1 Introduction
Cordierite (Mg2Al4Si5O18) honeycomb monoliths play a vital role as catalyst carriers in heterogeneous catalysis [1]. Catalyst coated honeycomb monoliths are widely used in automotive catalytic convertors (TWCs), combustion of VOCs, selective reduction of NOx, etc., which involve mainly gas phase reactions [2–9]. The catalyst coated honeycomb monoliths have advantages over powder catalysts, such as formation of a thin layer of catalyst with high active surface area, less amount of catalyst loaded on the monolith is highly effective, easy separation and complete recovery of the catalyst from the reaction mixture [10]. Though cordierite honeycomb monoliths have been used mostly for automotive applications, their use in liquid phase/vapor phase organic synthesis is less explored.
Solid acids as heterogeneous catalysts are extremely useful in many large-volume applications, especially in the production of fine and specialty chemicals [11,12]. Among various solid acid materials, metal oxides and their modified forms, which exhibit exceptional acidic properties, are more interesting with a wide range of applications as catalysts and catalyst supports. Especially among metal oxides, zirconia (ZrO2) a versatile oxide used as a catalyst as well as a catalyst support, is employed in many industrially important organic transformations. Much work has been done on sulfated zirconia as a solid acid in the field of fine chemical synthesis [13,14]. Due to a major disadvantage associated with sulfated zirconia, many efforts have been made to synthesize sulfate free-zirconia based solid acids. Sulfate free-zirconia based solid acids such as MoOx/ZrO2 and WOx/ZrO2 have been synthesized and used as solid acid catalysts in a few liquid and vapor phase reactions [15–17]. It is reported that modification of ZrO2 with Mo or W ions can generate strong acid sites including super acid sites [18]. Though these sulfate free-zirconias are superior solid acid catalysts and free form deactivation, unlike sulfated zirconia, they are less explored for organic synthesis [19].
Salicylates are an important group of esters used in the synthesis of drugs, food preservatives, plasticizers, pharmaceuticals, polyesters, solvents, perfumes and cosmetics [20]. Especially, phenyl salicylate (salol) is an ester, which has a pleasant taste and odor, is more useful in sun-tan lotions, as an antiseptic, anodyne and pain-relieving agent. It can be prepared by simple esterification of salicylic acid with phenol in presence of a liquid/solid acid catalyst. But an anhydrous condition prevalent in transesterification is advantageous in increasing the yield of the desired ester and also in increasing the lifetime of the catalyst system.
In this article, the work done on the synthesis of cordierite honeycomb coated with solid acids such as ZrO2, Mo/ZrO2 and W/ZrO2, determination of their physicochemical properties and catalytic properties in liquid phase synthesis of phenyl salicylate and other salicylate esters via transesterification is reported. The effect of concentration of Mo or W ions on ZrO2, nature of the catalyst (whether honeycomb coated or powder form), reaction temperature, reaction time and reusability of the catalytic material in transesterification is studied to obtain highest possible yield of salol with good selectivity. The use of honeycomb monoliths as catalyst carriers especially for solid acids such as ZrO2 and its modified forms would be a very cost effective and ecofriendly process and also will be very interesting initiative step in the field of organic synthesis.
2 Experimental
2.1 Preparation of the catalytic material
Cordierite honeycomb monoliths (height = 1.20 cm, diameter = 2.50 cm and hole size = 0.2 cm) were used for the present work and they were supplied by Shrey Ceramics, Baroda, India.
Zirconyl nitrate, ammonium molybdate and ammonium tungstate were supplied by M/S LOBA Chemie India Ltd., India.
2.1.1 Synthesis of catalytic material in honeycomb coated form
Solid acids such as ZrO2, Mo/ZrO2 (%Mo = 5, 10, 15) and W/ZrO2 (%W = 5, 10, 15) were coated on different monoliths by impregnation method. The honeycomb was wash coated with zirconia before coating the active catalysts. Wash coating is done to increase the surface area and to have a support material which has a better interaction with the active catalyst [21]. For wash coating, a dilute solution of zirconyl nitrate in presence of a binder (cellulose) was prepared which was then coated on a bare honeycomb monolith by dipping and drying in a furnace maintained at 400 °C.
Typically, for coating 5%Mo/ZrO2 on a honeycomb, dilute solution containing 0.96 g of zirconyl nitrate [ZrO(NO3)3. 8H2O] and 0.25 g of ammonium molybdate [(NH4)6Mo7O24. 4H2O] with 50 mL of deionised water, was prepared. The resulting solution was coated on a wash-coated monolith by dipping and drying in a furnace pre-heated at 400 °C. The dipping and drying steps were repeated for 12 to 15 times until ∼0.2 g of the catalyst is coated on the monolith. The honeycomb coated with 5%Mo/ZrO2 was calcined at 550 °C for 5 h in a muffle furnace before its use as a catalyst. Similarly, 10% and 15%Mo/ZrO2 were synthesized by using the above-described procedure.
Five percent, 10% and 15%W/ZrO2 were coated on a honeycomb by adopting the procedure described in the above paragraph using known amounts of zirconyl nitrate and ammonium tungstate salts.
2.1.2 Synthesis of catalytic material in powder form
ZrO2, Mo/ZrO2 and W/ZrO2 were also prepared in powder forms by impregnation method using salts such as zirconyl nitrate with/without ammonium molybdate or ammonium tungstate. Known amounts of the required salts were taken in a china dish and a paste is made by using small amount of water. The paste was then dried in an air oven at 120 °C for 12 h and calcined at 550 °C for 5 h in a muffle furnace.
2.2 Physicochemical characterization of catalytic materials
The catalytic material (both honeycomb coated and powder forms of ZrO2, Mo/ZrO2 and W/ZrO2) were characterized by BET, NH3-TPD/n-butylamine back titration, powder XRD and SEM techniques for their specific surface area, surface acidity, crystallinity and morphology, respectively.
The specific surface area of the catalytic material was evaluated by a NOVA 1000 Quanta chrome high-speed gas sorption analyzer instrument. In this analysis, the catalytic material was degassed at 523 K for 5 h before measurements. Specific surface area was calculated using the BET equation. The total surface acidity was measured by NH3-TPD method by using Puls Chemi-sorb 2705 from Micromeritics. Here, the catalytic material was degassed under a helium stream for 1 h, ammonia (99.99%) gas was injected into the stream until saturation was reached, cooled to 323 K. The system was maintained at 323 K for 30 min. After purging, the system with flowing helium for 2 h at 323 K, the sample was heated at the rate of 5 K min−1 in helium to 973 K. The change in concentration of the desorbed NH3 was monitored using an online thermal conductivity detector. The total surface acidity was also measured by n-butyl amine back titration method using dry benzene as the solvent and bromothymol blue indicator [22]. The X-ray powder diffraction (PXRD) patterns of the catalytic material were collected on an X-pert Pro Philips diffractometer equipped with Ni filtered Cu-Ka radiation λ = 1.5418 Å using a graphite crystal mono-chromator. The Scanning Electron Micrographs (SEM) were obtained using a JEOL JED-2300 analysis station microscope.
2.3 Catalytic activity studies
Typically, a liquid phase transesterification of methyl salicylate (MS) with phenol (P) was carried out by taking MS: P in 2: 1 ratio. The reactants along with the catalytic material (either honeycomb coated or powder forms of ZrO2, Mo/ZrO2 and W/ZrO2) were placed in the reactor and refluxed for a definite period of time. The total volume of the reaction mixture was always kept constant at 20 mL. After a definite period of time, the reaction mixture was cooled to room temperature, the catalytic material was separated from the reaction mixture, and the products obtained by the transesterification reaction were analyzed quantitatively by gas chromatograph (Mayura) fitted with a (10% SE-30 chromosorb w-AW, 3 m × 1/8″) column coupled with FID detector and qualitatively by GC–MS (Varian).
Similarly, transesterification reactions of methyl salicylate with benzyl alcohol or cyclohexanol were carried out under a definite set of reaction conditions over both honeycomb coated and powder forms of ZrO2, Mo/ZrO2 and W/ZrO2.
3 Results and discussion
The solid acids, ZrO2 or Mo/ZrO2 or W/ZrO2 were coated on different honeycomb monoliths by using impregnation method because it is easy and fast compared to other known techniques. In this article, pure ZrO2, Mo(VI)/ZrO2 and W(VI)/ZrO2 are abbreviated as Z, MZ and WZ respectively.
3.1 Catalyst characterization
3.1.1 BET surface area measurement
BET surface areas of catalytic materials used in the present study are given in Table 1. When the surface areas of bare honeycomb (HC) and honeycomb wash coated with ZrO2 are compared, the latter has higher surface area than the former ones. This indicates that wash coating helps in increasing the surface area of the bare honeycomb. However, surface area of pure ZrO2 (both honeycomb coated and powder forms) was found to be the least among the other catalytic materials. It is observed that when pure ZrO2 was incorporated with either 5%Mo(VI) or 5%W(VI) ions the surface area increased to nearly two fold. But when the concentration of Mo or W ions was increased beyond 5%, not much increase in the surface area of ZrO2 was observed which can be attributed to stabilization effect of ZrO2. A similar trend was observed with the BET surface area of powder form of the catalytic materials.
Surface properties of catalytic material used in the present study.
Sl. No. | Catalytic material | BET surface area (m2/g) | Total surface acidity (mmols/g) |
1 | Z | 42(48) | 0.42(0.40) |
2 | 5%MZ | 99(125) | 1.14(1.13) |
3 | 10%MZ | 97(122) | 1.21(1.20) |
4 | 15%MZ | 96(115) | 1.28(1.24) |
5 | 5%WZ | 92(119) | 1.12(1.11) |
6 | 10%WZ | 89(115) | 1.15(1.16) |
7 | 15%WZ | 85(112) | 1.20(1.19) |
8 | Bare HC | 19 | – |
3.1.2 Surface acidity measurements by NH3-TPD/n-butylamine back titration methods
Among the catalytic materials used in the present work, total surface acidity of pure ZrO2 was found to be the lowest and incorporation of Mo or W ions on ZrO2 increased the total surface acidity of pure ZrO2 drastically which may due to electron deficient states formed by the incorporation of Mo or W cations in to ZrO2 lattice (Table 1). Total surface acidity values obtained by NH3-TPD method and n-butylamine back titration method were found to be almost similar and therefore surface acidity values obtained by the latter method are not shown in Table 1.
However, surface acidic properties of the catalytic material were found to be independent of their forms whether they are in honeycomb coated form or powder form.
3.1.3 Powder XRD studies
Powder XRD (PXRD) patterns of the catalytic materials (honeycomb coated forms only) are shown in Fig. 1. It can be seen in the figure that pure ZrO2 consists of peaks arising from both monoclinic (M) and tetragonal (T) phases (Fig. 1a). But when pure ZrO2 was incorporated with Mo or W ions the intensity of tetragonal phase increased with a decrease in the intensity of the peak corresponding to monoclinic phase. When the concentration of Mo or W ions was increased in the order 5%, 10%, 15%, a gradual increase in the intensity of peaks corresponding to tetragonal phase with decrease in the intensity of the peaks related to monoclinic phase of ZrO2 was observed. This shows that the phase transition of both crystal phases (monoclinic and tetragonal) is dependent on the Mo or W ion concentration. For 10%MZ or 10%WZ, it is interesting to note that PXRD peaks corresponding to monoclinic phase have been totally eliminated, and only peaks from tetragonal phase are seen. However, neither diffraction peaks corresponding to crystalline MoO3 nor peaks corresponding to any new crystalline phases are observed. Further, no diffraction lines corresponding to cordierite honeycomb were detected after the monolith was coated with the catalyst. This shows that the surface of bare honeycomb was fully and effectively covered with the active catalyst. PXRD pattern of bare honeycomb (HC) is also shown in Fig. 1b.
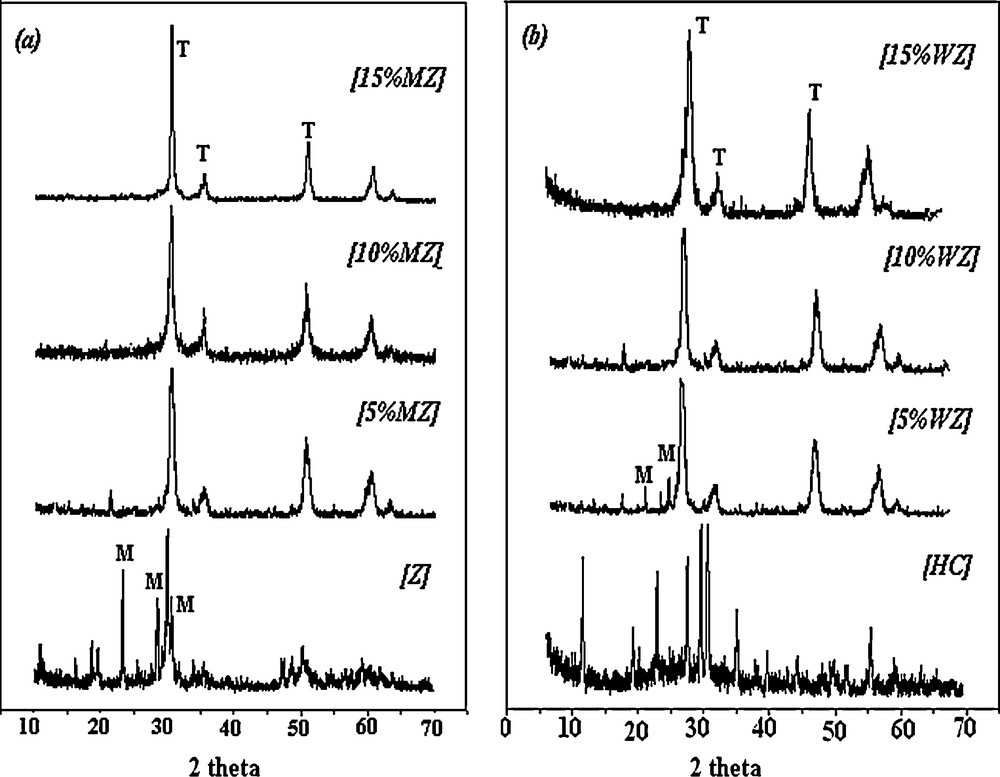
Powder X-ray diffraction (PXRD) patterns of (a) Z and MZ; (b) HC and WZ.
3.1.4 SEM studies
SEM images of honeycomb catalysts are shown in Fig. 2. SEM image of the monolith wash coated with ZrO2 (Fig. 2a) shows flakes which appear more strongly sticking to the surface of the monolith. In Fig. 2b–c, the SEM image of the honeycomb coated with 5%MZ and 5%WZ shows that the coating of the catalyst is considerably uniform. This information obtained from SEM also indicates that the method used for coating the catalytic material was suitable enough to obtain adherent and homogenous coating of the catalytic material.
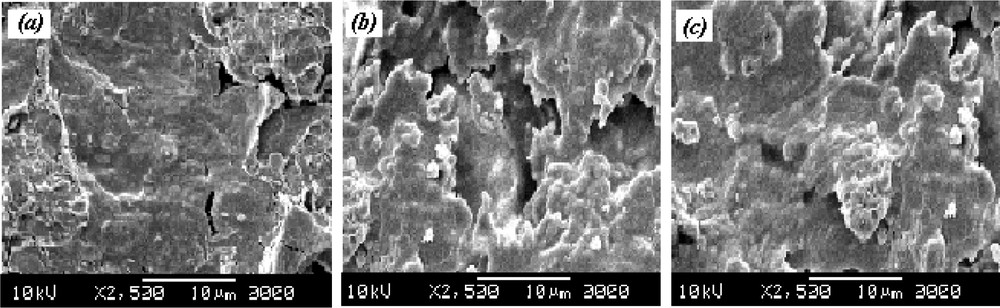
Scanning Electron Micrograph images of (a) honeycomb wash coated with zirconia; (b) honeycomb coated with 5%MZ; (c) honeycomb coated with 5%WZ.
3.2 Catalytic activity
The activity of the catalytic materials such as Z, MZ and WZ (both honeycomb coated and powder forms) was examined in the transesterification reaction of methyl salicylate with phenol.
First of all, when the reaction was carried out without any catalyst, no conversion of phenol into salol was observed. This suggests that transesterification reaction is a catalyzed reaction and a suitable catalyst must be used.
The transesterification reaction was carried out by taking methyl salicylate in excess to make the reaction proceed in forward direction so that a maximum yield of the desired ester is obtained. It has been reported [23] that adsorbed phenol does not react, only poisons the catalytic sites. Therefore, excess of phenol was avoided.
All the catalytic materials used in the present study (both honeycomb coated and powder forms) were found to be active in the transesterification reaction (Fig. 3a–d). The GC and GC–MS analyses showed the presence of only four components, i.e., methyl salicylate, phenol, salol and methanol over Z, 5%MZ, 5%WZ and 10%WZ. But in presence of 10%MZ, 15%MZ as well as 15%WZ, in addition to salol and methanol, diphenyl ether was also formed as the by-product. The catalytic activity of a solid acid catalyst in the formation of a definite product in an organic reaction depends on the acid strength and concentration of active sites present on the solid acid. Formation of diphenyl ether indicates that the catalytic materials 10%MZ, 15%MZ and 15%WZ consisted of very strong (super acidic) sites which are responsible for the dehydration of phenol resulting in the formation of ether. Further, when the selectivity of MZ and WZ towards the formation of diphenyl ether was observed, MZ was found to be more active than WZ indicating the stronger interaction of Mo with ZrO2 when compared to W ions. This strong interaction between Mo and ZrO2 may have resulted in the formation of more number of super acid sites which facilitate dehydration of phenol. The difference in the activity of Z, MZ and WZ in honeycomb coated and powder forms are explained in the next section.
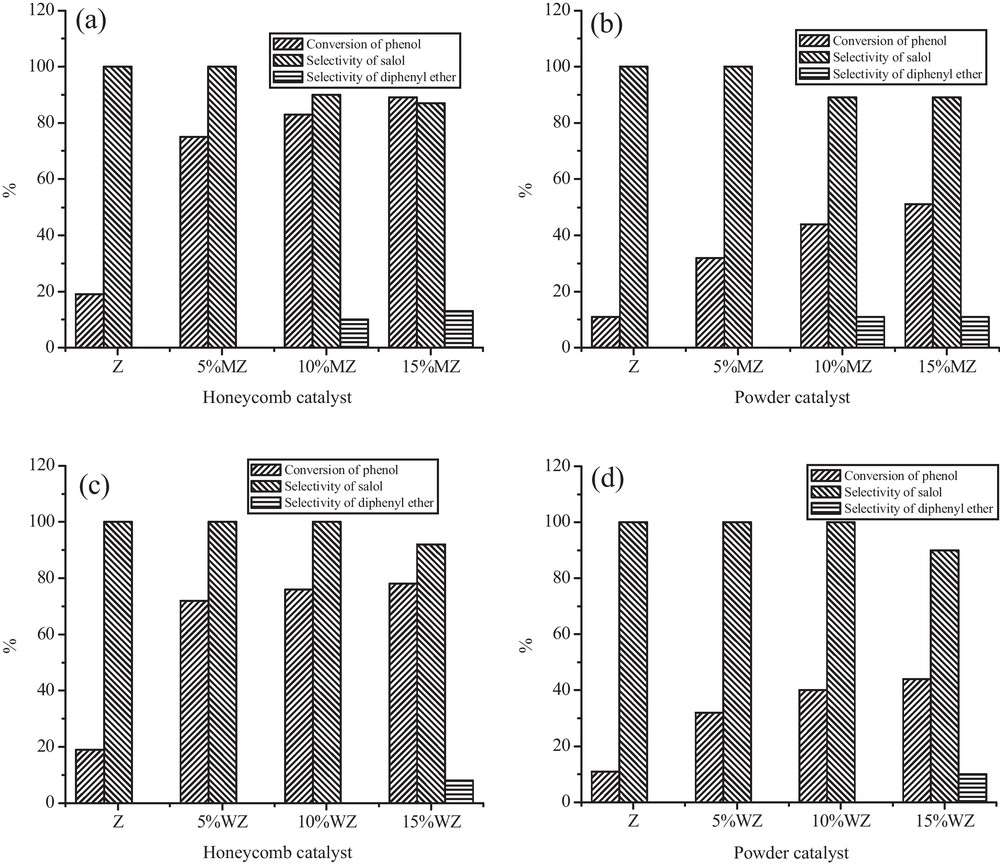
Catalytic activity of the materials used in the present study for transesterification of methyl salicylate with phenol (molar ratio of methyl salicylate: phenol = 2:1; reaction temperature = 130 °C; catalyst weight = ∼0.2 g of Z or MZ or WZ; reaction time = 4 h).
3.2.1 Effect of nature of the catalyst
Catalytic activity of Z, MZ and WZ in their honeycomb coated forms and powder forms were compared in transesterification of methyl salicylate with phenol. Even though same amount of the catalyst (0.2 g) was used in both the forms, a ∼2-fold increase in the conversion (%) of phenol was observed over honeycomb form of the catalyst (Fig. 3a–d). This may be attributed to the availability of more number of active sites on the surface of the honeycomb catalyst due to homogenous dispersion of solid acids which is not possible when used in their powder form. The drastic difference in the activity of the catalytic materials in their honeycomb coated and powder forms can be explained as follows.
The number of channels, their diameter and wall thickness determine the cell density, expressed as cells per square inch (cpsi), which in turn allows the calculation of the geometric surface area; the sum of the areas of all the channel walls upon which the catalyst is deposited. This leads to one of the most important advantages of the honeycomb in that it has a large open frontal area. The lower catalyst loading in case of honeycomb catalyst is compensated by the higher efficiency due to the good mass-transfer characteristics [1]. However, selectivity (%) towards either salol or diphenyl ether remained the same over both the forms of the catalytic material. This also indicates that dispersion of a catalyst over a honeycomb increases the number of active sites (acid sites) should be available for the reaction. But acid site distribution remained almost the same in both the forms.
3.2.2 Effect of reaction temperature
The transesterification of methyl salicylate with phenol was carried out at three different temperatures i.e., 120 °C, 130 °C and 140 °C over honeycomb catalysts such as 5%MZ and 5%WZ to study the effect of reaction temperature on the conversion (%) of phenol and selectivity (%) towards the products i.e., either ester or ether.
The results pertaining to the effect of reaction temperature are presented in the form of bar diagram (Fig. 4). As can be seen, at 140 °C of reaction temperature, diphenyl ether was formed over both 5%MZ and 5%WZ suggesting that, higher reaction temperature also may favor the dehydration of phenol. However, at 120 °C and 130 °C, conversion of phenol increased but the selectivity (%) towards the ester remained 100% over both 5%MZ and 5%WZ.
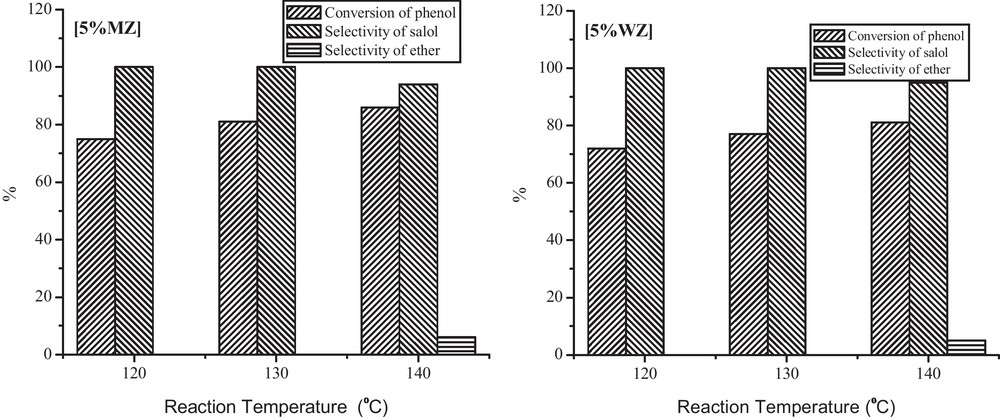
Effect of reaction temperature on the transesterification of methyl salicylate with phenol over honeycomb catalysts (molar ratio of methyl salicylate: alcohol = 2:1; catalyst weight = ∼0.2 g of 5%MZ or 5%WZ coated on the honeycomb; reaction time = 4 h).
3.2.3 Effect of reaction time
The effect of reaction time on the conversion (%) of phenol and selectivity of the products over 5%MZ and 5%WZ was also taken up by varying reaction time from 0.5 to 8 h and the results are presented in the form of a graph as shown in Fig. 5a, b. The conversion (%) of phenol increased rapidly in the beginning (up to 4 h) and gradually leveled off after 4 h. For example, over 5%MZ (honeycomb coated), the conversion increased from 17% in the first 0.5 h to 66% in the next 4 h and the conversion increased only to an extent of 89% in 8 h. However, over 5%MZ (powder), the conversion increased from 6 to 32% in the first 4 h and only 37% in 8 h (Fig. 5a). Selectivity (%) of salol remained 100% up to 6 h over 5%MZ (both honeycomb coated and powder forms) but after 6 h a greasy compound started forming on the surface of the catalyst which could not be separated/detected by GC or G-MS. A similar trend of conversion (%) of phenol and selectivity (%) of salol was observed over 5%WZ catalyst with respect to reaction time (Fig. 5b). Hence, reaction also played a vital role in obtaining high yield of the desired product.
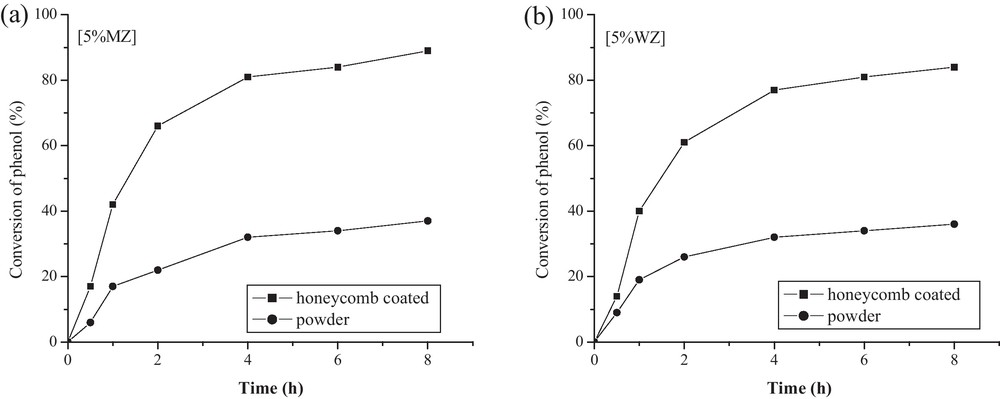
a, b: effect of reaction time on the transesterification of methyl salicylate with phenol (molar ratio of methyl salicylate: alcohol = 2:1; catalyst weight = ∼0.2 g of 5%MZ or 5%WZ; reaction temperature = 130 °C).
3.2.4 Effect of nature of alcohol
The transesterification of methyl salicylate was carried out with benzyl alcohol (BA) or cyclohexanol (CH) and the results of which are presented in Fig. 6 (a–d). The reactions were carried out over all the honeycomb catalysts except over 10%MZ and 10%WZ. At the first instance, Z, 5%MZ, 5%WZ as well as 15%WZ were found to be 100% selective towards the formation of either benzyl salicylate or cyclohexyl salicylate. However, Z showed no conversion of cyclohexanol to either its ester or ether. The nature of alcohol affects the formation of either salicylate ester or ether. Since cyclohexanol is a secondary alcohol it showed less conversion may be due to more steric hindrance when compared to benzyl alcohol which is a primary alcohol. A maximum conversion (8%) was observed over 15%MZ with 98% selectivity towards cyclohexyl salicylate and 2% selectivity towards cyclohexyl ether. Formation of ether suggests that the super acidic sites are also capable of dehydrating a secondary alcohol to produce its corresponding ether to a certain extent.
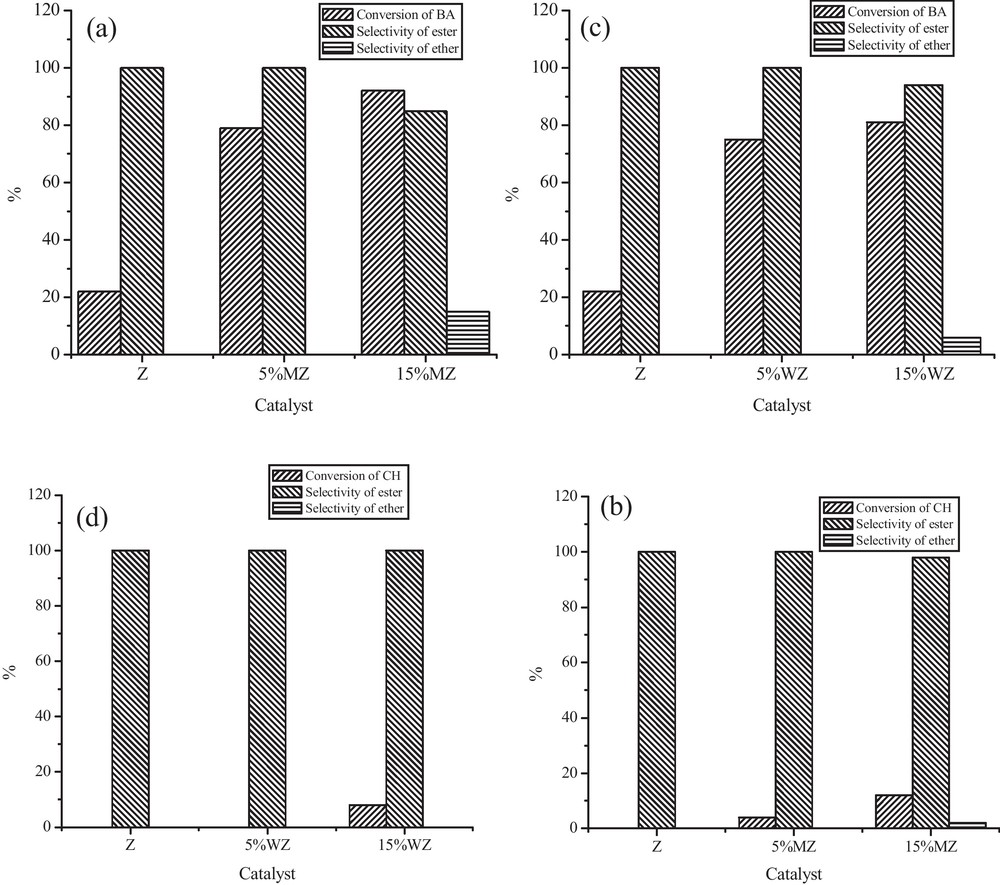
Effect of alcohol in the transesterification of methyl salicylate over honeycomb catalysts (molar ratio of methyl salicylate: alcohol = 2:1; reaction temperature = 130 °C; catalyst weight = ∼0.2 g of Z or MZ or WZ coated on the honeycomb; reaction time = 4 h).
A considerable conversion of benzyl alcohol into benzyl salicylate was observed over 5%MZ and 15%WZ catalytic materials.
On the other hand, Z and 5%WZ showed no conversion of cyclohexanol into either its ester or ether. But over 15%WZ, ∼8% conversion was observed with 100% selectivity towards the ester. Even though 15%WZ consisted of small number of super acidic sites, trace amounts of ether would have formed which was not detectable by the GC.
3.2.5 Effect of reactivation
The honeycomb catalysts used (Z or 5%MZ or 5%WZ) recovered from the reaction mixture were washed with acetone, dried at 120 °C for 2 h, calcined at 550 °C for 1 h, and used in transesterification to study the performance of reactivated catalyst. After reactivation of used honeycomb catalyst, when weight of fresh honeycomb catalyst and reactivated catalyst were compared, no change in weight was observed. This indicates that there is no loss of catalyst from the surface of honeycomb. However, 100% recovery of the catalyst is not possible when used in powder form.
The results are shown in Fig. 7. As can be seen in the figure, no considerable decrease in the conversion (%) of phenol is observed when reused up to four reaction cycles. The reused catalysts were 100% selective towards the formation of only salol. After every reaction cycle, the used honeycomb catalyst was reactivated by using the procedure described above.
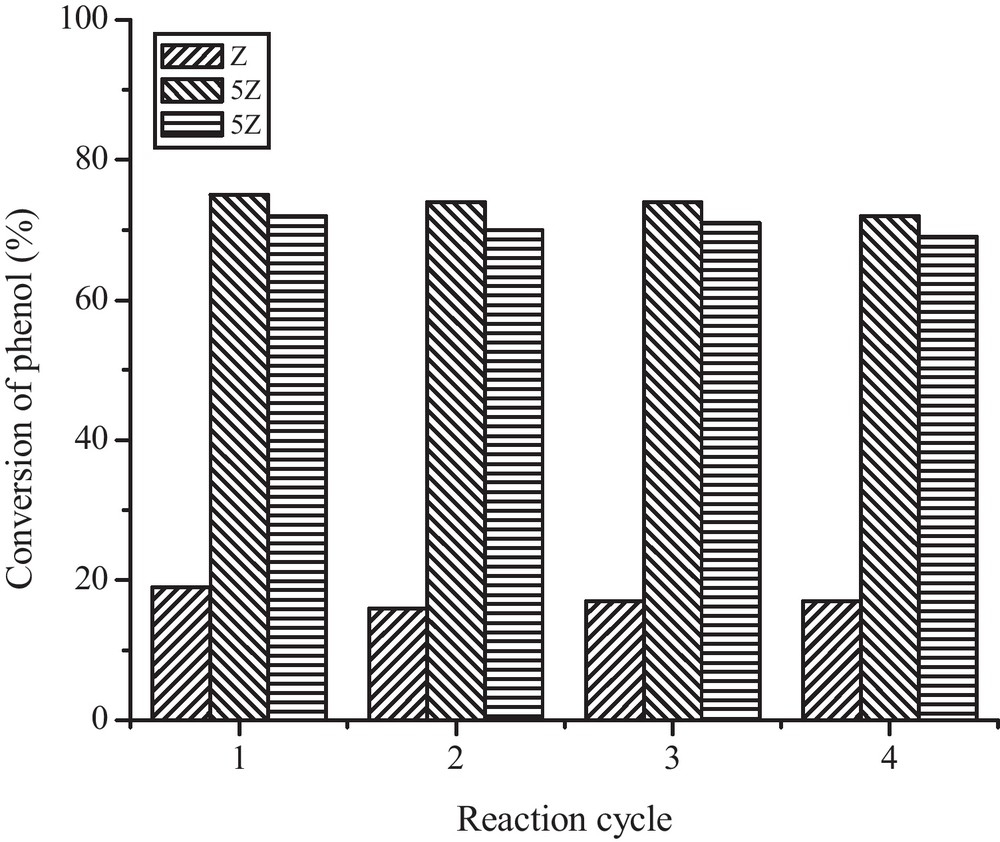
Effect of reusability of honeycomb catalyst in the transesterification of methyl salicylate with phenol (molar ratio of MS:P = 2:1; reaction temperature = 130 °C; catalyst weight = ∼0.2 g of Z or 5%MZ or 5%WZ coated on the honeycomb; reaction time = 4 h).
4 Mechanism of transesterification over a solid acid catalyst
A possible mechanism for the transesterification of adsorbed methyl salicylate and an alcohol (R”OH) over protonic acid sites of a solid acid catalyst is given in Scheme 1.
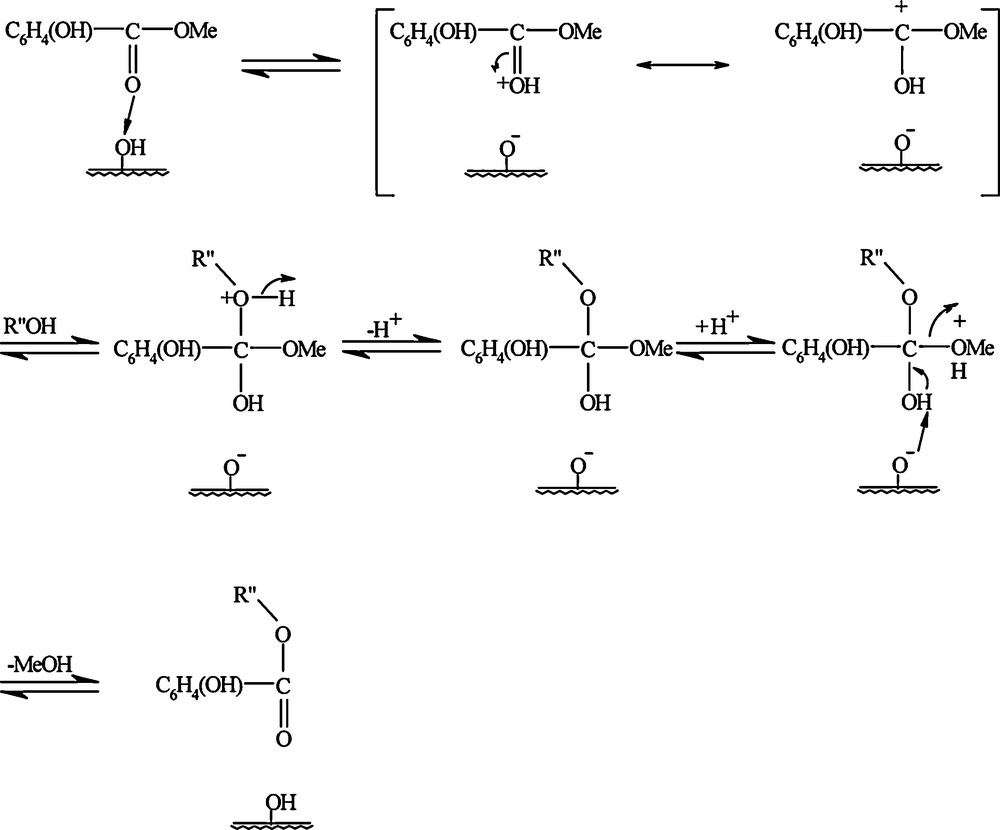
Transesterification of methyl salicylate and an alcohol over solid acids (protonic acid sites).
In the first step, methyl salicylate accepts a proton from the acid site of a solid acid. The second step is the nucleophilic attack of an alcohol molecule to the carbonyl-carbon of methyl salicylate to give an intermediate. In the final step, a proton is lost at one oxygen atom and gained at another to form another intermediate, which further loses a molecule of methanol that gives a salicylate ester. This mechanism is similar to the acid catalyzed Fischer esterification [24].
5 Conclusion
In summary, the honeycomb catalysts are very efficient compared to their powder forms for organic synthesis. These monolithic catalysts are easy to prepare, easy to use, easily recoverable, easily regenerable, reusable and therefore honeycomb catalysts are more advantageous than their powder form. In addition, they are ecofriendly. Further, both Mo(VI)/ZrO2 and W(VI)/ZrO2 coated on honeycomb monoliths were found to be efficient catalytic materials for liquid phase transesterification reactions. Further studies are in progress to use honeycomb coated with solid acids as catalytic materials in vapor phase transesterification. The selectivity towards the desired salicylate ester can be controlled by incorporating suitable amount of Mo or W ions on ZrO2. Increasing the concentration of Mo or W ions may result in the increase in concentration of super acid sites which are responsible for the formation of ether due to dehydration of alcohol used in transesterification reaction.
Acknowledgement
The authors, especially Dr. S.Z.M., thank DST, New Delhi for providing financial assistance through a major research project. Authors are thankful to the authorities of IITM for providing BET surface area and NH3-TPD analysis, Materials Research Group, St. Joseph's College, Bangalore for XRD analysis and IISc, Bangalore for GC-MS analysis.