1 Introduction
Today, one of the ultimate goals and challenges in modern chemistry is to develop highly efficient transformations for the preparation of biologically active and many other functional materials from small and commercially compounds with potential application in the pharmaceutical or agrochemical industries. In response to these, there is a need for synthetic chemists to find new, strategically important processes which are environmentally cleaner and more efficient and lead to greater structural variation in a shorter period of time with high simple work up and high yields and purity [1]. For these reasons, over the last few years, enormous advances have been made in chemical processes and products to achieve elegant solutions with the ultimate goal of hazard-free, waste-free, energy-efficient synthesis and application of non-toxic products without sacrificing efficacy of function. On the other hand, most of the chemical manufacturing industries and chemistry laboratory use electricity generated from coal for heating purposes and organic solvent, which are responsible for environmental pollution. In view of this, attempts have been made to use alternate energy sources and green reaction media in organic synthesis and industry. In this context, ultrasound may offer environmentally clean technology by improving product yields and selectivities, enhancing product recovery and minimizing the production of waste at source [2–6].
Epoxides are very useful intermediates and starting materials in organic synthesis due to their versatility and reactivity with a wide range of nucleophiles. The catalytic, simple ring opening of epoxides is an efficient means to convert readily available, inexpensive bulk chemicals into 1,2-difunctionalized fine chemicals in industry and laboratory [7,8]. Nucleophilic opening of epoxide rings by in situ generated dithiocarbamic acid is an important reaction for synthetic organic/medicinal chemists as the resultant 2-hydroxydithiocarbamates compounds have potential medicinal, anti-microbial and agricultural uses [9,10]. Therefore, the syntheses of biologically important 2-hydroxydithiocarbamates have received considerable attention, and there are some reports for the synthesis of dithiocarbamate derivatives in the literature [11–16]. However, there are various limitations such as long reaction times, use of organic solvents, high temperatures, moderate yields and limited substrate. Given the widespread availability of amines, carbon disulfide and epoxides, there is a substantial interest in developing efficient one-pot three-component reaction from these simple starting materials. Thus, several efforts have been made to develop green simpler methodologies for a one-pot reaction of amines with carbon disulfide and epoxide in novel reaction media such as water and ionic liquids [17–23].
2 Results and discussion
Considering the well-established applications of ultrasound activation to promote a variety of chemical reactions and as part of an ongoing program for development of efficient protocols for the synthesis of dithiocarbamate from simple starting material in water [24–27]. Herein, we report the extension of this methodology to the ultrasound-promoted one-pot three-component synthesis of 2-hydroxy dithiocarbamate in green reaction media with a view to explore the yields and rate of reaction. Our initial study focused on the development of the optimal reaction conditions for this transformation. Therefore, pyrrolidine, carbon disulfide and phenyl glycidyl ether were chosen to optimize the molar ratio of reagent and volume of water and ultrasound power. First, in order to verify the effect of ultrasound irradiation in different green solvents, we have performed the one-pot reaction of pyrrolidine (2 mmol), carbon disulfide (3 mmol) and phenyl glycidyl ether (2 mmol) in water (3 mL), choline chloride-based deep eutectic solvent (3 mL) and polyethylene glycol (3 mL); under ultrasound irradiation with sonotrode LS24d5 the reaction can be completed within 7 min in 95%, 80%, 82% yield respectively, (Scheme 1). Because of low cost, high yields and simple reaction in water, the further reaction was carried out in water.

Optimization of reaction condition.
We also monitored the effect of different output powers and sonication times on the reaction. Under 50%, 60%, and 80% of the output power (amplitude) the yield of dithiocarbamate was 60%, 80%, and 95% respectively. It seems that the higher amplitude of ultrasound irradiation can improve the yield of dithiocarbamates. We also carried out the indirect sonication of reaction in Eppendorf vials, with the frequency 24 kHz with the 80% of the output power, and the corresponding 2-hydroxy dithiocarbamate 3 was obtained in 60% yields for 10 min. Ultrasound mediated one-pot three-component synthesis of dithiocarbamate in an organic solvent such as acetonitrile, dichloromethane, methanol, toluene and tetrahydrofurane also gave the desired product in good yield. Among screened solvent systems, water and methanol were the solvent of choice, in which the one-pot reactions proceeded smoothly and afforded the desired adducts in high yields at short reaction times. However, separation of products from the solvent and inherent toxicity of methanol are major problems and consequently we conclude that water is an ideal solvent for this reaction.
Under optimized reaction condition, to explore the scope of this new three-component coupling, we investigated different amines and epoxides and the results of this investigation are shown in Table 1. This procedure is quite general, and a wide range of structurally varied amines such as primary, allylic, benzylic, hindered and unhindered secondary amines were used in this protocol with excellent results. Primary amines such as benzylamine and cyclohexylamine, sec-butylamine and hindered amine such as isopropylamine undergo efficient ring opening reaction with epoxides to give the corresponding 2-hydroxy dithiocarbamate with excellent results (Table 1). Furthermore, secondary amines such as pyrrolidine, piperidine, dibutylamine and diethylamine show higher yields of products under ultrasound irradiation. With regard to epoxides, the reactions proceeded smoothly with the commercially available epoxides such as glycidyl phenyl ether, allyl glycidyl ether, isopropyl glycidyl ether, propylene oxide, butane oxide, cyclohexene oxide and styrene oxide. The results indicate the usefulness of this method. The substituents do not show any significant effects on the reaction times and the yields of the products. The cleavages were regio- and stereoselective and can be explained by the steric and electronic factors associated with the epoxide and the dithiocarbamate anion. The major isomer in all these reactions with alkyl- and aryl-substituted epoxides was the secondary alcohol, which resulted from the usual nucleophilic attack of bulky dithiocarbamate anions at the less-substituted carbon atom of epoxides in high to quantitative yields.
One-pot synthesis of 2-hydroxy dithiocarbamate in the presence of ultrasound.
Entry | Epoxide | Amine (RR′NH) | Yield (%)a |
1 | 3a | 97 | |
2 | 3b | 92 | |
3 | 3c | 95 | |
4 | 3d | 78 | |
5 | 3e | 90 | |
6 | 3f | 80 | |
7 | 3g | 76 | |
8 | 3h | 72 | |
9 | 3i | 84 | |
10 | 3j | 82 | |
11 | 3k | 84 | |
12 | 3l | 78 | |
13 | 3m | 95 | |
14 | 3n | 84 | |
15 | 3o | 82 | |
16 | 3p | 82 | |
17 | 3q | 90 | |
18 | 3r | 82 | |
19 | 3s | 92 | |
20 | 3t | 90 | |
21 | 3u | 80 | |
22 | 3v | 70 | |
23 | 3w | 92 | |
24 | 3x | 88 | |
25 | 3y | 90 | |
26 | 3z | 80 |
a NMR yields.
Furthermore, in the case of cyclic epoxides such as cyclohexene oxide, trans products were obtained (Table 2). The trans stereochemistry of the corresponding products was determined from the coupling constants of the CH protons α to the heteroatoms in the 1H NMR spectra.
Reaction of cyclohexane oxide with in situ generated dithiocarbamate.
Entry | Amine | Product | Yield (%)a |
1 | 90 | ||
2 | 92 | ||
3 | 88 | ||
4 | 80 | ||
5 | 78 | ||
6 | 84 |
a NMR yields.
Aryl epoxides such as styrene oxide were reacted under these simple and green reaction conditions with short reaction times in a highly regioselective way, affording the corresponding product 7a as the major isomer along with a minor amount of 7b by cleavage at the benzylic position with the anti-Markovnikov addition (Scheme 2).
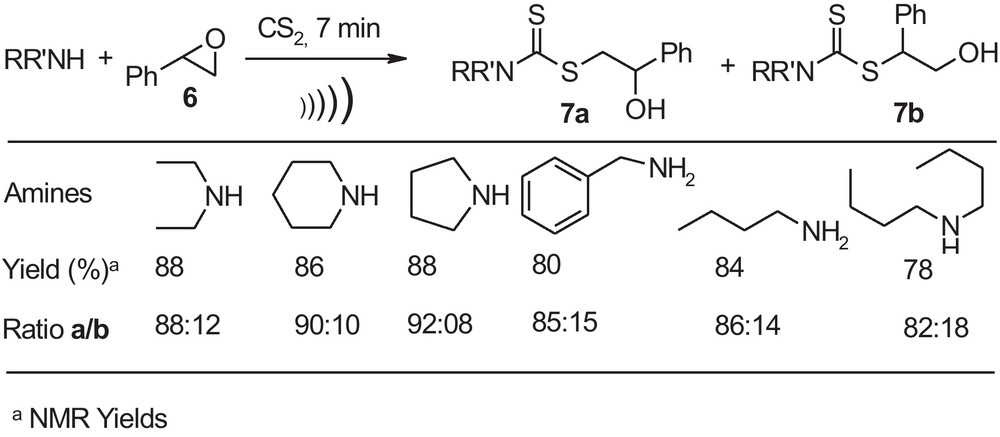
Dithiocarbamates derived from styrene oxide.
3 Conclusion
In summary, we have developed a mild and fast procedure for the clean synthesis of various 2-hydroxy dithiocarbamates with excellent yields in water under ultrasonic irradiation. This simple procedure is faster with high yields, and less laborious in isolation and purification procedures than those with iso-thiocyanates. Furthermore, the reaction was carried out in very mild conditions by just mixing the reactant with water, using a simple purification method, as it involves filtration, washing with water and recrystallization from ethanol or diethyl ether in some cases.
4 Experimental
4.1 General
Reactions were monitored by TLC and GC. FT-IR spectra were recorded using KBr disks on a Bruker Vector 22 FT-IR spectrometer and 1H NMR spectra were recorded on 500 MHz NMR spectrometer and 13C NMR spectra were recorded on 125 MHz NMR spectrometer respectively using CDCl3 or DMSO as a solvent; chemical shifts have been expressed in ppm downfield from TMS. Melting points were recorded on a Buchi 535 melting point apparatus and are uncorrected. All starting materials are commercially available and were purchased and used without further purification. Water and other solvents were distilled before use. Sonication was performed using a Hielscher ultrasonic device (Vial Tweeter at UIS250 v) with Sonotrode LS24d5 and with a frequency of 24 KHz and a nominal power of 200 W.
4.2 General procedure
A mixture of amines (2 mmol), carbon disulfide (3 mmol) and water (3 mL), epoxides (2 mmol) were added in test tube surrounded by water bath for cooling. The mixture was subjected to ultrasound irradiation for 7–10 min at 30–35 °C. The precipitate was filtered off, washed with water, ethanol or diethyl ether and dried in air to afford the corresponding dithiocarbamate derivatives. The reaction products were obtained in high purity (1H NMR and melting point instruments) in most cases, and did not require further purification by recrystallization or column chromatography. In the cases of liquid or viscose products, the aqueous mixture was extracted with 10 mL of diethyl ether or ethyl acetate and dried over anhydrous Na2SO4, and the solvent was removed under reduced pressure to give the desired products. All compounds were known and IR and 1H NMR spectra were found to be identical with the ones described in the literature.
4.3 Selected data
4.3.1 2-hydroxy-3-phenoxypropyl diethylcarbamodithioate (3a)
1H NMR (500 MHz, CDCl3): δ = 1.27 (t, J = 7.2 Hz, 6 H), 3.62–3.81 (m, 4 H), 3.95–4.18 (m, 4 H), 4.32 (m, 1 H), 6.89–7.00 (m, 3 H), 7.28 (t, = 7.5 Hz, 2 H). 13C NMR (125 MHz, CDCl3): δ = 12.08, 12.9, 40.2, 47.5, 50.5, 69.9, 70.9, 115.5, 121.5, 129.9, 158.9, 195.9.
4.3.2 2-hydroxy-3-phenoxypropyl piperidine-1-carbodithioate (3b)
1H NMR (500 MHz, CDCl3): δ = 1.53–1.64 (m, 6 H), 3.54–4.25 (m, 10 H), 6.87–6.88 (m, 3 H), 7.21 (t, J = 6.8 Hz, 2 H). 13C NMR (125 MHz, CDCl3): δ = 14.7, 24.7, 25.9, 40.1, 51.8, 53.7, 69.8, 70.9, 115.0, 121.2, 129.8, 158.9, 195.7.
4.3.3 2-hydroxy-3-phenoxypropyl pyrrolidine-1-carbodithioate (3c)
1H NMR (500 MHz, CDCl3): δ = 1.97–2.12 (m, 4 H), 3.59–3.84 (br, 5 H), 3.92 (t, J = 6.8 Hz, 2H), 4.09 (t, J = 6.8 Hz, 2H), 4.26 (m, 1H), 6.90–6.98 (m, 3H), 7.23–7.25 (m, 2H). 13C NMR (125 MHz, CDCl3): δ = 24.1, 27.2, 40.6, 52.4, 56.8, 71.0, 71.8, 115.8, 122.2, 130.2, 159.2, 194.2. Anal. calcd. for C14H19NO2S2: C 56.56, H 6.40, N 4.71; found: C 56.56, H 6.35, N 4.81.
4.3.4 2-hydroxy-3-phenoxypropyl sec-butylcarbamodithioate (3d)
1H NMR (500 MHz, CDCl3): δ = 0.94 (t, J = 6.4 Hz, 3H), 1.24 (t, J = ? Hz, 3H), 1.56–1.67 (m, 3H), 3.41–3.52 (m, 2H), 4.33–5.2a (m, 4H), 6.91–6.99 (m, 3H), 7.29 (t, J = 5.3 Hz, 2H), 8.20–8.42 (brs, 1H). 13C NMR (125 MHz, CDCl3): δ = 10.9, 19.4, 29.1, 38.8, 55.2, 70.0, 70.7, 115.0, 121.7, 130.0, 158.7, 196.3.
4.3.5 2-hydroxy-3-phenoxypropyl dibutylcarbamodithioate (3e)
1H NMR (500 MHz, CDCl3): δ = 0.96 (t, J = 7.29 Hz, 6 H), 1.36 (q, J = 7.2 Hz, 4H), 1.68–1.71 (m, 4H), 3.61–4.61 (m, 9H), 6.91–6.93 (m, 3H), 7.26 (t, J = 7.3 Hz, 2H).
4.3.6 2-hydroxy-3-phenoxypropyl tert butylcarbamodithioate (3f)
1H NMR (500 MHz, CDCl3): δ = 1.19–2.6 (m, 11H), 3.40–4.39 (m, 6H), 6.85–6.98 (m, 3H), 7.28 (t, J = 7.2 Hz, 2H), 8.11–8.50 (m, brs, NH). 13C NMR (125 MHz, CDCl3): δ = 25.2, 25.8, 3.0, 38.9, 56.6, 70.4, 70.7, 115.8, 121.7, 130.0, 158.7, 1985.8.
4.3.7 2-hydroxy-3-phenoxypropyl benzylcarbamodithioate (3g)
1H NMR (500 MHz, CDCl3): δ = 3.48 (dd, J = 7.01, 6.9 Hz, 1H), 3.59 (dd, J = 3.2, 3.4 Hz, 1H), 3.99–4.01 (m, 3H), 4.33–4.35 (m, 2H), 4.93 (d, J = 5.01, 2H), 6.95–7.07 (m, 3H), 7.35–7.07 (m, 3H), 7.35–7.39 (m, 7H), 8.42 (brs, NH). 13C NMR (125 MHz, CDCl3): δ = 39.1, 51.7, 69.4, 70.8, 115.2, 121.8, 127.8, 128.1, 129.0, 130.1, 135.7, 158.8, 198.1.
4.3.8 2-hydroxy-3-phenoxypropyl butylcarbamodithioate (3h)
1H NMR (500 MHz, CDCl3): δ = 0.95 (t, J = 7.2 Hz, 3 H), 1.36 (m, 2H), 1.60–1.66 (m, 2H), 3.46 (dd, J = 6.9, 7.01 Hz, 1H), 3.54 (dd, J = 3.1–3.8 Hz, 1H), 3.68–9.70 (m, 2H), 4.01–4.12 (m, 2H), 4.33–4.39 (m, 1H), 6.92–7.0 (m, 3H), 7.30 (t, J = 8.0 Hz, 2H), 8.5 (brs, NH, 1H). 13C NMR (125 MHz, CDCl3): δ = 4.1, 20.7, 31.0, 38.9, 47.9, 70.1, 71.2, 115.1, 129.3, 158.8, 197.3.
4.3.9 2-hydroxy-3-isopropoxypropyl diethylcarbamodithioate (3i)
1H NMR (500 MHz, CDCl3); δ = 1.01–1.16 (br, 12H), 3.40–3.45 (br, 6H), 3.66 (br, 2H), 3.88 (br, 3H). 13C NMR (125 MHz, CDCl3): δ = 12.1, 12.9, 23.2, 41.8, 48.01, 50.1, 71.3, 72.1, 73.2, 194.9.
4.3.10 2-hydroxy-3-isopropoxypropyl piperidine-1-carbodithioate (3j)
1H NMR (500 MHz, CDCl3) δ = 1.08 (d, J = 5.9 Hz, 6H), 1.64–168 (br, 6H), 3.12–315 (br, 1H), 3.30–3.32 (m, 2H), 3.44 (dd, J = 7.3, 4.1 Hz, 1H), 3.53–3.56 (m, 2H), 3.90–3.91 (br, 3H), 4.21–4.23 (br, 2H). 13C NMR (125 MHz, CDCl3): δ 23.1, 24.9, 26.2, 26.9, 41.1, 52.4, 54.2, 71.1, 71.9, 73.1, 195.9.
4.3.11 2-hydroxy-3-isopropoxypropyl benzylcarbamodithioate (3k)
1H NMR (500 MHz, CDCl3): δ = 1.07 (d, J = 6.1 Hz, 6H), 3.14 (dd, J = 6.8–7.1 Hz, 1H), 3.29–3.95 (m, 6H), 4.78 (brs, 0.9 H), 5.17 (brs, 0.1 H), 7.19–7.23 (m, 5H), 8.86 (brs, 0.1 H), 8.99 (brs, 0.9 H); 13C NMR (125 MHz, CDCl3): δ = 22.5, 39.0, 51.1, 70.6, 70.9, 72.6, 128.0, 128.5, 129.2, 136.9, 197.8.
4.3.12 2-hydroxy-3-isopropoxypropyl butylcarbamodithioate (3l)
1H NMR (500 MHz, CDCl3): δ = 0.69 (q, J = 6.9 Hz, 3H), 0.91 (d, J = 5.8 Hz, 6H), 1.12–1.39 (m, 4H), 3.01–3.76 (m, 9H), 8.74 (brs, 1H). 13C NMR (125 MHz, CDCl3): δ = 13.9, 20.3, 22.3, 30.4, 38.9, 47.2, 54.1, 67.3, 69.9, 70.2, 196.5.
4.3.13 3-(allyloxy)-2-hydroxypropyl pyrrolidine-1-carbodithioate (3p)
1H NMR (500 MHz, CDCl3): δ = 1.97–2.10 (m, 4H), 3.48–4.04 (m, 12H), 5.19 (d, J = 10.3 Hz), 5.26 (d, J = 10.3 Hz, 2H).
4.3.14 2-hydroxypropyl piperidine-1-carbodithioate (3t)
1H NMR (500 MHz, CDCl3): δ = 1.15 (d, J = 6.2 Hz, 3H), 1.54–1.59 (br, 6H), 3.18–4.19 (m, 8H). 13C NMR (125 MHz, CDCl3): δ = 22.9, 24.5, 25.8, 26.4, 45.3, 51.9, 53.7, 67.2, 196.0.
4.3.15 2-hydroxypropyl pyrrolidine-1-carbodithioate (3u)
1H NMR (500 MHz, CDCl3): δ = 1.10 (d, J = 6.8 Hz, 3H), 1.83–1.97 (m, 4H), 3.13–3.89 (m, 6H). 13C NMR (125 MHz, CDCl3): δ = 22.8, 24.6, 26.4, 44.6, 51.0, 55.4, 67.0, 192.9.
4.3.16 2-hydroxypropyl sec-butylcarbamodithioate (3v)
1H NMR (500 MHz, CDCl3): δ = 0.86–0.90 (m, 6H), 1.21–1.58 (m, 4H), 3.31–4.58 (m, 6H), 9.05 (brs, NH). 13C NMR (125 MHz, CDCl3): δ = 14.2, 19.5, 22.8, 33.0, 43.9, 53.7, 67.9, 197.4.
4.3.17 2-hydroxybutyl diethylcarbamodithioate (3w)
1H NMR (500 MHz, CDCl3): δ = 0.86 (t, J = 7.4 Hz, 3H), 1.21 (s, 6H), 1.50 (m, 2H), 3.05 (br, 1H), 3.20 (dd, J = 7.4 and 6.5 Hz, 1H), 3.47 (dd, J = 10.5 and 3.6 Hz, 1H), 3.66 (m, 3H), 3.90 (br, 2H). 13C NMR (125 MHz, CDCl3 + CCl4): δ = 10.3, 11.9, 12.9, 29.8, 43.7, 47.2, 50.1, 72.5, 196.2.
4.3.18 2-hydroxybutyl piperidine-1-carbodithioate (3x)
1H NMR (500 MHz, CDCl3 + CCl4): δ = 0.86 (t, J = 7.3 Hz, 3H), 1.44–1.56 (br, 6H), 3.20 (dd, J = 7.5 and 6.3 Hz, 1H), 3.41 (m, 2H), 3.61 (m, 1H), 3.82 (br, 2H), 4.12 (br, 2H). 13C NMR (125 MHz, CDCl3 + CCl4): δ = 10.3, 20.9, 26.13, 29.4, 45.0, 51.5, 55.4, 72.2, 196.1.
4.3.19 2-hydroxybutyl pyrrolidine-1-carbodithioate (3y)
1H NMR (500 MHz, CDCl3): δ = 0.82 (t, J = 7.4 Hz, 3H), 1.42 (m, 2H), 1.84 (t, J = 6.8 Hz, 2H), 1.94 (t, J = 6.8 Hz, 2H), 3.15 (br, 1H), 3.27 (dd, J = 7.4 and 6.7 Hz, 1H), 3.47–3.73 (m, 4H), 3.76 (br, 2H). 13C NMR (125 MHz, CDCl3): δ = 10.3, 24.7, 25.4, 29.7, 43.0, 51.2, 55.7, 72.4, 193.8.
Acknowledgements
The financial support of this work provided by the Iran National Science Foundation (INSF) is gratefully appreciated.