1 Introduction
Wine organoleptic qualities are the result of a perfect equilibrium between four groups of chemical components determining the four wine elementary flavors: sugars giving smooth, acids, salts and tannins determining astringency and/or bitterness. The majority tannins present in red wine are proanthocyanidins or oligomers of flavan-3-ols (OPCs). Two elements account for their gustative role: their concentration which depends on the variety of vine, vintage, soil, climate, wine-making procedure [1]… and their structure. Indeed, with two different structural units (epicatechin EC and catechin C), two possible links (4–6 and 4–8) and the possible galloylation of the hydroxyl group positioned on C3, they represent a disperse family (Scheme 1) in which each structure is neither completely different nor really the same, and has been already shown to exhibit specific 3D-structures [2,3]. For instance, many different procyanidin species have been identified in red wine, these species being, or not, galloylated, with a polymerization degree varying from 1 to 18. However, beyond their important gustative role, they act as structuring agents and are thought to be involved in red wine turbidity and stability. This second role is the consequence of their colloidal behavior. In order to contribute to a better comprehensive knowledge of the roles that tannins are supposed to play in wine, we choose to study the colloidal behavior of the simplest model, that is to say of synthesized procyanidins galloylated or not. Thus, in a previous work [4], the colloidal behavior of eight procyanidins (three monomers, four dimers and one trimer) was investigated; this study allowed us to clarify the role of procyanidin monomers versus oligomers in the turbidity of red wines: monomers are shown to form bigger micelles at lower concentration than oligomers. This particular behavior has been related to their 3D-structures and their hydration potential: the relative planar structure of catechin or epicatechin and their low hydration level allow them to form stacks, micelles or even insoluble aggregates above a relatively low concentration (< 9 mM). On the contrary, procyanidin dimers and trimers exhibit a higher hydration potential, and are able to form smaller micelles to be involved in wine turbidity. In the present work, we continue our investigations on galloylated tannins which exhibit a particular 3D-structure as regard to their non-galloylated form [2].
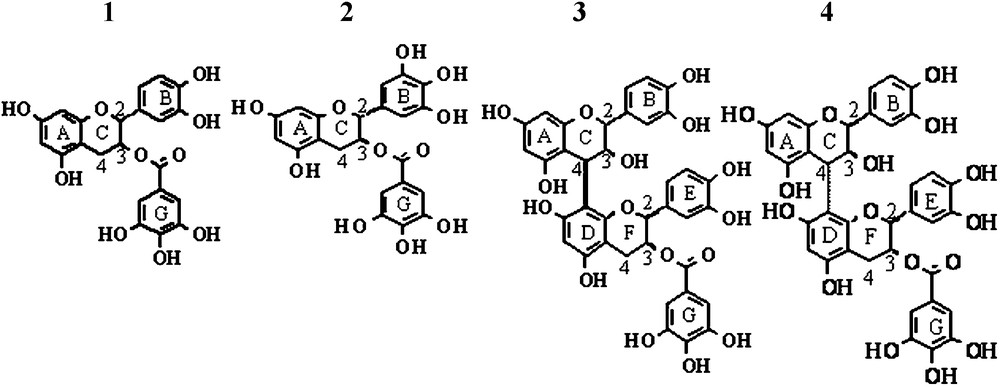
Chemical structure of the studied tannins. 1. Epicatechin Gallate (ECG). 2. Epigallocatechin gallate (EGCG). 3. B2 gallate (B2G). 4. B4 gallate (B4G).
2 Experimental
2.1 Materials
Epigalocatechin gallate (EGCG, 2), catechin (C) and epicatechin (EC) were purchased from Sigma. The tetrabenzylated EC [5] was galloylated and after deprotection, ECG (1) was obtained with a yield of 75%. The tetrabenzylated EC was also used as a precursor to synthesize B2 gallate (B2G) and B4 gallate (B4G) using the strategy described for B2 and B4 syntheses [5]. After deprotection, B2G (3) and B4G (4) galloylated procyanidins were obtained with a yield of approximately 50% (Scheme 1).
2.2 Methods
NMR experiments were performed at 298 K on a Bruker DPX 400 equipped with a 5 mm gradient inverse broadband probe. The self-association of (1), (2), (3) and (4) was monitored by following both 1H chemical shift variations (δH) and diffusion coefficient (D) with respect to tannins concentration (between 0.5 to 20 g/L in a D2O mixture, pH 7.0). Single pulse experiments were used to record 1H 1D spectra and D measurements were obtained as previously described [6] using the Stejskal and Tanner also called PGSE pulse sequence [7]. The following parameters were used: spectral width, 10 ppm; number of acquisitions, 64; recycling delay, 3 s; intergradient delay Δ, 250 ms; gradient pulse duration δ, 2.5 ms; 16 different G values varying from 0.01 to 0.4 G/m. For each concentration, different D values were collected as follow: two different zones corresponding to specific galloylated procyanidins resonances were integrated (one corresponding to the catechol and the galloyl ring protons, around 7 ppm and one corresponding to proton resonances from the heterocyclic ring, and particularly proton H3 for ECG or H3F for B2G and B4G, resonating at a lower field than water, around 5.3 ppm) for each G value. Their decrease was least squares fitted using the following relation:
(1) |
D and δH NMR data were analysed to obtain both self-association constant Ka and CMC value (Critical Micellar Concentration, that is to say, the concentration above which tannins preferentially exist upon a micelle or an aggregate form), these two physicochemical parameters describing the “micellization” process (Scheme 2). CMC is obtained by plotting D against the inverse concentration, two slopes are obtained and the CMC value is estimated at the interception of these two lines. The self-association constant Ka is obtained by following the evolution of either δH or D versus tannin concentration. D or δH changes were least squares fitted to the following equation representative of an indefinite non-cooperative way to form micelles [4,8]:
(2) |
(3) |
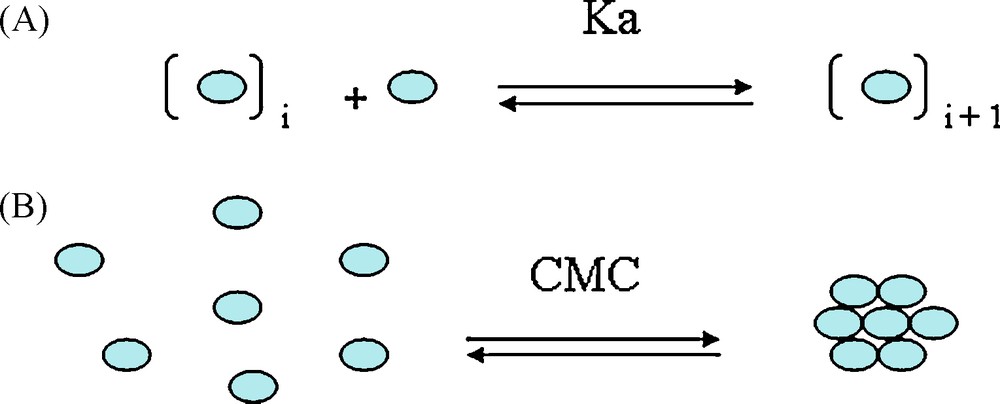
Tannin colloidal behavior – from ref. [1]. A. Self-association constant Ka according to an indefinite non-cooperative model. B. Critical Micelle Concentration (CMC), above which micelles are formed.
3 Results and discussion
3.1 Self-association of galloylated tannins
Self-association of galloylated tannins was studied in D2O for simplicity and because we previously demonstrated [4] that if the presence of 10% ethanol increases the CMC values of non-galloylated procyanidins and decreases the formed micelles size by a factor 2, the presence of salt (20 mM NaCl) appears to negate the effect of ethanol.
Fig. 1A shows chemical shift changes observed with respect to B2G concentration (proton H4). As for procyanidin dimers, δH from the heterocyclic ring protons (H2, H3 and H4) are the most responsive protons to concentration variations. Fitting these variations using Eq. (1) provides a measurement of Ka with a quite good accuracy showing that the model used well described the association process (Scheme 2).
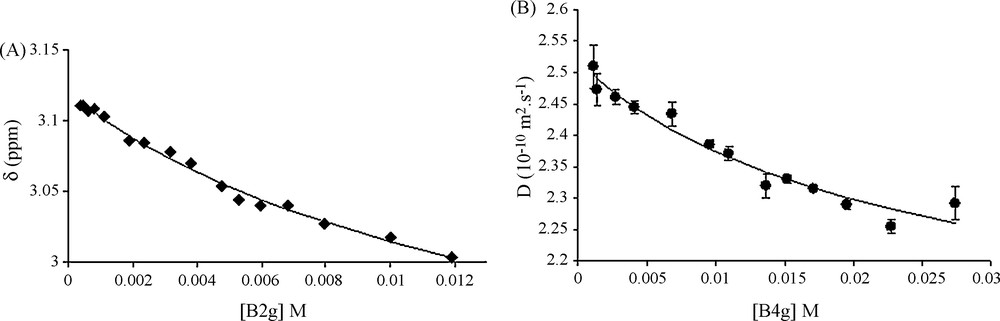
A. Chemical shift variations of the 4F proton (δobs, ppm) vs B2G concentration (M). Solid line represents the best fit obtained using Eq. (1) with Ka = M−1, (Δmax = 0.42; δfree = 3.12 ppm and χ2 = 1.6 × 10−4. B. Variation of D vs B4G concentration (M). Solid line represents the best fit obtained using Eq. (1) with Ka = 31 M−1, Dfree = 2.52 × 10−10 m2 s−1, ΔD max = 0.744 and χ2 = 3.7 × 10−3.
D were calculated from DOSY experiments as explained in the experimental part and as shown in Fig. 2. Fig. 2A shows the intensity decrease with the gradient field increase. Fig. 2B displays the variation of the ln of the intensity of the catechol resonance of ECG (27.2 mM, 12 g/L) and B4G (27.4 mM, 20 g/L) against the square gradient force field. Two straight lines with different slopes proportional to D are obtained. Using this procedure, D values were collected for the three galloylated tannins and at different concentration between 0.5 and 20 g/L. D variation against the concentration can also be fitted with the same equation, confirming the estimation of Ka value, as shown in Fig. 1B for B4G. Though, Ka values were obtained from different fits (protons H2, H3 and H4 chemical shift and Diffusion variations) of every tannin. The different Ka obtained for each tannin were averaged and are reported in Table 1. These values, expressed in M−1, show slight differences whatever the galloylated tannins considered: all Ka values are close to 40 M−1, values in the same range than the non-galloylated monomers catechin or epicatechin. This behaviour differs from the one of non-galloylated procyanidins for which the association constant reaches 6 M−1 for dimers or trimers [4].
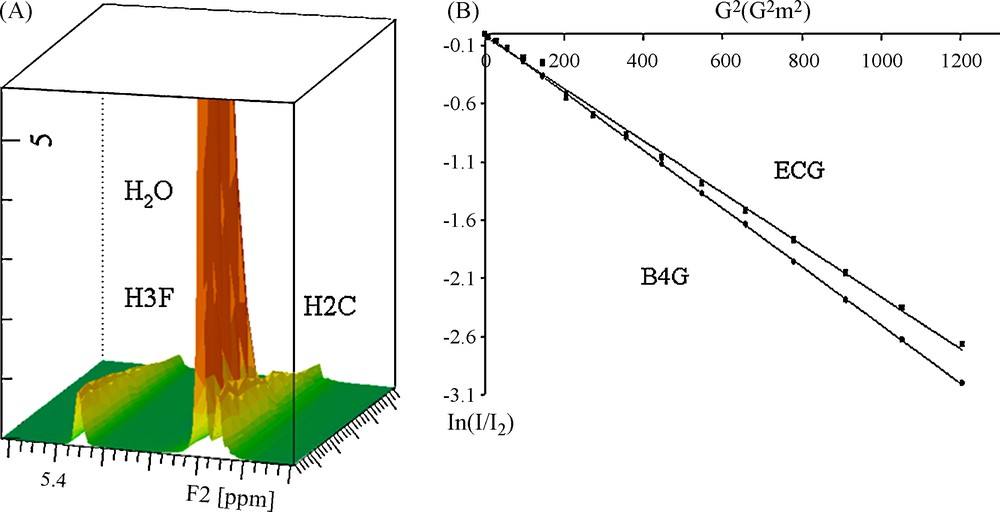
A. 3D representation of the DOSY spectrum in which the H3F and H2C resonances of B4G are observed as well as the HDO resonance. B. Measurement of the D value from a linear representation.
Different parameters obtained from D/δsH measurement at different tannin concentration, by fitting the experimental data points using Eq. (1) (Ka, Dfree, Dmicelle) or Eq. (2) (rH free, rH micelle) or by displaying D against the inverse tannin concentration; which gives two slopes intercepting at the CMC value.
Tannins | ECG/ECa | EGCGa | B2G /B2a | B4G/B4a |
Ka (M−1) | 42 ± 6/39 ± 8 | 44 ± 5 | 40 ± 8/7 ± 1 | 34 ± 4/7 ± 2 |
Dfree(10−10 m2 s−1) | 2.71 | 2.70 | 2.69 | 2.53 |
rH free (Å) | 7.36 | 7.39 | 7.41 | 7.91 |
Dmicelle (10−10 m2 s−1) | 1.0 | 1.0 | 1.2/0.9 | 1.4/0.9 |
rHmicelle (Å) | 19.9 | 19.9 | 16.6/22.1 | 14.2/22.1 |
CMC (mM) | 2.8 ± 0.3/< 5 | 6.0 ± 2.0 | 4.2 ± 1.4/18 ± 3 | 5.6 ± 0.7/17 ± 4 |
a Values from ref. [4].
3.2 Micelles size assessment
Fig. 1B clearly shows that diffusion coefficients decrease with increasing tannin concentration, suggesting that they get organized in micelles. At lower concentrations, D value reaches the value of the tannin in its free form (Dfree) whereas, at higher concentration, D value reflects the value of the tannin embedded in the micelle. This value evolves till a plateau to reach a minimum D value called herein Dmicelle. This Dmicelle value is obtained from the fitting of D vs tannins concentration using Eq. (1) and is used as a probe to evaluate the average micelles size thanks to Eq. (2). As shown in Table 1, Dmicelle reaches the Å2 s−1range, that corresponds to micelles radii up to 20 Å as for non-galloylated tannins.
3.3 CMC measurements
Fig. 2A displays the D values evolution with respect to the inverse of galloylated tannins concentration. As expected, it exhibits two slopes at the interception of which the CMC value can be collected. The same kind of profiles can be obtained by displaying the chemical shift evolution against the inverse of tannins concentration (Fig. 2B). CMC values were estimated from all these graphs and values obtained were averaged and are reported in Table 1. It is noteworthy that CMC values obtained for these tannins are significantly lower than values exhibited by their non-galloylated counterpart CMC (Fig. 3).
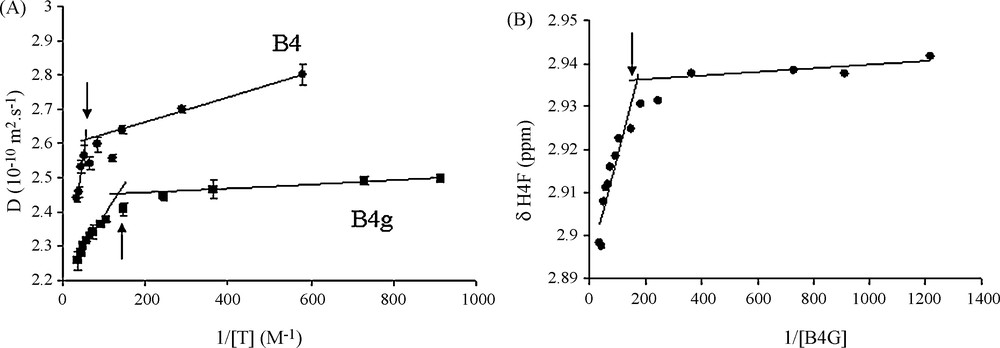
Determination of the CMC's from diffusion coefficient D. A. for B4G and B4 or chemical shift δH for H4F of B4G. B. The intercept of the two slopes obtained for concentrated (colloidal state) and diluted (soluble state) regions of D or δH against inverse tannin concentration gives the CMC (arrows).
4 Discussion
Concerning the self-association processes, the fact that the isodesmic model used provides good fittings whatever the monitored parameter (chemical shifts or D values), demonstrates that self-association of galloylated procyanidins follows the same law than non-galloylated procyanidins. In this model, the association constant is the same at each step of the association process, contrarily to surfactant behavior, for example [9]. Such a model leads to the formation of micelles of polydisperse size. Galloylated procyanidins stay in a free state until a concentration around 3 to 5 mM, and preferentially self-associate above this concentration with an equilibrium constant close to 40 M−1. The lower CMC and higher Ka values obtained for galloylated tannins with respect to their non-galloylated counterpart show that galloylated tannins are more able to form micelles at OPCs wine content (∼3 mM found in wine coming from the Southwest of France [10]) than non-galloylated forms. Differences between the behavior of galloylated vs non-galloylated procyanidins should be attributed to differences in their internal dynamic. As previously described [2], galloylated B2G has been shown to exist upon one rotameric compact form in which stacking occurs between the galloyl moiety and the upper epicatechin unit while its non-galloylated counterpart exists upon two rotameric forms in a 40:60 ratio. Such a result let us suggest that galloylated tannins thanks to their propensity to self-associate should play a structuring role in wine. However, it is noteworthy that the radius of the formed micelles did never exceed the nm whatever the tannin considered: this result suggests that micelles are composed of almost seven galloylated procyanidins and confirms that OPCs cannot be involved in wine turbidity alone [4,11].
Conflict of interest
No conflict of interest exists for all the co-authors.
Acknowledgements
This work has been supported by the Conseil Interprofessionnel de Bordeaux (CIVB), the CNRS, the University of Bordeaux 1.