Metal string complexes that contain a 1D linear transition metal backbone are considered to be potential molecular electronic devices [1]. The general expectation is to transport an electron through the continua of metal wire-like frameworks that are similar to the electric wires in our macroscopic world. In addition to this potential application, the studies of metal strings can also provide useful information for understanding the metal-metal bonds [2]. Because of these significances, numerous metal string complexes have been synthesized and studied in the past two decades [1].
Among the family of metal strings, the linear metal framework of most reported complexes consists of an odd number of metal atoms [1]. The physical properties and electric conductance of odd-numbered metal strings therefore have been studied fruitfully. Compared with the odd-numbered metal stings, research on even-numbered metal stings remains relatively underdeveloped.
The even-numbered metal strings, however, show different physical properties than odd-numbered analogues do [3]. For example, two axial coordinated water molecules of the tetranuclear nickel string, [Ni4(Tsdpda)4(H2O)2], can be removed by one-electron oxidation (Tsdpda2− = N-(p-toluenesulfonyl)dipyridyldiamido) [3a]. The flexibility of the axial interactions suggests that the geometry and electronic structure of even-numbered metal strings may be tuned by carefully modifying the axial interactions, which was rarely observed in the middle of odd-numbered metal strings. Because of this interesting character, one research goal in our group is to synthesize and to study new even-numbered metal stings, which can help scientists to gain more insights into the design of future molecular wires.
In our previous report, the [Ni4(Tsdpda)4(H2O)2] and its one-electron oxidized product, [Ni4(Tsdpda)4]+, show different electronic structure due to both the oxidation states and the leaving of axial coordinated water molecules [3a]. These concurrent factors result in difficulties for studying the variation of electronic structures of tetranickel strings upon one-electron oxidation. Before we proceed to develop more even-numbered metal strings, we think it is worth understanding the details of tetranickel strings. In this study, we thus report the synthesis, crystal structure, magnetic properties and DFT calculations of [Ni4(DAniDANy)4](PF6) (2), which is the one-electron oxidized product of the previously reported [Ni4(DAniDANy)4] (1) compound [3a]. Since both compounds possess no axial ligands, the [Ni4(DAniDANy)4](PF6) directly shows the variations of geometry and electronic structure upon one-electron oxidation.
The H2DAniDANy ligand and tetranickel string, [Ni4(DAniDANy)4], were prepared according to the literature procedures (Scheme 1) [3a]. The one-electron oxidized product, [Ni4(DAniDANy)4](PF6), was obtained by reacting [Ni4(DAniDANy)4] with FcPF6 in CH2Cl2 [4]. The crystal structure of 2 is shown in Fig. 1 [5]. The core structure of 1 and 2 are similar. Both compounds consist in a linear tetranickel chain helically wrapped by four DAniDANy2− ligands, which display approximate D4 symmetry.
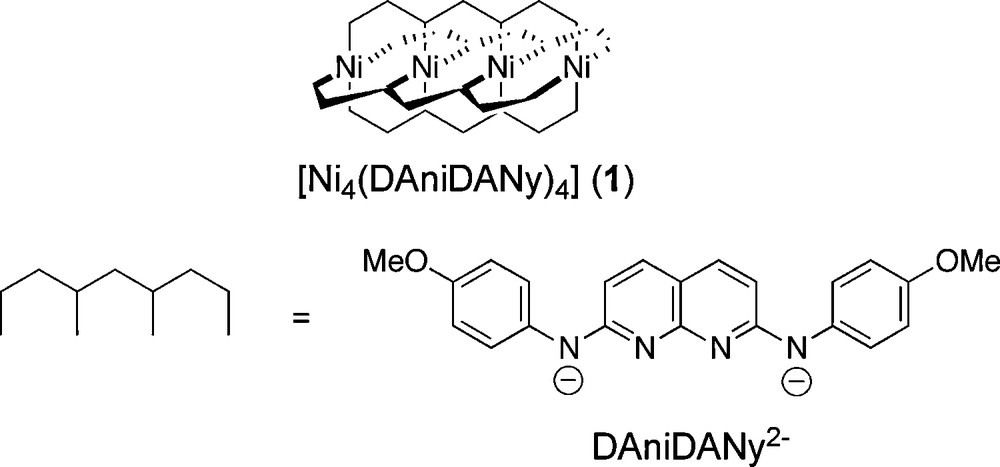
[Ni4(DAniDANy)4] (1) and DAniDANy2−.
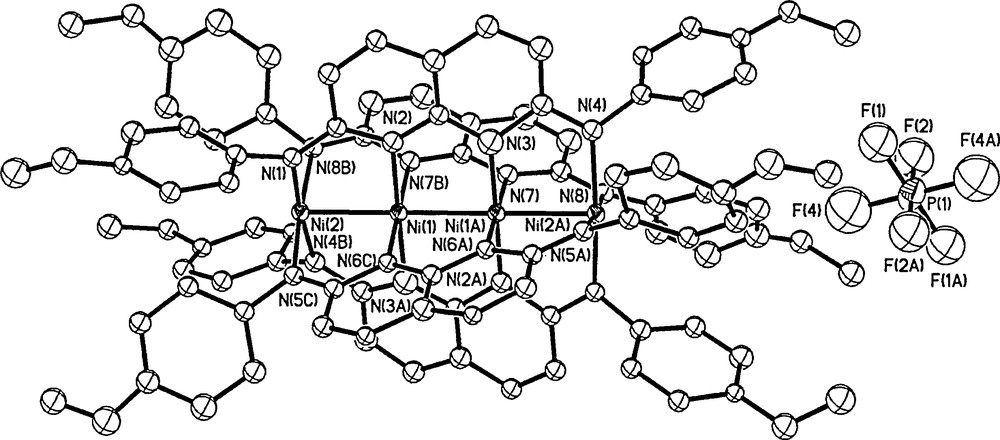
Crystal structure of 2. Thermal ellipsoids are drawn at the 30% probability level. Hydrogen atoms and solvent molecules are omitted for clarity.
Selected bond lengths observed for 2 are reported in Table 1, together with the corresponding values reported for 1 [3a]. The terminal and internal Ni–N bond distances of 2 are 2.326 and 2.309 Å, respectively, which are ∼0.03 Å shorter than those in 1. Considering the oxidation of 1 increases the electrostatic repulsion between Ni ions, the Ni–Ni distances in 2 should be elongated. The contraction of Ni–Ni bond lengths in 2 thus indicates that the formation of significant attractions between Ni ions instead of electrostatic repulsions. Such structural variations are similar to those of the oxidation of [Ni4(Tsdpda)4(H2O)2], which suggests the formation of Ni–Ni bonds [3a]. However, the oxidation of [Ni4(Tsdpda)4(H2O)2] accompanies the loss of axial coordinated water molecules, which also shorten the Ni–Ni bond lengths due to the trans effect. The contraction of Ni–Ni bond lengths in 1 upon oxidation clearly indicates that the one-electron oxidation of tetranickel string mainly results in Ni–Ni bond formation.
Selected bond distances (Å) of 1a and 2.
1 | 2 | |
Ni-Ni terminal | 2.356 (1) | 2.326 (1) |
Ni-Ni internal | 2.332 (1) | 2.309 (2) |
Ni-N terminal | 1.912 (3) | 1.898 (9) |
Ni-N internal | 1.912 (3) | 1.903 (11) |
a Reference [3a].
Magnetic susceptibility measurements for compounds 1 and 2 were made on polycrystalline samples in the temperature range 5–300 K. As previously reported, compound 1 is diamagnetic [3a]. The magnetic behaviour of 2 is different from that of 1. Temperature-dependent χmT versus T is displayed for 2 in Fig. 2. The χmT value for 2 is ca. 0.368 cm3 K mol−1 at 300 K and remains constant down to 15 K. This value indicates that compound 2 possesses one unpaired electron (theoretical value: 0.375 cm3 K mol−1). Furthermore, the axial EPR spectrum (g⊥ = 2.17 and g∥ = 2.03) obtained at 77 K for 2 is coincident with the feature of the 3d7 Ni(III) ion (S = 1/2) in an elongated octahedral geometry, which supports the previous oxidation reaction and magnetism (Fig. 2, insert) [4].

Plot of χmT versus T of complex 2. Insert: powder X-band EPR spectrum of 2 at 77 K.
Cyclic voltammetry of compound 1 in CH2Cl2 solution showed three reversible one-electron oxidation processes at potentials of 0.13, 0.87 and 1.02 V, respectively (Fig. 3). The first oxidation process is assigned to the loss of one electron from 1, leading to the formation of 2. Fig. 4 shows the spectral changes in the near-IR range observed for compound 1 at applied potential from 0 to 0.30 V in CH2Cl2 containing 0.1 TMAP. A new broad band at 1150 nm appears as the applied potential increases, which is attributed to the inter-valence charge transfer (IVCT) transition [5]. This IVCT band is a character of the electron delocalization within the mixed-valence complex 2 [2h,6].
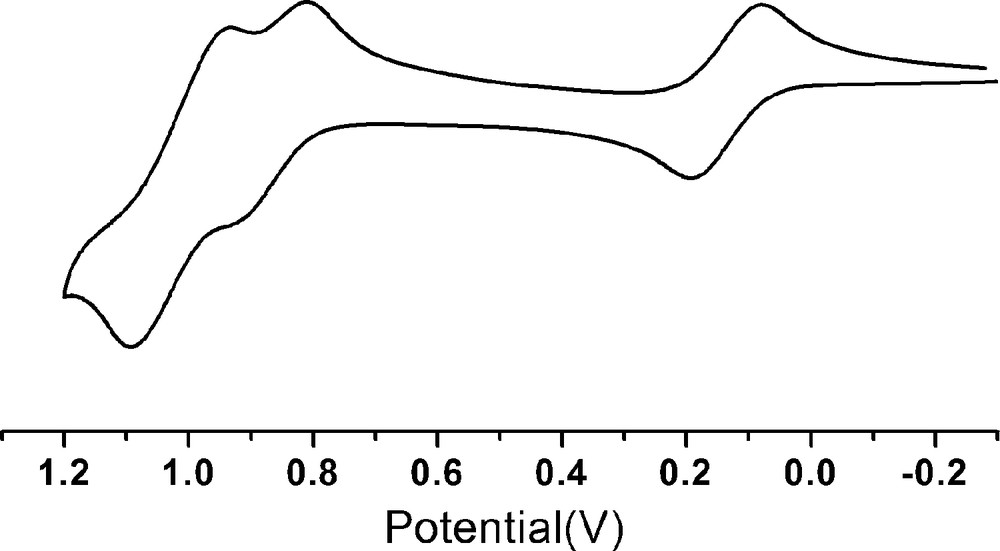
Cyclic voltammograms of 1 in CH2Cl2 containing 0.1 M TBAP with scan rate = 100 mVS−1.

UV-Vis and near-IR spectral changes for the first oxidation of compound 1 in CH2Cl2 with 0.1 M TBAP at various applied potentials form 0 to +0.30 V.
To obtain further insight into the electronic structures of compounds 1 and 2, a series of DFT/BP86 calculations was performed using ORCA [7,8]. For the sake of simplicity, the anisyl groups of the ligand DAniDANy2− are replaced by methyl groups. The geometries of compounds 1 and 2 were optimized on the singlet state for 1 and doublet state for 2. The z-axis is assumed to be collinear with the tetranickel framework. Some relevant geometrical parameters and results from DFT calculations are listed in Table 2. The optimized geometrical parameters are in good agreement with the experimental data.
Selected DFT calculated bond lengths (Å) of compounds 1 and 2 in the gas phase.
1 | 2 | |
Ni-Ni terminal | 2.366 | 2.338 |
Ni-Ni internal | 2.350 | 2.331 |
Ni-N terminal | 1.911 | 1.908 |
Ni-N internal | 1.938 | 1.936 |
In a linear tetranickel system, the d orbitals of the transition metals generate three types of molecular orbitals: (1) σ-type MOs (σ, σ(1), σ(2) and σ*), composed of dz2 combinations; (2) π-type MOs (π, π(1), π(2) and π*), composed of dxz and dyz orbitals; (3) δ-type MOs (δ, δ(1), δ(2) and δ*), composed of dxy orbitals [3a]. The qualitative molecular orbital diagram is shown in Fig. 5. The computed electronic configuration of 1 is (σ)2(σ(1))2 (π and δ block)24 (σ(2))2 (σ*)2. Since all the bonding and antibonding orbitals of 1 are doubly occupied, there are no Ni-Ni bonding interactions in 1. On the contrary, calculations indicate that the electronic configuration of 2 is (σ)2(σ(1))2 (π and δ block)24 (σ(2))2 (σ*)1, which suggests that the removal of one electron from the σ* antibonding orbital occurs upon oxidation. The one-electron oxidation of 1 gives the product 2 with partial Ni–Ni σ-bonds, which supports the observed shortening in the Ni-Ni distances from 1 to 2. The electrons on the σ orbitals are proposed to delocalize over the tetranickel framework through Ni–Ni σ-bonds, which is confirmed by the mulliken atomic spin densities (Scheme 2). Moreover, the observed IVCT band of 2 may be assigned qualitatively to the transition from lower doubly occupied σ, σ(1) or σ(2) orbitals to the highest singly occupied σ* orbital [9].

The qualitative molecular orbital diagram of the linear tetranickel system (left). The computed electronic configuration of compounds 1 and 2 (right).

Computed mulliken atomic spin densities of compound 2.
In summary, in order to study the structural change of tetranickel string which results from one-electron oxidation, we have oxidized the [Ni4(DAniDANy)4] (1) and isolated its one-electron oxidized product [Ni4(DAniDANy)4](PF6) (2). The magnetic measurements show that 1 and 2 exhibit singlet and doublet ground states, respectively, which were confirmed by EPR spectra. DFT calculations suggest that the chemical oxidation of 1 involves removal of the electron from the σ* antibonding orbital (Fig. 5), which leads 1 to form Ni-Ni bonds upon oxidation. Considering this oxidation process is reversible (Fig. 3), these tetranickel strings are along the line toward potential applications in molecular switches (Scheme 3) [10]. The studies of the shortest even-numbered tetranickel string, 1 and 2, therefore, provide more insights into not only the development of future longer even-numbered metal strings but also the design of molecular switches.
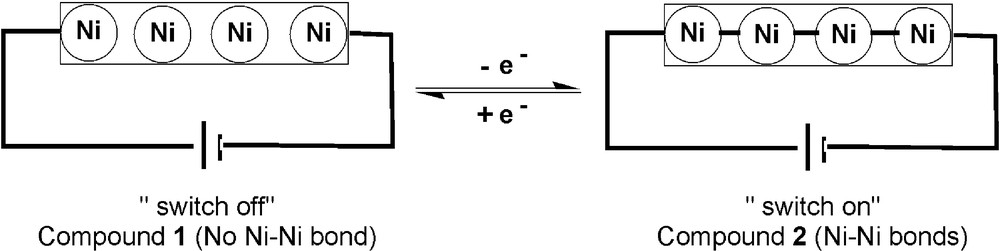
The proposed redox-controlled molecular switch.
Acknowledgment
The authors thank the National Science Council and the Ministry of Education of the Republic of China (Taiwan, ROC) for financial support.