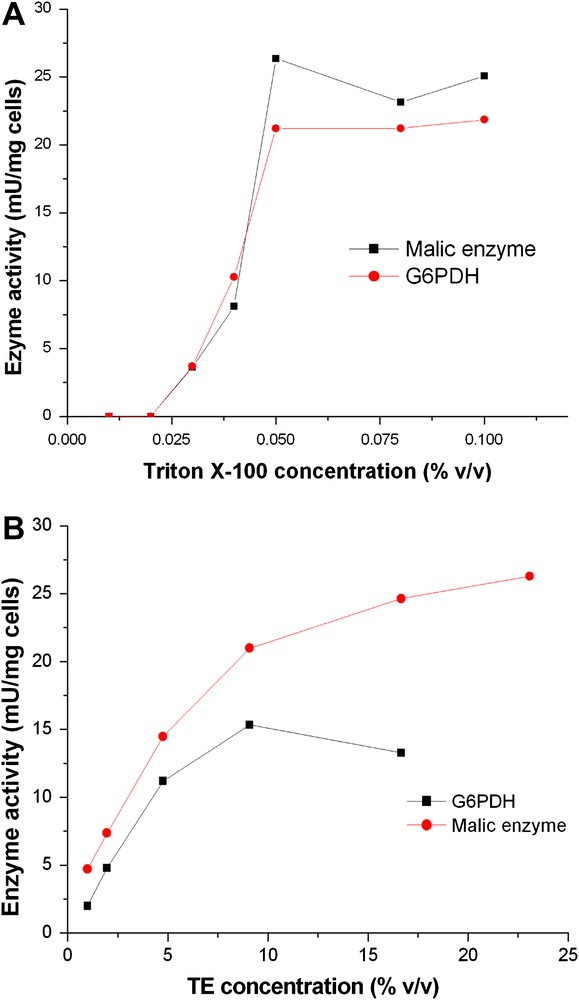
Influence of agent concentration on permeabilization in C. glutamicum. Washed cells were resuspended in Tris–HCl buffer at a density of 10 mg/ml (CDW). A: Triton X-100 was added to yield different concentrations from 0.01% to 0.2%. B: Triton TE was added to give different concentrations from 1% to 23%.The suspension was incubated at room temperature for 30 min and assayed for enzyme activity.
3.2.4 Effect of incubation condition on permeabilization
Several different conditions suggested in the literature were tested and results are shown in Fig. 5. No significant differences were found under the conditions tested with Triton X-100 permeabilized cells, i.e. freeze/thaw cycles with −70 °C, −20 °C, dry ice bath and incubation at room temperature. Continuous shaking during the incubation at room temperature also did not affect enzyme activities. Room temperature incubation without shaking was found most appropriate and was further studied. For Triton X-100 treated cells, the effect of varying incubation time at room temperature was investigated (Fig. 6A). The activities of ME and G6PDH both reached maximum values after an incubation of 5 min and decreased slightly with increasing time. For TE treated permeabilized cells (Fig. 6B), a longer time was necessary for both enzymes. After 40 min incubation, ME reached its top activity but G6PDH activity remained constant after 40 min.
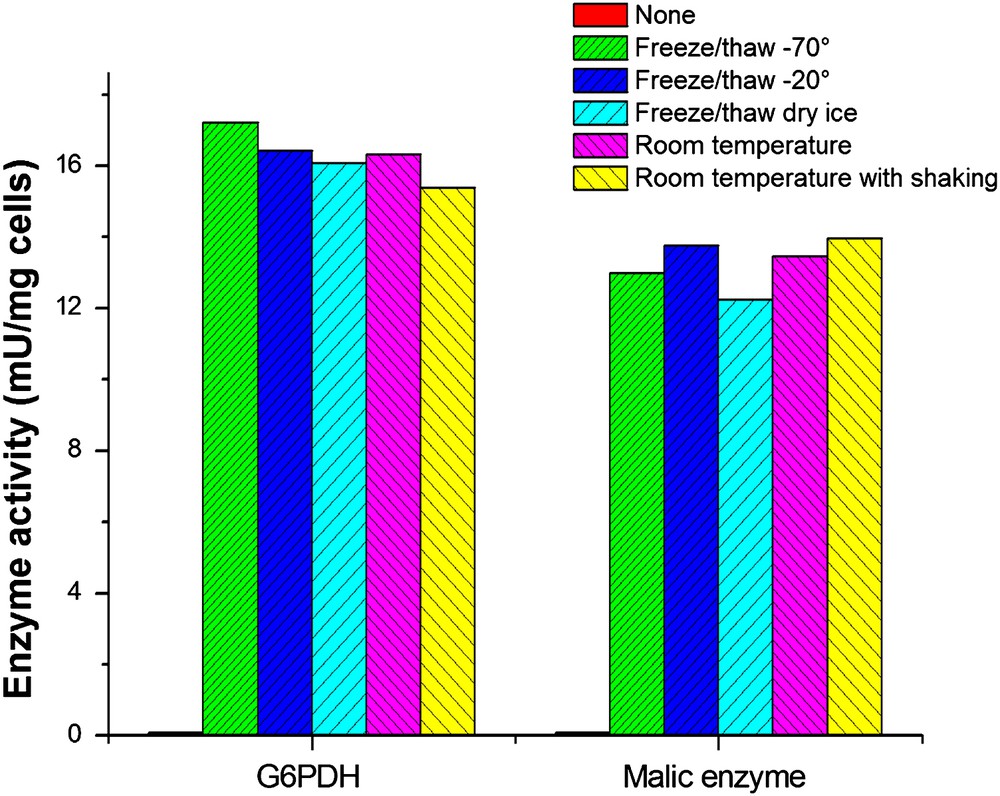
Enzyme activity after permeabilization under different incubation conditions. C. glutamicum cells were harvested during the exponential growth phase and washed twice with Tris–HCl buffer. The pellet was resuspended in the same buffer to 10 mg/ml (CDW) and divided into several portions, subjected to further Triton X-100 treatments as indicated below. Freeze/thaw at -70 °C: Cells were frozen in -70 °C freezer for 24 h and thawed by swirling in a water bath at 30 °C. Freeze/thaw at −20 °C: Cells were frozen in −20 °C freezer for 24 h and thawed by swirling in a water bath at 30 °C. Freeze/thaw in dry ice bath: Cells were frozen in dry ice bath for 2 h and thawed by swirling in a water bath at 30 °C. Room temperature: Triton X-100 was added to a final concentration of 0.05% and kept at room temperature for 30 min. Room temperature with shaking: Triton X-100 was added to a final concentration of 0.05% and the cell suspension was kept shaking at a speed of 500 rpm for 30 min.

Influence of incubation time on permeabilization of C. glutamicum. Washed cells were resuspended in Tris–HCl buffer at a density of 10 mg/ml (CDW). A: Triton X-100 was added to a final concentration of 0.5%. The suspension was incubated at room temperature for various times from 0 to 170 min and assayed for enzyme activity. B: TE was added to a final concentration of 10%. The suspension was stored at room temperature for various times from 0 to 80 min and assayed for enzyme activity.
3.2.5 Enzyme-substrate affinity in permeabilized cells
The substrate affinity to an enzyme, usually characterized by the Michaelis-Menten kinetic parameter, Km, is a key characteristic of an enzyme. In this study, Km values of several intracellular enzymes from both the cell-free extract and permeabilized cells were determined and compared with literature values for purified enzymes (Table 3). Km values of all four enzymes were similar in cell-free extracts and permeabilized cells [21,31,32]
Km values of enzymes from cell-free extract, permeabilized cells in C. glutamicum and literature values of purified enzymes.
Km value (mM) | Malic enzyme | G6PDH | PGI | |
For Malate | For NADP+ | For G-6-P | For F-6-P | |
Cell-free extract | 13.9 ± 5.2 | 0.17 ± 0.12 | 0.14 ± 0.0005 | 0.34 ± 0.15 |
Permeabilized cells | 10.7 ± 4.0 | 0.23 ± 0.03 | 0.092 ± 0.017 | 0.42 ± 0.12 |
Data from the literature | 3.8 ± 0.6a | 0.083 ± 0.017a | 0.15 ± 0.021b | 0.54c |
3.3 Protein release
After washing permeabilized cells, all the supernatants were collected and analyzed for protein secretion. Protein concentration was determined by the Bradford method, bovine serum albumin was used as the standard. Analyzed protein levels in the supernatant were always below 0.2 mg/ml, i.e. less than 5% of the total protein (data not shown here).
3.4 Activity analysis in fermentation broth without washing step
The developed method was also applied directly to the fermentation broth without any washing. A cell suspension from a growing culture was taken, directly treated by Triton X-100 and used for enzyme activity measurements. In these experiments linear relationships were also obtained between absorbance at 340 nm and NADPH concentration, but the coefficient in Eq. (1) changed from −0.0036 to −0.0202. Enzyme activities under this condition were only about half of those obtained with a washing step (data not shown here).
4 Discussion
There is a ongoing discussion whether in vitro enzyme characteristics can be directly applied for describing the dynamics of complex metabolic networks [33]. It is very difficult and sometimes even not possible to directly measure intracellular enzyme activity and kinetic characteristics in intact whole cells due to the cell membrane permeability barrier. Only pulse techniques combined with appropriate measurement of intracellular metabolite profiles and modelling permits the in vivo kinetic analysis [33,34]. For metabolic enzyme kinetic research, traditional studies using purified enzymes or cell-free extracts, which are called in vitro conditions, is restricted because many unknown interactions, e.g. between enzymes and other effecting proteins, may influence the final results that will then be different from in vivo conditions [33]. In addition, the preparation procedure is generally complex and time consuming. Enzymes in gently permeabilized cells are assumed to be much closer to their in vivo condition and therefore more suitable to be applied in metabolic studies. In this article, permeabilization of C. glutamicum was investigated for potential applications for metabolic enzyme kinetic study.
The influence of cell density on the absorbance at 340 nm for NADPH determination was investigated in detail in this study, which is very important for NAD(P)H-dependent enzyme activity measurement. Absorbance values of the enzymatic reaction system containing permeabilized cells cannot be directly used for activity calculation only after appropriate correction. Since NADH has an absorption spectrum very similar to NADPH, the results of this report are directly applicable to NADH dependant enzymes.
The results using a crude fermentation broth indicate the possibility of high-throughput screening of mutants using the permeabilization method in microtiter plates. After pipetting into wells, mixing with the permeabilization agent and corresponding enzyme substrates and incubating for an appropriate time, absorbances at 660 nm and 340 nm could be read with a microplate reader. After correction of absorbance using Eq. (4), activities can be easily calculated and compared. In this way a large number of mutants could be screened in a short time. Besides C. glutamicum, this method could be also applied to other organisms.
Many agents have been applied for permeabilization in various organisms. The efficiency of different agents on permeabilization are different (Table 2). Triton X-100 and TE were most suitable for C. glutamicum for enzyme activity measurement. Compared to TE, treatment with Triton X-100 needs a much lower concentration and shorter incubation time. If other analytical methods, e.g. using mass spectrometry with ion spray ionization or MALDI, are applied, it might be necessary to remove the permeabilizing agent. Since Triton X-100 is a detergent, it is very difficult to remove. However, TE could be removed easily by evaporation.
The environment of enzymes in permeabilized cells (in situ) is thought to be closer to that in living cells (in vivo) compared to cell-free extract (in vitro). Therefore, the kinetics of enzymes in situ is assumed be closer to in vivo conditions than in vitro kinetics. The Km value is regarded as a key parameter for kinetic studies, and values from in situ condition are assumed to be closer to those in vivo condition. In our experiments Km values of enzymes measured in permeabilized cells were similar to those from the cell-free extract, whereas other reports reported differences, however using different cells [15,35]. In this study, Km values of both malic enzyme and G6PDH were clearly higher than those of purified enzymes reported in the literature [31,32]. Only for PGI were the Km values measured here slightly lower than those reported in the literature [21].
5 Conclusion
NAD(P)H-dependent intracellular enzyme assays in permeabilized cell suspension were investigated in this study. Permeabilization of C. glutamicum cells depends on several variables such as agent type, cell density, agent concentration but less on the incubation condition. The optimized permeabilization condition in C. glutamicum by Triton X-100 was obtained with CDW concentration of 10 mg/ml and treatment with 0.05% Triton X-100 at room temperature for only 5 min. TE treatment needed a concentration of 10% and a much longer incubation time of more than 40 min to get the highest enzyme activity. Compared to the cell-free extract preparation, permeabilization is a rapid, simple and mild technique. Using this method single enzyme activity measurements for the dehydrogenases G6PDH, 6PGDH and ME as well as for PGI were carried out successfully. Furthermore, permeabilized cells can provide intracellular enzymes whose properties are assumed to be more similar to those of in-vivo conditions
Acknowledgement
We acknowledge financial support from the German Research Foundation DFG under the project, ‘European Postgraduate School of Physical Methods for the Structural Investigation of New Materials (GRK-532)’.
Conflict of interest
The authors declare that there is no conflict of interest.