1 Introduction
The esterification reaction is an extremely slow reaction and needs the addition of a catalyst in order to obtain high actual yields. The most common catalysts used for an esterification reaction are strong mineral acids such as sulfuric and hydrochloric acids as well as sulfonic acids [1]. Their catalytic activity is high, but they have several disadvantages, such as their corrosive nature, the existence of side reactions and they are often hardly separated from the reaction mixture. The use of solid acid catalysts offers an alternative as they can be easily separated from the liquid reaction mixture by filtration or decantation and, thus, reused, they have high selectivity and, last but not least, they are environmentally friendly. The solid catalysts mostly used in esterification reactions are classical solid acids including ion-exchange resins [2–6], zeolites [7–9] and superacids like sulphated zirconia [10]. The commercially available solid acid catalysts have recently been comparatively studied in the esterification of acetic acid with butanol [11].
It is well known [12] that oxides of elements with valence five or higher, such as tungsta, molybdena, vanadia, phosphoric anhydride and niobia, present strong to very strong Brønsted acidity which make them good candidates as acid catalysts. Some of them have already been studied as catalysts for esterification [13–17] and transesterification [18–20] reactions. Thus, Nb2O5/SiO2–Al2O3 has been studied as catalyst for the esterification reaction of acetic acid with ethanol, n-Butanol and iso-pentanol showing conversions of 83, 87 and 91%, respectively, and 100% selectivity for esters (8 h reaction time) [13]. WO3/USY showed good catalytic performances (conversion above 74% at 200 °C and reaction time 2 h) in the esterification of oleic acid with ethanol [14], and WO3/ZrO2 was an effective catalyst in the esterification of the free fatty acids from dark oil to fatty acid methyl esters with conversion of 96% at 150 °C (2 h reaction time) [15]. It has recently been shown that MoO3 supported on γ-alumina is an efficient (81% conversion at 100 °C and 2 h reaction time) and stable solid acid catalyst for the esterification of acetic acid with n-Butanol [16], and MoO3 supported onto a thin alumina film has found to give 62% conversion and 100% selectivity for ester in the esterification reaction of acetic acid with ethanol [17]. Unsupported and silica-supported V2O5 catalysts have been tested in the transesterification reaction of dimethyl oxalate with phenol, the unsupported system showing relatively good performances (35% dimethyl oxalate conversion and total selectivities to methyl phenyl oxalate and diphenyl oxalate exceeding 75%) [18], but, to the best of our knowledge, there are no studies investigating alumina-supported V2O5 as catalysts for the esterification reaction in general and for the esterification of acetic acid with n-Butanol in particular. The reaction product, butyl acetate as the most of alkyl acetates is generally used as solvent, as component in flavoring and as additive in perfumes [1].
In the present work, the catalytic properties of vanadium oxides supported on γ-alumina in the esterification of n-Butanol with acetic acid have been for the first time studied and compared with those of γ-alumina support. The effects of the reaction time, the molar ratio between the reactants, the speed of agitation and the catalyst loading on the reaction were investigated.
2 Experimental
2.1 Catalyst preparation and characterization
Al2O3 support was prepared from Al(NO3)3·9H2O (Fluka Analytical) by precipitation with ammonium carbonate (Lachema) at controlled pH of 6.5. V2O5 was introduced at two concentrations, 5% and 10% by weight, via incipient wetness impregnation of the alumina support with aqueous NH4VO3 (Fluka Analytical) solutions containing appropriate amounts of vanadium. After impregnation, the samples were dried in air at 100 °C and then calcined at 600 °C for 4 h. The 5 wt % V2O5/Al2O3 and 10 wt % V2O5/Al2O3 samples were labelled 5V-Al2O3 and 10V-Al2O3, respectively.
The crystalline phases were investigated by the X-ray diffraction (XRD) method. XRD patterns were obtained with a Philips PW 3710 type diffractometer equipped with a CuKα source (λ = 1.54 Å), operating at 50 kV and 40 mA. They were recorded over the 10–70° angular range with 0.02° (2θ) steps and an acquisition time of 1 s per point. Data collection and evaluation were performed with PC-APD 3.6 and PC-Identify 1.0 software.
Surface areas of the catalysts were measured from the adsorption isotherms of nitrogen at –196 °C using the BET method with a Micromeritics ASAP 2020 sorptometer.
Qualitative and quantitative electron probe microanalyses were performed using a Philips XL 30 ESEM (Environmental Scanning Electron Microscope) having EDX (Energy Dispersive X-ray) analyzer. An accelerating voltage of 20 kV was used. The powder samples were fixed on a holder and sample compartment was evacuated in order to prevent the electrical charging. Electron beams were very finely focused. Therefore, elemental analysis of very small area on specimen surface was done in so-called spot mode. Simultaneously, the surface of the sample was photographed, the micrographs obtained being presented in Fig. S1.
The acidity of the catalysts was estimated by temperature-programmed desorption of ammonia (NH3-TPD). About 0.1 g of the catalyst sample was dehydrated at 500 °C in dry air for 1 h and purged with N2 for 0.5 h. The sample was then cooled down to 100 °C under the flow of N2, and NH3 was supplied to the sample until its saturation. For desorption of the physisorbed ammonia, a nitrogen stream was passed over the sample, at the same temperature, until no more NH3 was observed in the exit flow. Finally, the chemisorbed NH3 was desorbed in a N2 flow by increasing the temperature successively up to 350 °C and 500 °C with a heating rate of 10 °C/min. The ammonia desorbed was bubbled through a solution of sulfuric acid. The acid in excess was titrated with a solution of NaOH, the amount of ammonia desorbed being then calculated. The ammonia desorbed at temperatures lower than 350 °C accounted for the weak and medium-strength acid sites while that desorbed in the temperature range from 350 to 500 °C, for the strong acid sites.
Characterization of the catalysts samples has been performed before and, for some of them, after the catalytic test.
2.2 Esterification reactions
The esterification reactions of acetic acid (Chimactiv, 99.5%) with n-Butanol (Riedel-de Haën, 99.5%) were performed in a 150 mL two-neck flask equipped with a condenser and an additional port for sample withdrawal. The above assembly was heated using a thermostated hotplate. The reaction was carried out at 100 °C with a molar quantity of acetic acid of 0.09 and an n-Butanol-to-acetic acid molar ratio varied from 1 to 3. Cyclohexane (Riedel-de Haën, 99.5%) was always added to the reaction mixture for water removal, the cyclohexane-to-acetic acid molar ratio being kept at 1. The amount of catalyst was varied between 0.5 and 1.0% of the mass of mixture charge in the reaction and the reaction time varied between 30 and 120 min. Unless specified, all experiments were conducted at a speed of agitation of 600 rpm. All the catalysts used in the reaction were in the powder form. Samples from the organic layer were withdrawn at regular intervals and analyzed with a Thermo Finnigan chromatograph using a DB-5 column and a flame ionization detector. Under the employed conditions of reaction, both in the presence and in the absence of a catalyst, butyl acetate was the only product detected. The mass balances, calculated after a reaction time of 120 min, were always higher than 94%.
3 Results and discussion
3.1 Catalysts characterization
Table 1 lists the BET surface areas of the catalysts. It can be seen that the surface area of the alumina support was higher then that of the supported catalysts and for the latter, as expected, the surface area decreased with increasing vanadia loading. This has already been observed for alumina-supported vanadia [21] and may be due to the blockage of pores of alumina by vanadia with increasing its loading.
Specific surface areas and chemical compositions of the catalysts.
Catalyst | BET surface area (m2/g) | Chemical composition (wt. %) obtained by EDX | |||||
Before the catalytic test | After the catalytic testa | ||||||
V | Al | O | V | Al | O | ||
Al2O3 | 227 | – | – | – | – | – | – |
5V-Al2O3 | 178 | 15.30 | 45.58 | 39.12 | 6.49 | 50.72 | 42.79 |
10V-Al2O3 | 147 | 20.75 | 40.34 | 38.91 | 8.32 | 50.55 | 41.13 |
a Reaction conditions: 0.7 wt % catalyst, temperature 100 °C, n-Butanol-to-acetic acid molar ratio = 3:1 and reaction time 120 min.
The XRD patterns of the alumina support and of the supported vanadia catalysts are presented in Fig. 1. Only broad lines corresponding to γ-alumina (PDF 10-425) were observed in the case of γ-alumina support and in the case of 5V-Al2O3 sample suggesting that vanadia is relatively well dispersed on the alumina support in the latter case, while crystalline V2O5 (PDF 41-1426) is evident in the case of 10V-Al2O3 sample. This is in line with the observed decrease in the surface area with increasing the vanadia loading.
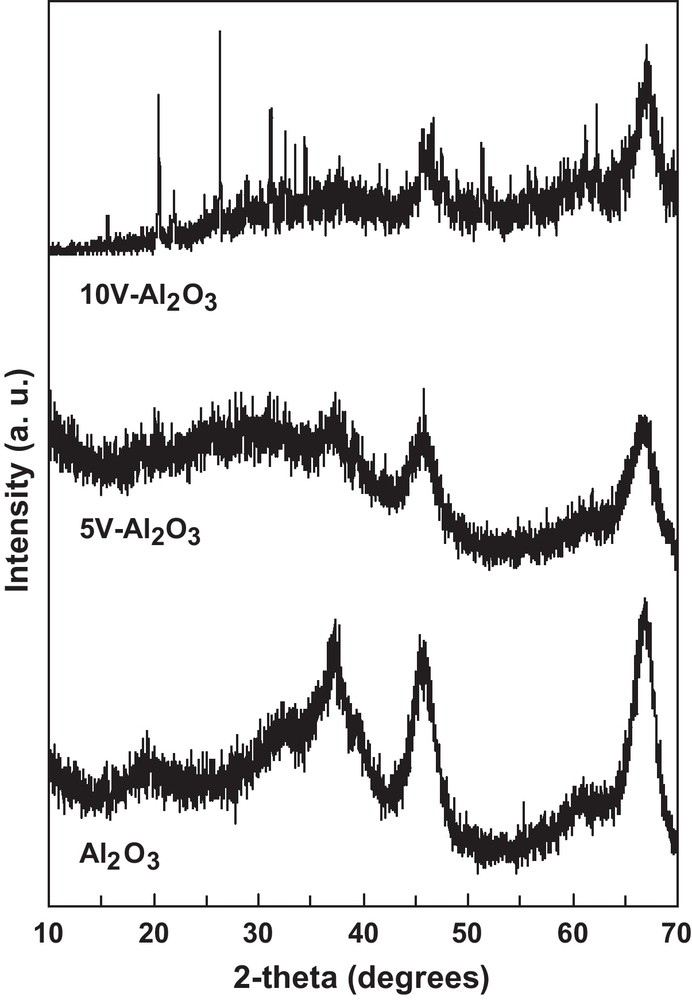
X-ray diffraction patterns of the alumina support and of the alumina-supported V2O5 catalysts.
The chemical compositions of the vanadium-containing samples before and after the catalytic test, determined by EDX analysis, are reported in Table 1. They showed that the vanadium content is much higher than the nominal value for both samples. This is not surprising because the EDX analysis give, in the conditions used, the surface and subsurface rather than bulk composition of the solids. On the other hand, it can be observed that the vanadium content strongly decreased after the catalytic test for both samples, suggesting the loss of vanadium during the catalytic reaction.
A key point to understand the catalytic behavior in the esterification reaction is to investigate the acidity of the catalyst. This was achieved using the NH3-TPD technique. The total acidities of the catalysts, expressed as the total number of acid sites per gram of catalyst, determined by NH3-TPD, are presented in Table 2. It can be observed that the total acidity increased by adding vanadia to alumina and by increasing its content following the order: Al2O3 < 5V-Al2O3 < 10V-Al2O3. It can also be observed that the number of weak and medium-strength acid sites decreased while the number of strong acid sites increased by adding vanadia to alumina and by increasing its content. This behavior has already been observed for alumina-supported vanadia systems [22]. Note that, at similar transition-metal oxide loadings, the total number of acid sites was twice lower while their strength was higher for supported vanadia than for supported molibdena catalysts [16].
Number and strength of acid sites of the catalysts.
Catalyst | Number of acid sites (mmol/g) | Total number | ||
Weak and medium | Strong | (mmol/g) | 104 (mmol/m2) | |
Al2O3 | 0.097 | 0.027 | 0.124 | 5.46 |
5V-Al2O3 | 0.076 | 0.094 | 0.170 | 11.56 |
10V-Al2O3 | 0.068 | 0.182 | 0.250 | 14.04 |
10V-Al2O3 useda | 0.060 | 0.150 | 0.210 | – |
a Reaction conditions: 0.7 wt % catalyst, temperature 100 °C, n-Butanol-to-acetic acid molar ratio = 3:1 and reaction time 120 min.
The acidity of the 10V-Al2O3 sample has also been determined after the catalytic test (Table 2), a decrease of the total number of the acid sites being observed. This is in line with the loss of vanadium observed by EDX chemical analysis.
3.2 Catalytic activity
Some factors such as reaction time, acid to alcohol mole ratio and catalyst amount influence the conversion of acetic acid in the esterification reaction of acetic acid with n-Butanol. These influences are presented below.
3.2.1 Influence of the reaction time
The influence of the reaction time was studied in the following reaction conditions: 8 mL of n-Butanol, 5 mL of acetic acid (n-Butanol-to-acetic acid mole ratio 1:1) and 10 mL of cyclohexane; the catalyst represents 0.7% of the mass of mixture charge in the reaction; the reaction temperature was kept at 100 °C. In these conditions, the selectivity of n-butyl acetate was, in all cases, 100%. This means that the conversion of acetic acid can represent the yield of n-butyl acetate. The conversion of acetic acid measured in these reaction conditions as a function of the reaction time over Al2O3, 5V-Al2O3 and 10V-Al2O3 catalysts as well as in the absence of a catalyst is shown in Fig. 2a. As expected, the conversion of acetic acid was higher in the presence of a catalyst than without a catalyst and increased remarkably with the prolonging of the reaction time. The activities at 120 min of reaction time are compared in the following. As known, esterification is a typical acid catalyzed reaction, and the acidity of the catalyst would exert a great influence on the catalytic activity. The highest conversion (62.6%) of acetic acid over 10V-Al2O3 catalyst may thus be due to its higher acid site density and strength (Table 2) compared with 5V-Al2O3 (conversion 38.7%) and Al2O3 (conversion 32.2%) catalysts that have both lower acid site densities and strengths. The catalytic performances of the V2O5/Al2O3 catalysts were comparable with those of the MoO3/Al2O3 catalysts with similar transition-metal loadings and measured in similar reaction conditions [16]. With increasing the reaction time from 120 to 210 min for 10V-Al2O3 catalyst, the conversion of acetic acid increased from 62.6 to 87.7% without a change in the selectivity for n-butyl acetate (Fig. 2b).
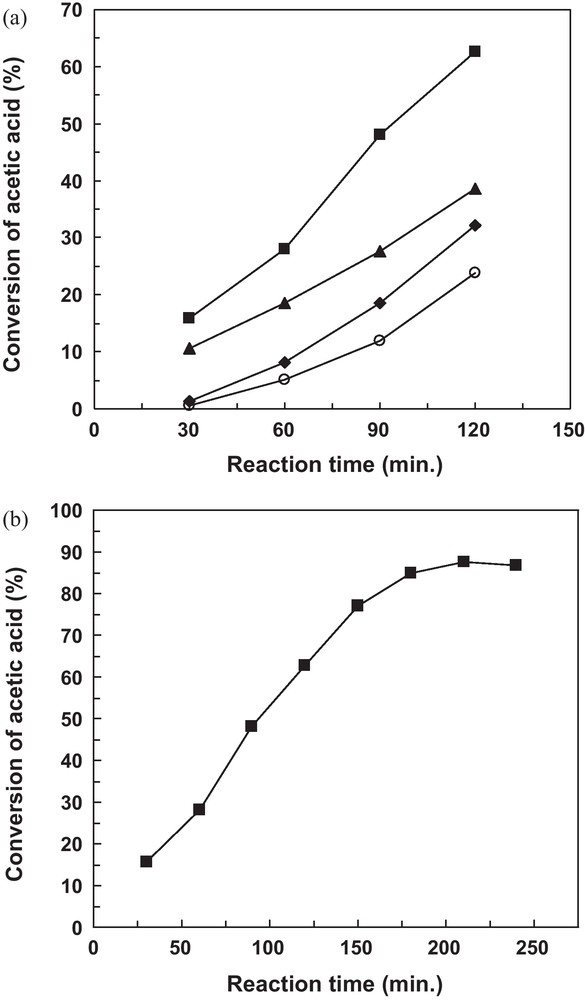
Conversion of acetic acid as a function of the reaction time in the catalytic and non-catalytic esterification reaction at 120 min (a) and 240 min (b). Reaction conditions: n-Butanol-to-acetic acid molar ratio = 1:1, reaction temperature 100 °C, 0.7 wt % catalyst (–non-catalytic reaction, –Al2O3, –5V-Al2O3, –10V-Al2O3).
3.2.2 Influence of the molar ratio of reactants
The effects of different feed ratio on acetic acid conversion over 5V-Al2O3 and 10V-Al2O3 catalysts were studied and the results are presented in Fig. 3. By increasing the n-Butanol-to-acetic acid mole ratio from 1:1 to 2:1 the conversion increased, as expected, for both catalysts for all the reaction times considered. Nevertheless, by further increasing the mole ratio to 3:1, a decrease in conversion was observed probably due to the leaching of vanadia from alumina support in line with the observed loss of vanadium (Table 1) and decrease of the number of acid sites (Table 2) of the catalyst after the catalytic test. This phenomenon has already been observed during the esterification of acetic acid with n-Butanol on montmorillonite-supported dodecatungstophosphoric acid catalyst [23]. Note that the selectivity for n-butyl acetate was, in all cases, 100% and the conversion of acetic acid reached 66.4% in the presence of 10V-Al2O3 catalyst for an n-Butanol-to-acetic acid mole ratio equal to 2:1 and a reaction time of 120 min.
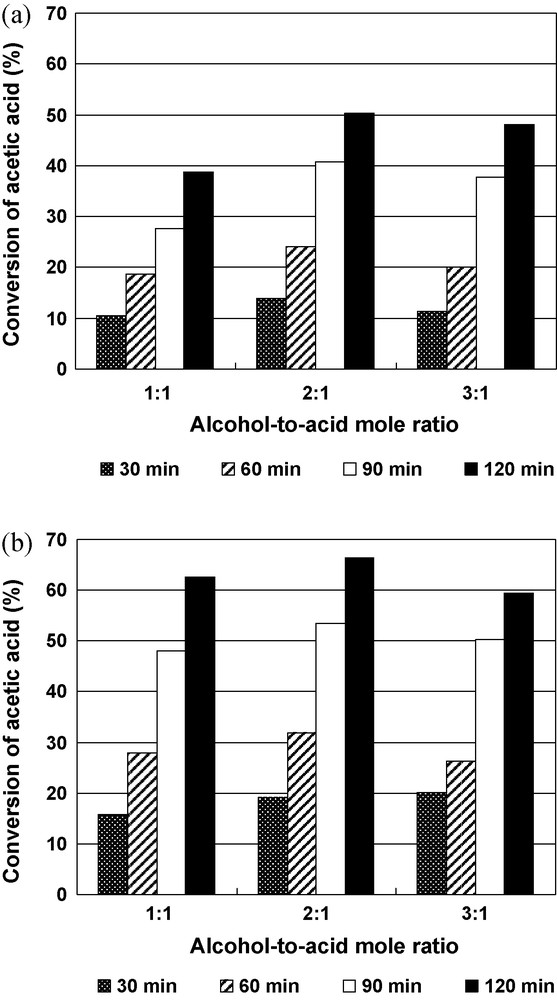
Effect of mole ratio on the esterification of acetic acid with n-Butanol using 5V-Al2O3 (a) and 10V–Al2O3 (b) as catalysts. Reaction conditions: temperature 100 °C, 0.7 wt % catalyst.
3.2.3 Influence of the catalyst loading
The effect of catalyst loading on the acetic acid conversion was studied for the vanadia-supported catalysts at 0.5, 0.7 and 1% by mass of the total reaction mixture, n-Butanol-to-acetic acid mole ratio equal to 2:1 and reaction times of 60 and 120 min. The results are presented in Fig. 4. In all cases, a significant increase of the conversion of acetic acid with increasing the catalyst loading from 0.5 to 0.7% was observed, while the conversion tends to a plateau for catalyst loadings higher than 0.7% suggesting that this is the optimal mass fraction of the catalyst in the reaction medium.
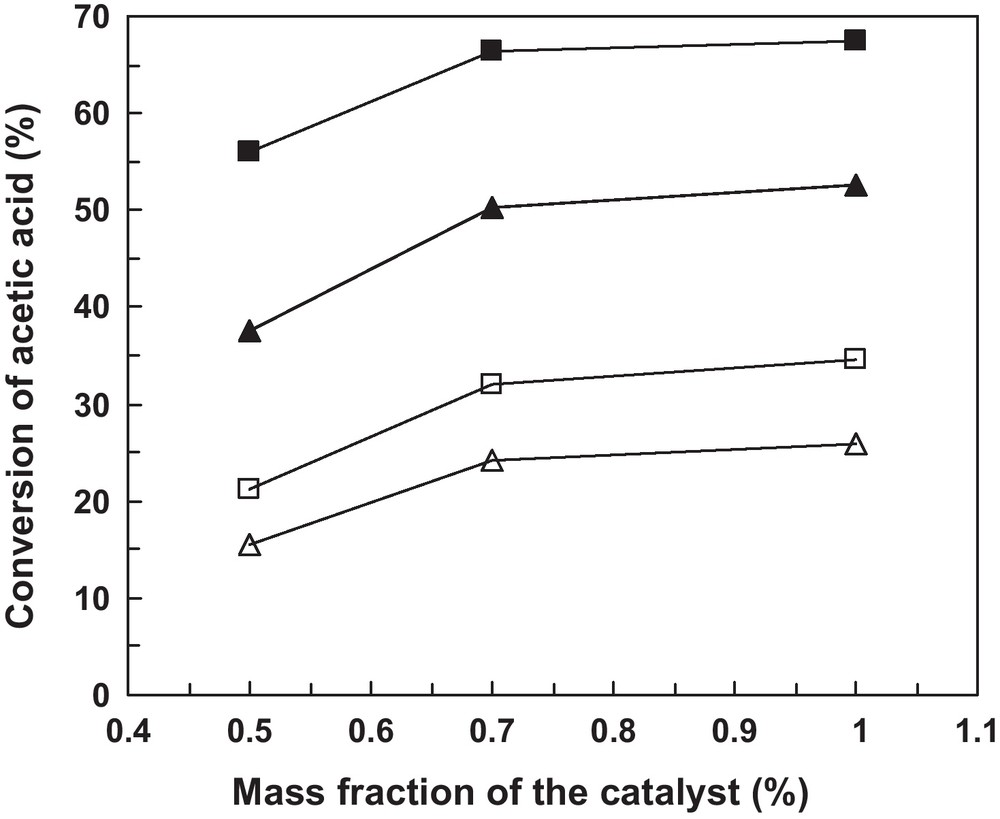
Effect of catalyst concentration on the esterification of acetic acid with n-Butanol using 5V-Al2O3 (, ) and 10V-Al2O3 (, ) as catalysts. Reaction conditions: temperature 100 °C, n-Butanol-to-acetic acid molar ratio = 2:1 and reaction time 60 min (,) and 120 min (, ).
3.2.4 Influence of the speed of agitation
The influence of the speed of agitation on the conversion of acetic acid was carried out for 10V-Al2O3 catalyst samples at three different speeds, i.e. 450, 600 and 750 rpm, to examine the influence of a possible external mass transfer effect under the following reaction conditions: n-Butanol-to-acetic acid mole ratio was 2:1, 0.7 wt % catalyst, reaction temperature 100 °C and reaction times 60 and 120 min. The results obtained are shown in the Fig. 5. It is clear from the figure that there was no effect of the speed of agitation in the range 450–750 rpm on the conversion of acetic acid. This implies that there was no resistance to mass transfer of acid to the external surface of the catalyst.
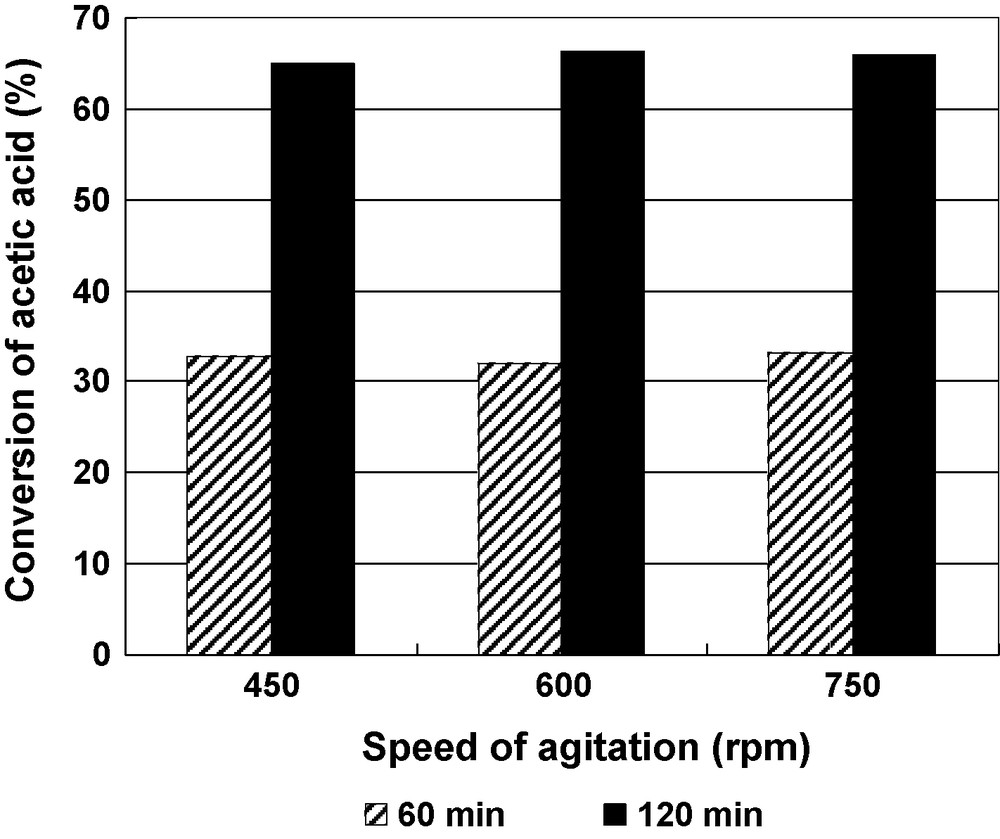
Effect of the speed of agitation on the conversion of acetic acid for 10V-Al2O3 catalyst at 60 min and 120 min reaction time. Reaction conditions: n-Butanol-to-acetic acid molar ratio = 2:1, reaction temperature 100 °C, 0.7 wt % catalyst.
3.2.5 Reusability of the catalyst
In order to know if the catalysts lose their catalytic activity during the reaction, the 10V-Al2O3 catalyst was recovered and reused as catalyst in the esterification reactions under the following reaction conditions: n-Butanol-to-acetic acid mole ratio was 1:1, 0.7 wt % catalyst, reaction temperature 100 °C. After the first catalytic test (2 h reaction time), the catalyst was filtered, washed with distilled water several times, dried at 120 °C in air and then used in the esterification reaction with a fresh reaction mixture in a second test. The procedure was repeated in a third reaction test. The results obtained, presented in Fig. 6, show a loss of activity of the 10V-Al2O3 catalyst with its subsequent reuse. Thus, for a reaction time of 2 h the conversion of 62.6% in the first run decreased to 52.0% in the second run and to 47.6% in the third run. This decrease in catalytic activity is probably due to the subsequent leaching of vanadia from the alumina support into the liquid phase during the catalytic reactions as suggested by the EDX chemical analysis of the catalysts after the catalytic test (Table 1). Note that the selectivity for n-butyl acetate remained 100%.
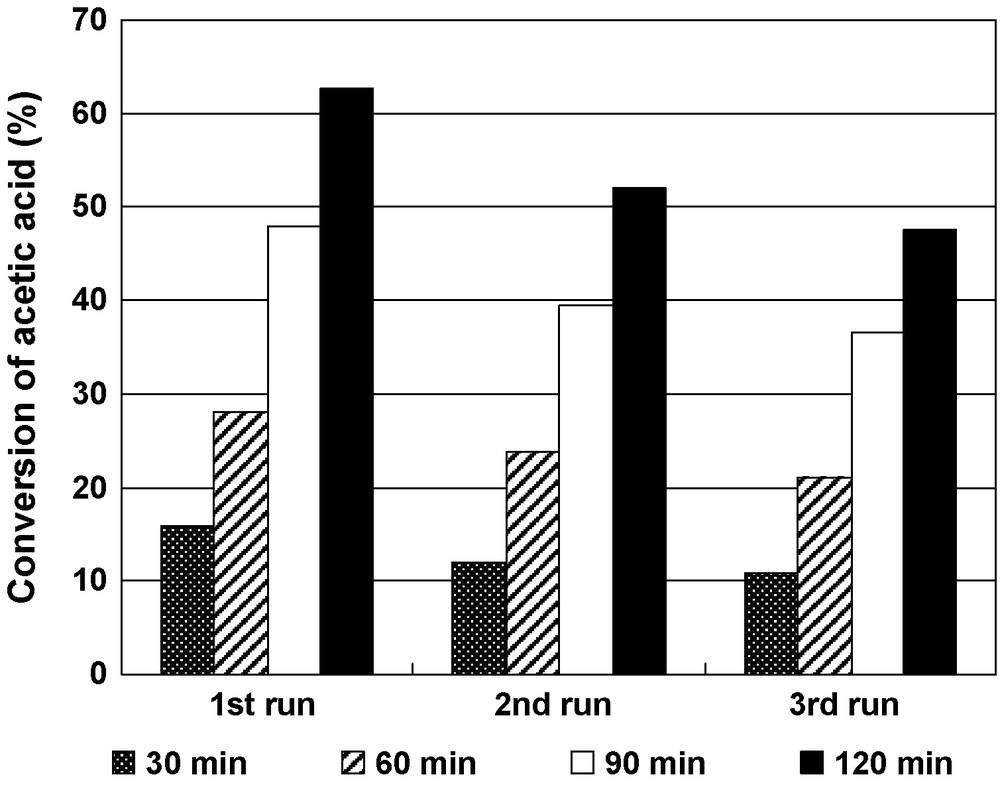
Reusability of the 10V-Al2O3 catalyst. Reaction conditions: n-Butanol-to-acetic acid molar ratio = 1:1, reaction temperature 100 °C, 0.7 wt % catalyst.
4 Conclusions
The present work demonstrates that vanadium oxides supported on γ-alumina containing well dispersed VOx species on the alumina support act as relatively efficient solid acid catalysts for the esterification of acetic acid with n-Butanol. In all the esterification reactions the selectivity for butyl acetate was 100%. The conversion increased in the following order: uncatalytic < Al2O3 < 5V-Al2O3 < 10V-Al2O3 in line with the total acid site density and strength measured by NH3-TPD. There was no resistance to mass transfer of acid to the external surface of the catalyst and the optimal catalyst loading in the reaction medium was around 0.7%. Although the selectivity for butyl acetate remained 100%, the catalytic activity of the 10V-Al2O3 catalyst decreased after three successive reactions because of the leaching of vanadia from the alumina support.
Acknowledgements
The authors are grateful to Mr. Oravetz Dezsö for technical assistance with scanning electron micrographs recording and EDX analysis.