1 Introduction
Our research group has recently described pitting corrosion of Al and Al-Zn alloys induced by the aggressive attack of SCN- anions [1]. Recently, glycine (Gly) was successfully reported as a corrosion safe inhibitor for some metals and alloys [2–6]. In view of the inhibition characteristics of Gly, a new Gly derivative (GlyD), namely 2-(4-(dimethylamino)benzylamino)acetic acid hydrochloride was synthesized in our laboratory to effectively inhibit pitting corrosion of Al in KSCN solutions. Measurements were carried out with the use of cyclic polarization and potentiostatic and galvanostatic measurements. The obtained results were compared with results recorded for Gly. Some SEM examinations of the electrode surface in absence and presence of the inhibitor were also performed.
2 Experimental
The working electrode employed here was made of pure Al (99.98%) provided by the Egyptian Aluminium Company (EAC). The Al electrode was used in the as-received condition. The investigated electrode was cut as a cylindrical rod, welded with Cu-wire for electrical connection and mounted into glass tubes of appropriate diameter using Araldite to offer an active flat disc shaped surface of (0.50 cm2) geometric area for the working electrode, to contact the test solution. Prior to each experiment, the electrode surface was wet polished with silicon carbide from 220 to 500 grits, degreased with acetone and, finally, rinsed with distilled water.
The glycine derivative (GlyD) was synthesized in the laboratory according to the procedure described below:
Glycine (0.75 g, 10 mmol) and 4-(dimethylamino)benzaldehyde (1.49 g, 10 mmol) were dissolved in ethanol (20 ml) in the presence of KOH (0.56 gm, 10 mmol) and vigorously stirred at room temperature and refluxed for 2 h. A white precipitate was formed on cooling, filtered off, washed with cold ethanol, and dried in vacuo. The produced imine derivative was then added to a solution of 0.5 gm (14 mmol) of NaBH4 in ethanol and the reaction was allowed to stand for 4 h at room temperature. The resultant solution was acidified by concentrated HCl. A white precipitate of 2-(4-(dimethylamino)benzylamino)acetic acid.HCl (the newly synthesized glycine derivative; GlyD) was formed, filtered, and dried up. Yield 1.98 (81%); FT-IR (KBr): ν(cm−1): 3428, 2947, 2360, 1751, 1635, 1564, 1539, 1472, 1420, 1209, 1051, 908, 665, 607, and 516. 1H NMR (500 MHz, DMSO-d6) δ (ppm): 13.56 (s, 1H, OH), 6.79 (s, 1H, NH), 7.62 (d, J = 7.8, 2H, C-Hbenzene), 7.42 (d, J = 7.8, 2H, C-Hbenzene), 4.07 (s, 2H, -CH2−CO−), 3.49 (s, 2H, -CH2−NH−), and 2.95 (s, 6H, −CH3).
All experiments were carried out in 0.04 M KSCN solutions without and with various concentrations (10−5–5 × 10−3 M) of Gly or its derivative (GlyD). For each run, a freshly prepared solution as well as a cleaned set of electrodes was used. Each run was carried out in aerated stagnant solutions at 25 ± 1 °C, using water thermostat.
Cyclic polarization measurements were carried out by sweeping linearly the potential from the starting potential, −2000 mV (SCE), into the positive direction at a scan rate of 0.50 mV s−1 till the end potential, 2000 mV (SCE), and then reversed with the same scan rate till forming a well-defined hysteresis loop. The potentiostatic j/t transient measurements were carried out after a two-step procedure, namely: the working electrode was first held at the starting potential for 60 s to attain a reproducible electroreduced electrode surface. Then the electrode was held at constant anodic step potential (Es.a) where the anodic current was recorded with time. The stabilization period prior to collecting data was 12 h. The open circuit potential of the working electrode was measured as a function of time during this stabilization time. This time was quite sufficient to reach a quasi-stationary value for the open circuit potential.
Electrochemical experiments were performed in a 100 ml volume Pyrex glass cell using Pt wire and a saturated calomel electrode (SCE) as auxiliary and reference electrodes, respectively. The SCE was connected via a Luggin capillary, the tip of which was very close to the surface of the working electrode to minimize the IR drop. All potentials given in this paper are referred to this reference electrode. Electrochemical measurements were performed using An Autolab Potentiostat/Galvanostat (PGSTAT30) connected with a personal computer with GPES and FRA (ver. 4.9) software provided by Autolab. The stabilization period prior to collecting data was 12 h.
For surface morphology studies, some Al samples were exposed to pitting attack; the samples were immersed for 5.0 min in 0.04 M KSCN solutions without and with 10−3 M Gly or GlyD at 25 °C. Measurements were performed under potentiostatic regime at 1300 mV (> Epit recorded for Al in 0.04 M KSCN), and finally washed thoroughly and subjected to 20 min of ultrasonic cleaning in order to remove loosely adsorbed ions. The morphology of the electrode surface was then examined by SEM using an Analytical Scanning Electron Microscope JEOL JSM 6390 LA.
3 Results and discussion
Fig. 1 shows s typical cyclic voltammogram recorded for Al in 0.04 M KSCN solution at a scan rate of 0.5 mV s−1 at 25 °C. The obtained plot had the familiar form for Al showing a well-defined corrosion potential, Ecorr, followed by a passive region. It is obvious that the passive region extends up to a certain potential, designated as the critical potential (Ec). As the electrode potential exceeds Ec, the passive current (jpass) increases slightly with potential. Then at a certain potential, a sudden increase in jpass is observed without any sign for oxygen evolution, indicating passivity breakdown, initiation and propagation of pitting corrosion. When the sudden increase in jpass was observed after the passive region, the potential value acquires the name of pitting potential (Epit).

Typical cyclic polarization plot recorded for Al in 0.04 M KSCN solution at a scan rate of 0.50 mVs-1 at 25 oC.
At Epit, the aggressive SCN− anions displace the adsorbed passivating species at some locations and accelerate local anodic dissolution. This mechanism is accelerated when SCN− concentration increases [1]. Pitting growth occurs as a result of an increase in SCN− concentration resulting from its migration inside pits and hydrolysis of Al3+ ions, since the high acidity required for pitting corrosion site growth must be achieved by hydrolysis of Al3+ ions inside pits; see more details in our previous studies [1,7]. The reverse scan shows a wide hysteresis cycle, this being characteristic of passivity breakdown on the upward sweep and repassivation. Finally, a repassivation potential, Erp, was attained.
Fig. 2 presents cyclic polarization plots recorded for Al in 0.04 M KSCN solutions without and with 10−3 M Gly or GlyD at a scan rate of 0.5 mV s−1 at 25 oC. It is obvious that addition of both Gly and GlyD decreases the anodic and cathodic current densities, reduces the corrosion current density (jcorr), and shifts Ecorr to more negative values. These results reflect, at first sight, the ability of these organic compounds to inhibit uniform corrosion of Al in these solutions. This point will be presented in details in a further complementary paper. In the present work, special attention was focused on the influence of Gly and GlyD on the pitting corrosion phenomena, regarding pit initiation and growth, of Al in SCN− solutions
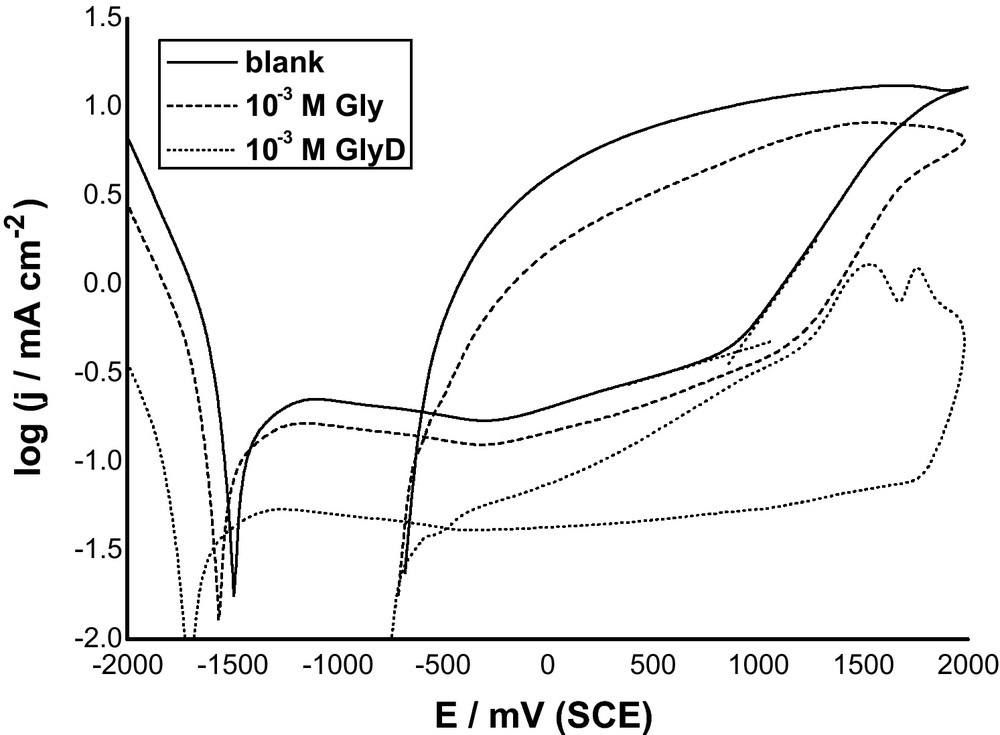
Cyclic polarization plots recorded for Al in 0.04 M KSCN solution without and with 10–3 M Gly or GlyD at a scan rate of 0.50 mVs-1 at 25 oC.
Referring again to Fig. 2, it is obvious that upon the addition of both Gly and GlyD, a decrease in the current in the passive region (jpass) results, with a positive shift in Epit. The negative shift in Ecorr and positive shift in Epit reflect resistance towards pitting, Rpit (Rpit = |Ecorr − Epit|). Electrochemical parameters derived from these measurements (not included here) showed that all these electrochemical events depend on the type of the introduced inhibitor, with GlyD being more effective than Gly. The positive shift of Epit suggests that pitting corrosion in presence of Gly and GlyD is unlikely to occur. This is most probably due to inhibitor adsorption and subsequent formation of a protective film on the electrode surface, see later.
Fig. 3 shows j/t transients recorded for Al in 0.04 M KSCN solutions without and with 10−3 M Gly or GlyD at an anodic potential of 1300 mV (>Epit) at 25 oC. In absence of inhibitor, curve 1, the anodic current density initially decreases, due to oxide film growth, to a minimum value determining the characteristic pitting parameter, namely the incubation time (ti). These data reveal that an incubation time is necessary before pit initiation. The magnitude of ti reflects the susceptibility of sample to pitting corrosion. Beyond ti, the current density increases rapidly and finally reaches a steady state value. The rise in the current density (i.e., the pit growth current density (jpit)) indicates pit growth with time, while the steady state is due perhaps to pits blocked by corrosion products that accumulated on the anode surface.
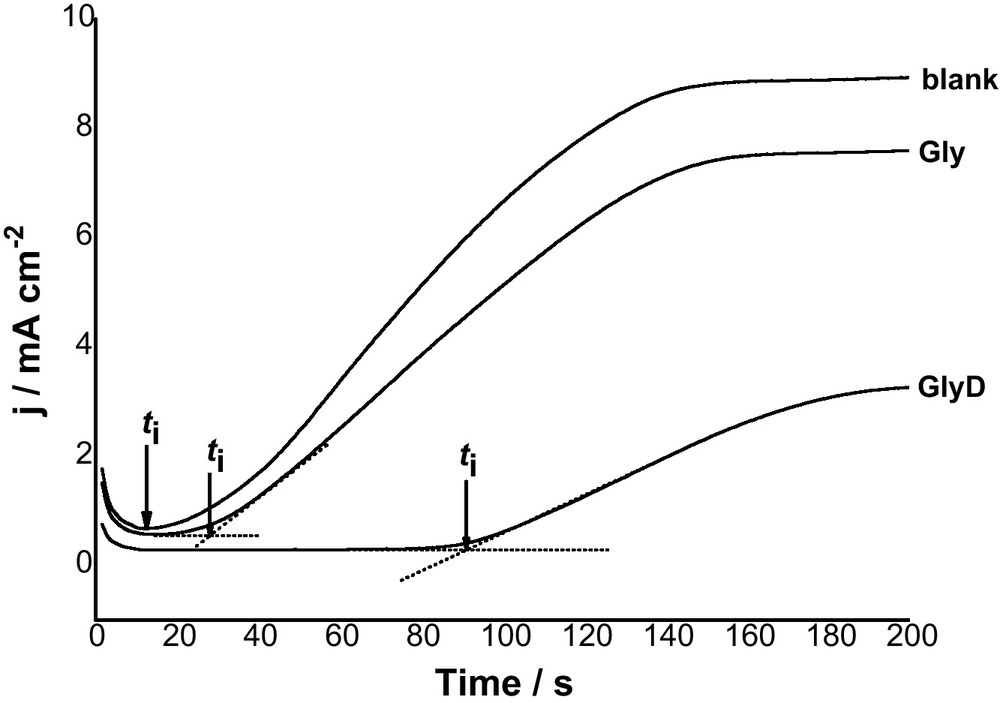
Potentiostatic current-time plots recorded for Al in 0.04 M KSCN solution without and with 10-3 M Gly or GlyD at a an applied anodic potential of 1300 mV (> Epit) at 25 oC.
Addition of both Gly and GlyD decreases Al susceptibility towards pitting. This is clearly seen from the decrease in jpit and the subsequent increase in ti. This decrease in susceptibility towards pitting depends on the type of the introduced inhibitor, with GlyD being more effective than Gly. These findings support results of polarization measurements that the presence of these inhibitors protects Al against the aggressive attack of SCN− anions.
Fig. 4 presents the dependence of the anodic potential on time for anodization of Al in 0.04 M KSCN solutions without and with 10−3 M Gly or GlyD at a current density of 50 μA cm−2 at 25 oC. The linear increase in potential at the commencement of anodization accompanies the initial formation and growth of the oxide film. A potential maximum appears at a certain time, assigned as the incubation time, ti, which is necessary before pit growth to occur. This maximum is thought to correspond, as will be shown, to the pitting potential (Epit). The appearance of which is the correspondence of competition between two processes, namely, further oxide film growth and its breakdown.

Galvanostatic potential-time plots recorded for Al in 0.04 M KSCN solution without and with 10-3 M Gly or GlyD at a an applied anodic current of 50 μA at 25 oC.
The decline in potential following the maximum corresponds to breakdown of passive layer followed by the nucleation and formation of pits as a result of the aggressive attack of SCN− anions [1]. After prevalence of the latter reaction at Epit, or in other words, after ti, the potential drops to a steady value, corresponding to the repassivation potential (Erp). It obvious that the values of Erp obtained from cyclic voltammetry [1] and galvanostatic measurements are in good agreements. These findings confirm that the potential maximium observed in the E/t plots corresponds to Epit.
It follows from the data of Fig. 4 that the rate of potential increase (i.e., the rate of oxide growth) and the value and location of potential maximum depend on the type of introduced inhibitor. The value of the potential maximum (i.e., Epit) increases and its location shifts towards more positive value (i.e., longer ti values) in presence of these inhibitors. These findings confirm results of polarization measurements that these organic compounds inhibited pitting corrosion of Al in KSCN solutions.
The formation of a protective film of inhibitor is confirmed from SEM examinations of the electrode surface. Fig. 5 presents SEM images recorded for Al in 0.04 M SCN− solutions without and with 10−3 M Gly or GlyD. It is quite clear that the surface morphology varies depending on the type of inhibitor. Severe pitting attack is observed in absence of the inhibitor (see image a); a large number of small pits were observed. On the other hand, in presence of both Gly and GlyD, the corroded areas obviously diminished. Significant decrease in corroded areas was again observed in presence of GlyD (image c) more than in presence of Gly (image b). Pitting attack still appears in case of Gly. Surface morphology in presence of GlyD, image c, showed that pitting attack is prevented and the surface is efficiently separated from the corrosive medium.

SEM images of Al in 0.04 M KSCN solutions at 25 oC without (image a) and with 10-3 M Gly (image b) or 10-3 M GlyD (image c). The electrode is potentiostatic held at 1300 mV (> Epit recorded for Al in 0.04 M KSCN) for 5.0 min at 25 oC.
The pH of the potential of zero charge (PZC) for aluminium oxides/hydroxides is 9 [8,9]. Depending on solution pH, the electrode surface can bear net negative, or positive, or no charge. In 0.04 M KSCN solution (pH 6.8), therefore oxide surface is positively charged. Addition of GlyD provides KSCN solution with Cl− anions. These Cl− anions adsorb, in competition with SCN− anions [1], at the oxide/solution interface through electrostatic attraction force because of the excess positive charge at this interface at the corrosion potential. This process changes the solution side of the interface from positive to negative. Thus, the quaternary ammonium cations in the GlyD molecule, −H-N+(CH3)2, electrostatically adsorb on the oxide surface covered with primarily adsorbed Cl− and/or SCN− anions. Detection of Cl and N signals on the electrode surface using EDX spectra (not shown here) may confirm this type of adsorption.
The group –Ph– in the GlyD structure is a donor of electron and it may offer itself the possibility to be a center of adsorption beside nitrogen atoms. It is probable that, in case of GlyD, the adsorbed nitrogen atoms are oriented with the benzene ring structure parallel to the metal surface (due to aromaticity of the GlyD molecule). This mode of adsorption suggests that the adsorbed GlyD molecule covers a large area, thereby inhibiting Al corrosion effectively more than Gly.
4 Conclusion
The presence of glycine and its derivative in KSCN solutions inhibited uniform and pitting corrosion process. Corrosion and passive currents were reduced, and the pitting potential was increased with increase in additive concentration. Transient measurements showed that pit initiation and growth decreased in presence of these organic compounds. The inhibition efficiency of the newly synthesized glycine derivative was found to be higher than that of glycine itself. Polarization measurements showed that glycine and its derivative acted as mixed-type inhibitors with cathodic predominance. SEM examinations of the electrode surface showed that the aggressive pitting attack of SCN– anions decreased with increase in additive concentration.