1 Introduction
Transesterification is one of the classic organic reactions that have enjoyed numerous laboratory uses and industrial applications. Organic chemists make use of this reaction quite often as a convenient means to prepare esters. In general, transesterification reactions can be accelerated by both acids and bases, in either homogeneous or heterogeneous conditions; they can also occur under neutral conditions [1]. Thus, a number of procedures for transesterification have been reported, which are catalyzed by traditional acids, organic bases. However, not only the protocols reported by transesterification are time consuming but also the catalysts used are toxic, corrosive, expensive and produce large amounts of byproducts which are difficult to separate from the reaction mixture. In view of environment and economical concern, it is still remarkable to design a catalyst which is easily separable from the products, less toxic and reusable.
Malonic esters are known for their importance either as starting materials or intermediates in organic fine chemicals synthesis [2,3]. Generally the synthesis of benzyl malonate is associated with the use of either toxic or lachrymetric reagents under homogenous conditions. The synthesis of substituted malonates via transesterification reaction in the presence of heterogeneous catalysts has been found to be a better route than the conventional methods. However, not only the protocols followed here were time consuming but also the catalysts used were toxic or expensive [4,5]. Further, the synthesis of bulky dibenzyl malonate (DBM) via transesterification is found to be challenging due to porous diffusional limitations of the heterogeneous catalysts.
Aluminophosphate (ALP) and metal aluminophosphates (MALP) are well known for their applications as heterogenous catalysts in organic fine chemical synthesis [6]. The catalytic activity of the heterogeneous catalysts has been found to depend on their pre- and postsynthesis conditions [7]. There are several attempts made on the modification of the acid-base properties of ALPs by incorporation of a transition metal [8,9] to investigate their influence on the textural properties and catalytic activity.
As a part of our on-going research, we have reported [10] the dependence of the structure and catalytic activity relationship of amorphous MALPs for the synthesis of biphenyl urea via alkylation. The effect of preparation and iron loading on the transesterification activity of iron ALPs were already studied and found that catalyst prepared by dropwise addition of ammonia with Fe: Al: P (0.025: 0.95: 1) mole ratio exhibited highest activity for the selected reaction [11].
The main objective of this study was to investigate the effect of transition metal ions on the textural properties of modified ALPs and hence on their catalytic activity in the transesterification of diethyl malonate (DEM) with benzyl alcohol (BA). Furthermore, the scope, generality and ecofriendly nature of the iron ALP catalyst are demonstrated by conducting transesterification of DEM using various aliphatic, alicyclic and aromatic alcohols. Optimization reactions were also conducted over FeALP in the same reaction which led us to identify the reaction conditions for high yield and selectivity for the desired transesterification products. A possible mechanism of the catalytic reaction was also proposed.
2 Experimental
2.1 Preparation of the catalysts
MALPs (M = V, Fe, Co, Ni and Cu) were prepared by a simple precipitation method in the absence of templates. Aluminum nitrate, the transition metal salt and orthophosphoric acid in the molar ratio 0.95 (Al): 0.025 (M): 1 (P) were dissolved in 500 cm3 deionised water and stirred mechanically for 1 h at 60 °C. Aqueous ammonia (28%) was added drop wise at a rate of 0.5 ml/min from a microburette until the pH of the solution rose to 9. The precipitate obtained was filtered, washed several times with deionised water to remove the soluble impurities. The solid obtained was dried at 120 °C for 12 h and calcined at 550 °C for 5 h. The same method was followed but in the absence of transition metal salt to prepare pure ALPs.
2.2 Characterization of catalysts
The percentage composition of the transition metal, aluminum and phosphorous in the catalyst was determined by spectrophotometric methods [12]. Powder X-ray diffraction (PXRD) patterns of the catalysts were recorded in the range of 2θ = 2°–70° using an X-pert Pro Philips diffractometer equipped with Ni filtered Cu-Kα radiation λ = 1.5418 Å using a graphite crystal monochromator. FTIR spectra were recorded over a range of 400–4000 cm−1 using Philips Nicolet IR 200 instrument using the KBr pellet technique. The specific surface area, pore diameter and pore volume were evaluated by NOVA 1000 Quanta chrome high-speed gas sorption analyzer instrument. In this analysis, the samples were degassed at 523 K for 5 h before measurements. Specific surface area was calculated using the BET equation. The pore volume and pore size distributions were estimated at a relative pressure of 0.94 (p/p0) and N2 adsorption-desorption isotherms using BJH model respectively. Total surface acidity by NH3-TPD was determined using Puls Chemisorb 2705 from Micromeritics. Here, 100 mg of fresh sample was degassed under a helium stream for 1 h, ammonia (99.99%) gas was injected into the stream until saturation was reached, cooled to 323 K. The system was maintained at 323 K for 30 min. After purging, the system with flowing helium for 2 h at 323 K, the sample was heated at the rate of 5 K min−1 in helium to 973 K. The change in concentration of the desorbed NH3 was monitored using an online thermal conductivity detector.
2.3 Catalytic activity
The catalytic activity of ALP and MALP was studied in liquid phase transesterification reaction of DEM with BA (Scheme 1). In a typical reaction, a mixture of 0.5 g of the catalyst, 5 mmol of DEM and 15 mmol of BA was taken in a 100 ml RB flask fitted with water-cooled condenser and heated in temperature controlled oil bath. The reaction mixture was stirred magnetically at 110 °C for 4 h. Aliquots of the reaction mixture were periodically withdrawn and subjected to gas chromatographic analysis. After the stipulated time, the reaction mixture was cooled to room temperature and centrifuged to separate the solid catalyst. The components in the filtrate were analyzed by GC-MS.

Transesterification of diethyl malonate with benzyl alcohol.
3 Results and discussion
3.1 Composition and surface area
The abbreviations used and the physico-chemical properties of the catalysts are given in Table 1. The percentage composition of the metal, aluminium and phosphorous in the catalyst were found to be in the expected range according to the formula; M0.025 Al0.95 P1. The specific surface area of pure ALP was lower than the MALP, indicating the influence of transition metal on the textural properties of ALP. The increase in surface area can be explained as follows: the strong interaction of metal in the form of oxide or phosphate with the ALP reduces the surface diffusion of ALP and inhibits the sintering of the material. The maximum surface area was exhibited by the FeALP (181 m2/g) materials compared to other catalysts used.
Physico-chemical properties of metal aluminophosphates (MALP) catalysts.
Sl No | Catalyst | Surface area (m2/g) | Total acidity NH3-TPDa | Pore diameter (nm) | Total pore volume (cm3/g) |
1. | FeALP | 181.4 | 12.058 | 7.98 | 0.362 |
2 | CoALP | 125.1 | 10.786 | 14.5 | 0.456 |
3 | CuALP | 84.51 | 8.401 | 11.9 | 0.252 |
4 | NiALP | 171.0 | 7.395 | 9.84 | 0.407 |
5 | VALP | 110.3 | 7.386 | 12.3 | 0.426 |
6 | ALP | 78.6 | 6.723 | 5.48 | 0.107 |
a mg of NH3/g of catalyst.
3.2 Powder X-ray diffraction
The PXRD patterns of ALP and MALP calcined at 550 °C are given in Fig. 1. All the samples except FeALP exhibited a broad band in the range 2θ = 15°–30° range. This feature of PXRD is characteristic of amorphous ALPs [13]. FeALP exhibited sharp diffraction peaks at d = 3.8 (2θ = 23.3°), 3.3 (2θ = 26.99°) and 2.3 Å (2θ = 38.5°), indicating the presence of an iron aluminum phosphate phase [JCPDS File No.: 210006]. It is an indication of the intervention of iron species in the formation of amorphous aluminum phosphate. Thus, PXRD pattern clearly revealed the amorphous nature and low degree of crystallinity of the materials.
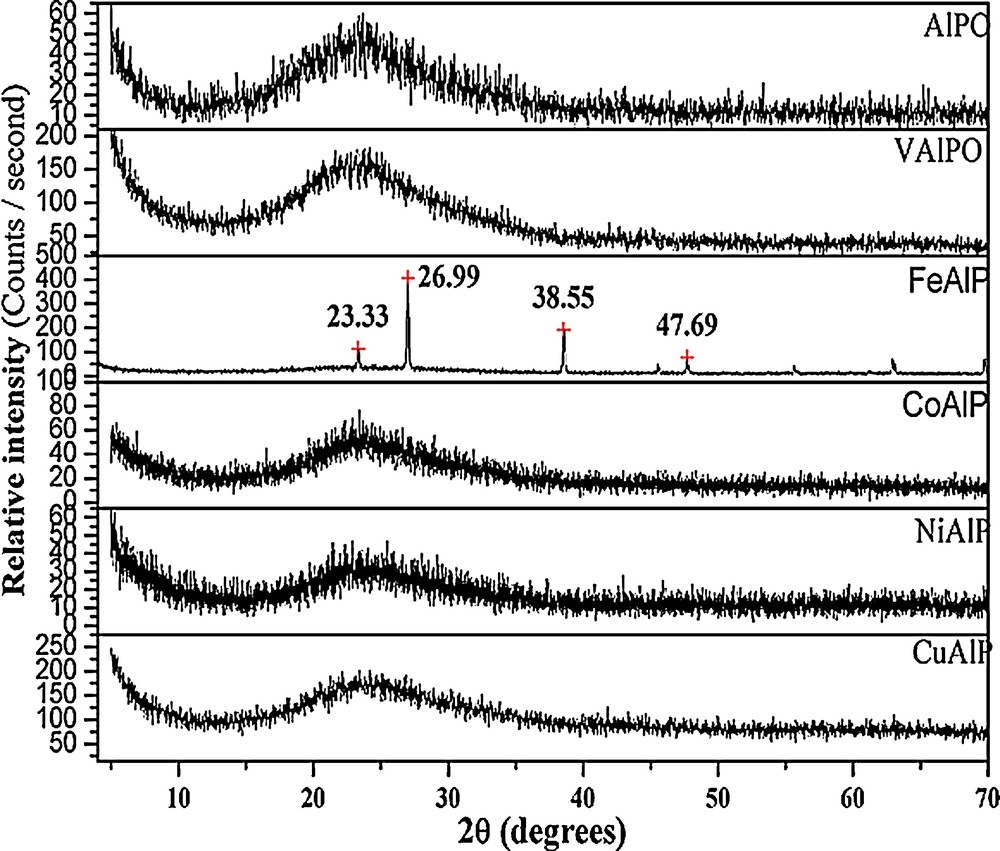
Powder X-ray diffraction (PXRD) patterns of aluminophosphate (ALP) and metal aluminophosphates (MALP) catalysts.
3.3 FTIR spectra
The FTIR spectra of ALP and MALP are given in the Fig. 2. A broad absorption band in the range 3430–3448 cm−1 is attributed to the surface hydroxyl groups, which may be associated with transition metal, aluminum and phosphorous atoms [14]. The intensity of the band is significantly higher in the case of MALP's compared to ALP. Broad and weak absorption bands around 1623–1640 cm−1, especially in the case of MALP's indicated the presence of adsorbed water molecule. The broad and strong bands in the range 1100–1128 cm−1 exhibited by all the samples is due to the asymmetric vibration of phosphate (triply degenerate P–O stretching vibration) [15]. The peak centered at 478–490 cm−1 is assigned to the triply degenerate bending vibration of O–P–O bond in pyrophosphates. All the samples except NiALP and CuALP exhibited absorption at 691 cm−1 due to stretching vibrations of Al–O and P–O bonds [16]. It is also noteworthy that the bending vibration of the hydroxyl groups and the phosphate groups assigned in the range as indicated above were sensitive to the nature of the transition metal. The centre of these bands has been shifted to the lower wave number with increase in the atomic number of the metal. Thus, FTIR studies indicated clearly the presence of surface hydroxyl groups and the phosphate moiety in ALP and MALP's.
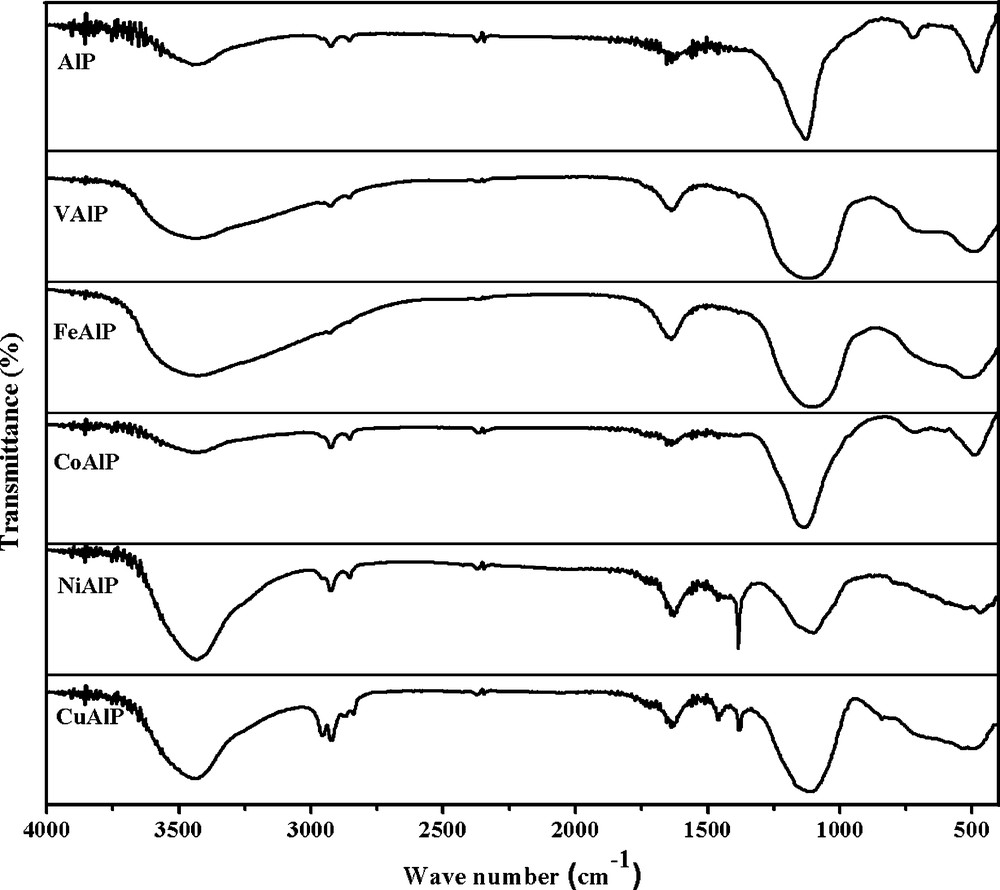
FTIR Spectra of aluminophosphate (ALP) and metal aluminophosphates (MALP) catalysts.
3.4 N2 adsorption–desorption isotherms
The adsorption–desorption isotherms of ALP and MALP were nearly the same, and belonged to type IV category (Fig. 3) indicating the mesoporous nature of the materials [17]. Further except FeALP, all other materials exhibited H1 type hysteresis loops indicating difference in the type of pores. The nitrogen adsorption–desorption isotherms of FeALP exhibited H2 loop. The appearance of H2 hysteresis loop between the 0.4–0.7 indicated the condensation and evaporation of adsorptive liquid occurring from the pores with narrow necks and wide bodies referred to as ‘ink bottle’ pores [18]. The steep rise in adsorbed volume of nitrogen at p/p0 > 0.4 represented narrow pore size distribution in FeALP. These observations indicated efficient adsorptive capacity of FeALP as well as significant difference in its textural properties compared to other MALP's.
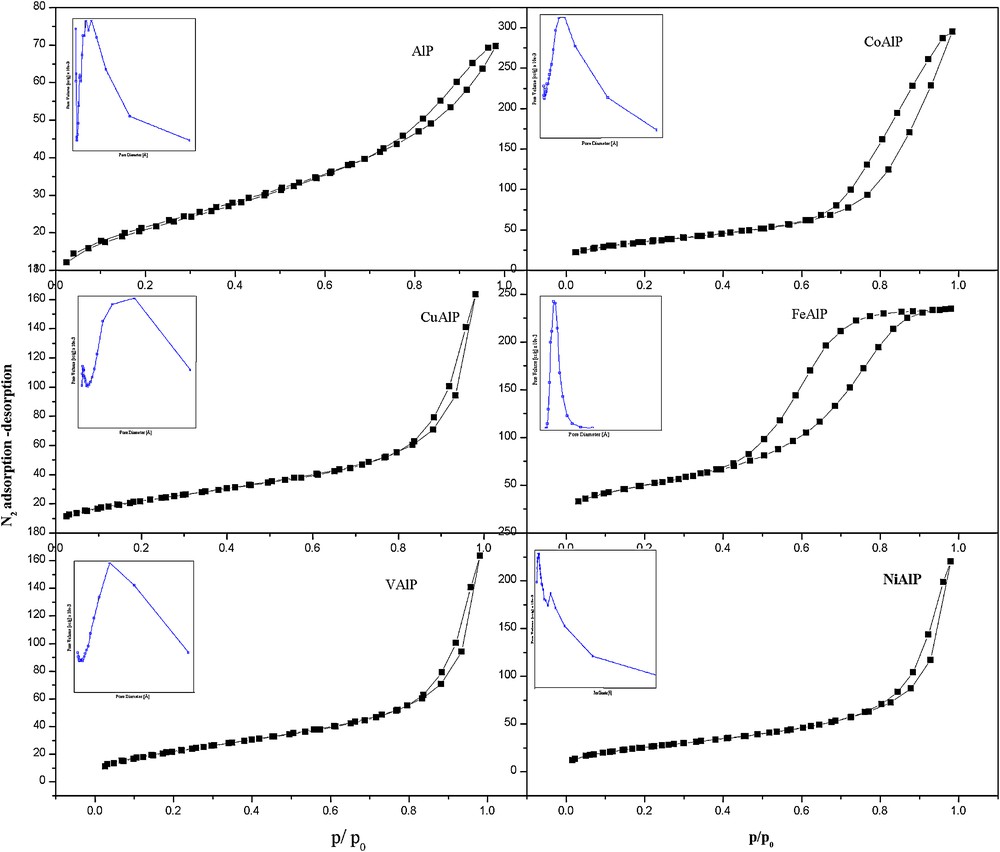
Nitrogen adsorption–desorption isotherms of aluminophosphate (ALP) and metal aluminophosphates (MALP).
3.5 NH3–TPD studies
The concentration and strength of the surface acid sites on ALP and MALP were determined by NH3–TPD method. The total acidity in terms of mg of NH3 per g of the catalyst is presented in Table 1. All the samples were found to possess weak to moderate strength acid sites. The surface acidity is attributed to the presence of Al–OH and P–OH groups in amorphous matrix. In the case of ALP, complete desorption of ammonia occurred below 100 °C indicating the presence of only very weak acid sites [19]. However, desorption of ammonia from MALP occurred in the temperature range 180–300 °C. This showed the presence of stronger acid sites on MALP compared to ALP. Further the broad temperature range (180–300 °C) of desorption of ammonia revealed that the surface acid strength is widely distributed. In the case of FeALP, the NH3– TPD profiles were broader and occurred at higher temperature regions. It is a clear indication that the acid sites concentration as well the strength was higher on FeALP compared to other MALP's [20].
Thus PXRD, FTIR, BET surface area, N2 sorption and NH3 TPD studies indicated that the textural properties of ALP were modified by incorporation of transition metal ions and FeALP sample was distinctly different from other MALP's in its textural properties.
3.6 Catalytic activity studies
The catalytic activity of ALP and MALP's was investigated in the transesterification reaction between BA and DEM. The bulky reactant molecules were consciously chosen to derive information on the effect of textural properties mainly pore size of the amorphous MALP's on their catalytic activity.
The transesterification activity of ALP and MALP's in terms of the percentage conversion of DEM into benzyl malonates along with the selectivity towards the mono- and dibenzyl transester products are given in the Table 2. The catalytic activity of the materials was in accordance with the increasing order of their total surface acidity as indicated by NH3-TPD studies. The yield and selectivity of the transester products altered with the variation in density and strength of the acid sites.
Transesterification activity of aluminophosphate (ALP) and metal aluminophosphates (MALP) in the reaction between diethyl malonate (DEM) and benzyl alcohol (BA).
Sl No: | Catalyst | Conversion of DEM (%) | Selectivity for BEM (%) | Selectivity for DBM (%) |
1 | FeALP | 87.7 | 49.4 | 50.6 |
2 | CoALP | 74.9 | 33.8 | 66.2 |
3 | CuALP | 45.2 | 43.1 | 56.9 |
4 | NiALP | 40.3 | 57.7 | 42.3 |
5 | VALP | 40.1 | 50.9 | 49.1 |
6 | ALP | 35 | 100 | 0 |
Pure ALP favored the formation of only benzylethyl malonate (BEM). NH3-TPD studies on this material revealed the presence of only weak acid sites on its surface. Hence acid sites with weak strength associated with the surface hydroxyl groups are the active centres for the formation of BEM. MALP, on the other hand, favored the formation of both BEM and DBM. Thus the transition metal ions are the catalytically active centres that are responsible for the formation of DBM. This aspect is further clear from the fact that the pure ALP and V, Ni, Cu containing MALP's possessed comparable surface acidity but only transition metal containing samples exhibited activity towards the formation of DBM. Further Fe and Co containing ALP exhibited significantly higher activity for DBM formation. This is due to their higher total surface acidity compared to other transition metal containing ALP. It is further interesting to note that FeALP exhibited higher total transester yield compared to CoALP. This difference in catalytic activity may be attributed to the difference in the pore structure between these samples.
FeALP, as indicated by sorption studies, had pores of regular size with diameter in the mesopores range and uniformly distributed through out the sample, which could overcome the porous diffusional constraints and enable the molecules to access all the active sites of acidic nature and hence higher catalytic activity. CoALP and other MALP's exhibited higher average pore diameter value than FeALP, due to large number of irregular pores with smaller diameter. Thus, from these observations, it may be inferred that not only total acid strength but also the textural properties of MALP were responsible for the formation of DBM.
3.7 Optimisation reactions
As FeALP exhibited the highest conversion of DEM and also retained its activity for a longer duration of reaction, further optimization reactions were carried out in presence of this catalyst by varying the molar ratio of the reactants, amount of the catalyst and duration of the reaction.
3.7.1 The effect of molar ratio
An increase in BA content in the reaction mixture increased the percentage yield of DBM with a corresponding decrease in the yield of BEM (Fig. 4). We propose that BEM formed in the first step may further react with excess of BA increasing the amount of DBM (Scheme 2). It is also noteworthy that the total percentage yield of transester products increases with increase in alcohol content. The maximum yield of transester products was obtained when the DEM: BA ratio was 1:3.
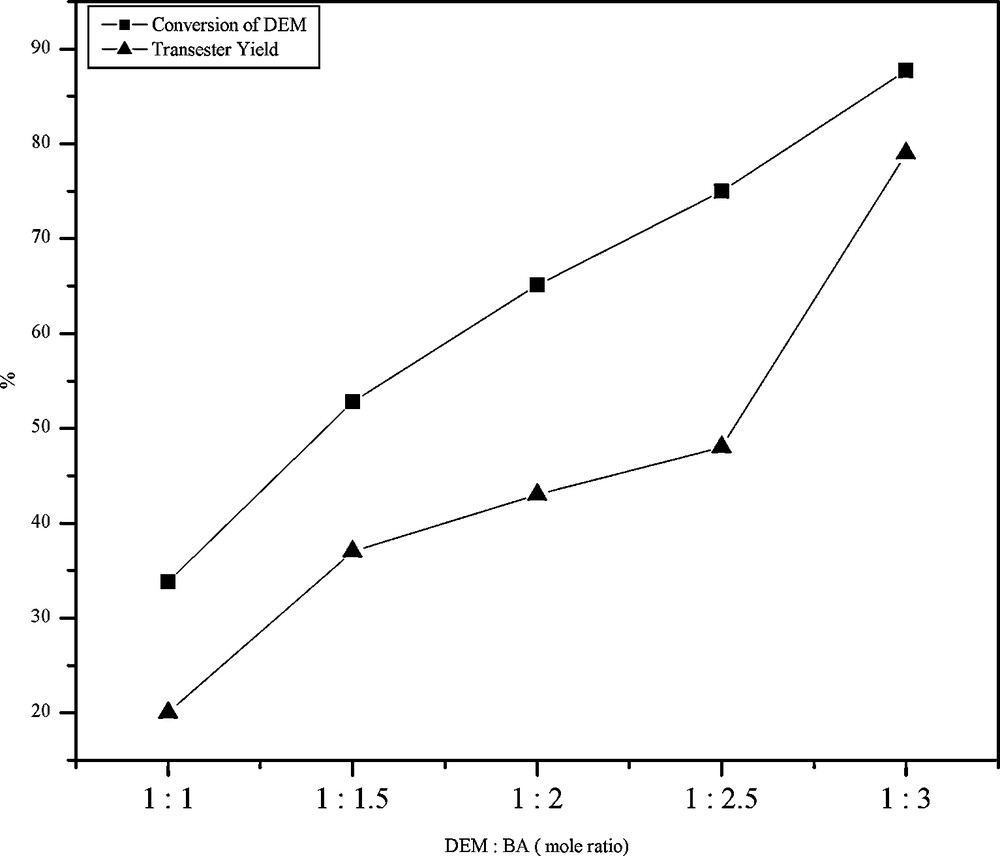
The effect of molar ratio of reactants in the transesterification of diethyl malonate (DEM) with benzyl alcohol (BA) over FeALP catalyst.

Transesterification in two steps in excess of benzyl alcohol (BA).
3.7.2 The effect of catalyst amount
It is clear from the Fig. 5, that the conversion of DEM was only 65.6% when the amount of catalyst was 0.05 g. However, there was a significant increase in the conversion to 79%, when the amount of the catalyst was increased to 0.1 g. Further, increase in the amount of the catalyst up to 0.5 g, did not show any significant change. Thus 0.1 g seems to be an optimum amount of the catalyst to get a good exposure of the reactants to the catalytically active sites and hence obtain products in good yield.

The effect of the amount of FeALP in the transesterification of diethyl malonate (DEM) with benzyl alcohol (BA).
3.7.3 The effect of duration of the reaction
The transesterification reaction was monitored by changing the duration of the reaction. With increase in time the amount of BEM increased up to 4 h then decreased. However, the amount of DBM increased throughout the reaction (Fig. 6). This may be accounted for the disproportionation of initially formed BEM into DBM according to Scheme 3. For the disproportionation reaction to occur, it appears that there is a need of a minimum concentration of BEM, hence the decrease in concentration of BEM is more significant only after 4 h. This aspect is further confirmed by the fact that the percentage conversion of DEM (Fig. 7) almost remained constant after 4 h.
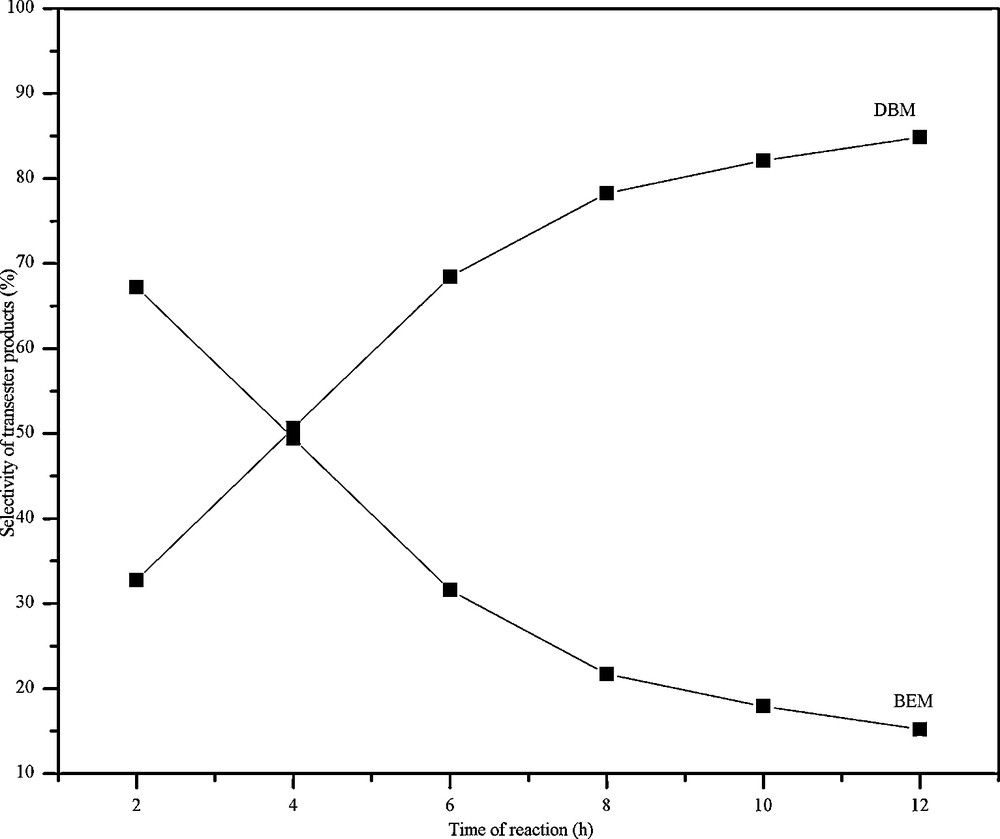
The effect of reaction time in the selectivity of benzylethyl malonate (BEM) & DBM over FeALP catalyst.

Disproportionation of monoester to diester.
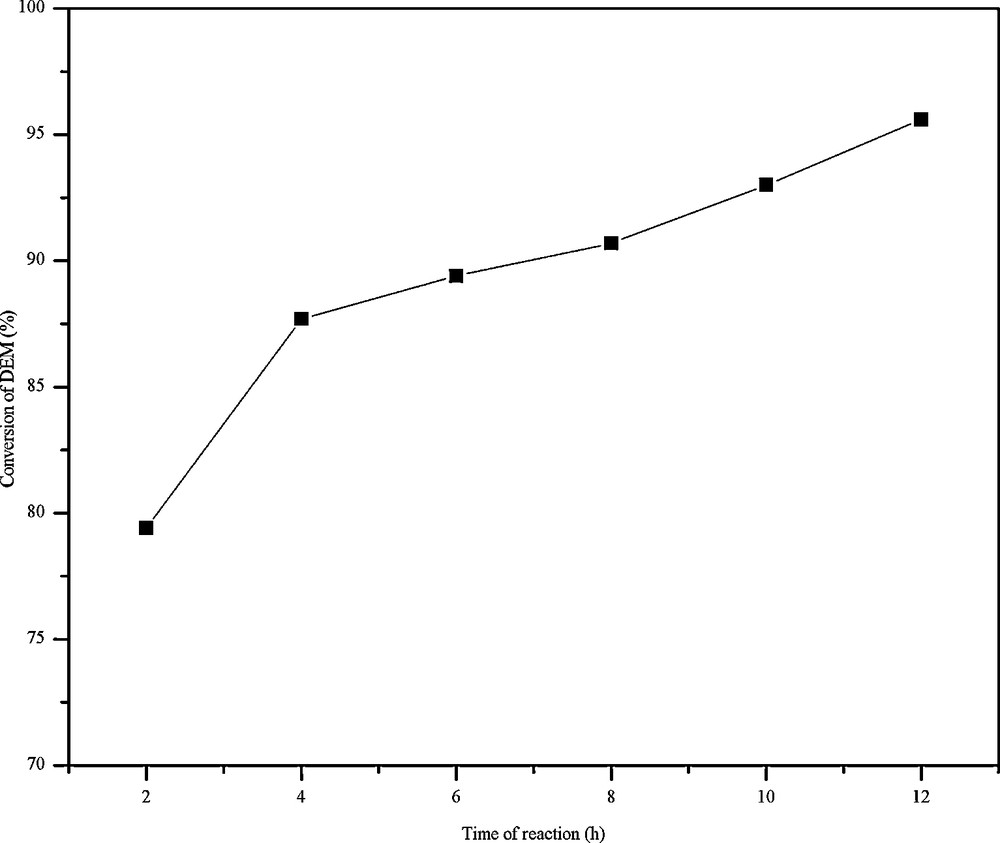
The effect of the reaction time in the conversion of diethyl malonate (DEM) over FeALP in the transesterification of diethyl malonate (DEM) with benzyl alcohol (BA).
4 Scope and generality of the catalyst
The scope and versatility of the FeALP as a catalyst in the transesterification is examined by further conducting the reaction between DEM with different alcohols under the optimized reaction conditions. The results are presented in the Table 3. It is noticed that the transesterification of DEM could be performed with various aliphatic and aromatic alcohols with high efficiency over FeALP catalyst. The percentage conversion of DEM was higher in the case of aliphatic and alicyclic alcohols than with aromatic alcohols. The DEM conversion increased with increase in aliphatic chain length form n-propanol to n-octanol (Table 2, entry 1–5). This may be due to the ease with which the alkoxy group of alcohol generated becomes more facile with increase in the chain length of the aliphatic alcohol, which decreases the electron releasing property of an alkyl group. The generation of alkoxy group is further facilitated by the acid sites of the catalyst.
Transesterification of diethyl malonate (DEM) with different alcohols over FeALP catalyst.
Entry | Alcohol | DEM conversion % | Product selectivity (%) | |
A | B | |||
1 | n-propanol | 83.2 | 32.3 | 67.7 |
2 | n-butanol | 91.3 | 48.8 | 51.2 |
2 | 2-butanol | 87.0 | 55.3 | 44.7 |
3 | n-hexanol | 94.1 | 17.4 | 82.6 |
4 | n-heptanol | 96.5 | 12.1 | 87.9 |
5 | n-octanol | 98.7 | 15.4 | 84.6 |
6 | Cyclohexanol | 100 | 27.4 | 72.6 |
7 | o-Cresol | 100 | 14.3 | 85.7 |
8 | Benzyl alcohol | 85.7 | 15.2 | 84.8 |
9 | Amino benzyl alcohol | 67 | 42.2 | 35.8 |
10 | Hydroxyl benzyl alcohol | 78 | 47.4 | 31.6 |
11 | Benzyl alcohol (RI) | 82 | 15.2 | 84.8 |
12 | Benzyl alcohol (RII) | 80 | 15.2 | 84.8 |
Though in the present studies, the percentage conversion of DEM with BA was low, (Table 3, entry 8) the yield and selectivity for DBMs was significantly higher compared to the catalysts reported in the literature [3,4,21]. Substituted BAs (Table 3, entries 9, 10) showed very low conversion and selectivity for DBMs. Thus the conversion of DEM and the product selectivity (monoester/diester) depended on the structural properties of the alcohol and total acidity and pore structure of the catalysts. The reusability of the catalyst was also investigated in the reaction of DEM with BA and found to be recyclable without loss of its activity for at least two cycles. (Table 3, entries 11 and 12).
It is noteworthy from the reported literature that several attempts have been made to synthesize diaryl malonate using different catalysts. However, the duration of reaction, preparation, cost and recyclability of the catalyst, selectivity of the products were not advantageous. In this report, we have demonstrated that dialkyl as well as diaryl malonates can be synthesised with high yield in short duration using FeALP catalyst prepared by a simple precipitation method.
5 Conclusions
In the present investigation, the modification of the surface and bulk properties of ALPs by the incorporation of transition metals is demonstrated. Structure and catalytic activity correlations have been made in the transesterification between DEM and BA. Pure ALPs resulted in the replacement of only one of the ethoxy groups of DEM whereas the presence of transition metal also resulted in the replacement of both the ethoxy groups. Iron ALP has been found to be the most efficient catalyst in terms of percentage conversion of DEM, transester selectivity and product yield. In the case of bigger molecules like BA; the formation of benzyl ethyl malonate was favored by weak acid sites and the formation of DBM was due to the mesopores with uniform pore distribution along with surface acidity of the catalyst. The scope and versatility of the iron ALP as a catalyst in the transesterification is examined by conducting the reaction between DEM with a wide variety of alcohols. The results showed that the structural properties of alcohols along with textural properties of catalyst play an important role in the yield and selectivity of transester products.
Acknowledgment
We thank the Material Research Group, St Joseph's College Research Centre, Bangalore for powder XRD analysis and Bangalore Institute of Technology, Bangalore for providing the BET surface area analysis facilities. We thank Mr. C.U. Anis, SUD-CHEMIE, Cochin for the NH3-TPD analysis. We also thank UGC, New Delhi for providing financial assistance for a minor research project.