1 Introduction
Multicomponent reactions (MCRs), by virtue of their synthetic efficiency, high atom economy, procedural simplicity and an easy access in the creation of large libraries of structurally related compounds, have attracted much attention from synthetic organic chemists [1]. Especially the last decade has witnessed tremendous developments in multicomponent condensation reactions and one of the important developments amongst them involves the use of small organic molecules as catalysts, organocatalysts [2,3]. These catalysts are known to offer advantages such as lowering of activation energy, metal-free environment, reduced toxicity, possibility of reuse, etc. A range of Lewis/Brønsted acids, bases, ureas, amino acids, etc. are continuously being screened for their catalytic potential in various organic transformations [4]. At the same time, search for an efficient, easily accessible, inexpensive and environmentally friendly organocatalyst is a constant endeavor.
Chromene moiety often appears as an important structural motif in many naturally occurring compounds with important biological activities [5]. Amongst chromene family members, 2-amino-4H-chromenes (2-amino-4H-benzo[b] pyrans) constitute an important class of compounds customarily used in pigments, cosmetics as well as biodegradable agrochemicals [6]. Owing to their wide spectrum of biological activities such as antibacterial, mutageneticidal, antiviral, antihypertensive, antitumor activities, etc., these compounds have also attracted the attention of medicinal chemists [7]. They also find application as cognitive enhancers in the treatment of neodegradable diseases like Alzheimer's disease, insomnia, etc. [8] The recent SAR studies on 4H-chromene derivatives revealed that, in cancer therapy, 2-amino-4H-chromenes of type A (e.g. HA-14-1, Fig. 1) serve as tumor antagonists and that a family of related alkyl (4H-chromen-4-yl) cyano acetates exhibit binding activity for surface pocket of cancer-implicated Bcl-2 proteins and induce apoptosis in follicular lymphoma-B cells as well as leukemia HL-60 cells [9]. On the other hand, compound D (Fig. 1) has been reported to inhibit mitogen-activated protein kinase-activated protein kinase-2 (MK-2) and to suppress the expression of TNF-α in U 937 cells [10]. A few other compounds containing the 2-amino-4H-chromene moiety (B and C, Fig. 1) are also known to exhibit important biological activities [11].

Structures of few bio-active chromene derivatives.
As regards the synthesis of 2-amino-4H-chromene derivatives, a literature survey revealed that a useful number of protocols have been reported earlier for the synthesis of 4-aryl-substituted 2-amino-4H-chromene derivatives following multicomponent condensation between aromatic aldehydes, malononitrile/ethyl cyanoacetate with phenols, polyphenols, 1,3-diketones, coumarins, etc. [12] On the other hand, only a limited number of protocols have been reported for the synthesis of 4-(functionalized-alkyl)-substituted chromene derivatives of type A–F (Fig. 1). For instance, the synthesis of A as well as of F (Fig. 1) has been reported earlier by one-pot condensation between salicylaldehyde and alkyl cyanoacetate/malononitrile using Amberlyst-21, alumina, K-exchanged zirconium phosphonate, ammonium acetate, molecular sieves and KF-sodium acetate as catalysts [13a–f]. On the other hand, Elinson et al. have reported an electrochemical method for library synthesis of 2-amino-4H-chromene derivatives of type B, C and E (Fig. 1) [14]. Quite recently, Gao et al. have reported the asymmetric synthesis of 2-amino-4H-chromenes of type E (Fig. 1) [15]. Each of these methods has its own merits. However, a few of them are plagued with the use of large excess of catalyst, moderate diastereoselectivity (especially towards the synthesis of A, C and E), longer reaction times, essentiality of conventional work-up procedure, etc. In view of the increased biological importance of 2-amino-4H-chromene derivatives, development of a simple, eco-safe, high yielding and scalable protocol for their synthesis is desirable.
2 Results and discussion
Our interest in this class of compounds stems from our continued efforts towards the development of eco-safe and operationally simple synthetic methodologies [16]. In our recent communication, we have reported for the first time the use of a commercially available, inexpensive, non-toxic and easy to handle diethylamine as an efficient organocatalyst for one-pot synthesis of β-phosphonomalononitriles, 2, as well as diethyl (2-amino-4H-chromen-4-yl) phosphonates, 3, by three-component condensation between an aldehyde, malononitrile and dialkyl phosphite [17a] (Scheme 1a). In continuation of these studies herein, we describe efficacy of diethylamine in the synthesis of medicinally privileged 2-amino-4H-chromenes by pseudo three-component condensation between salicylaldehyde and three different C–H acids at ambient temperature (Scheme 1b).
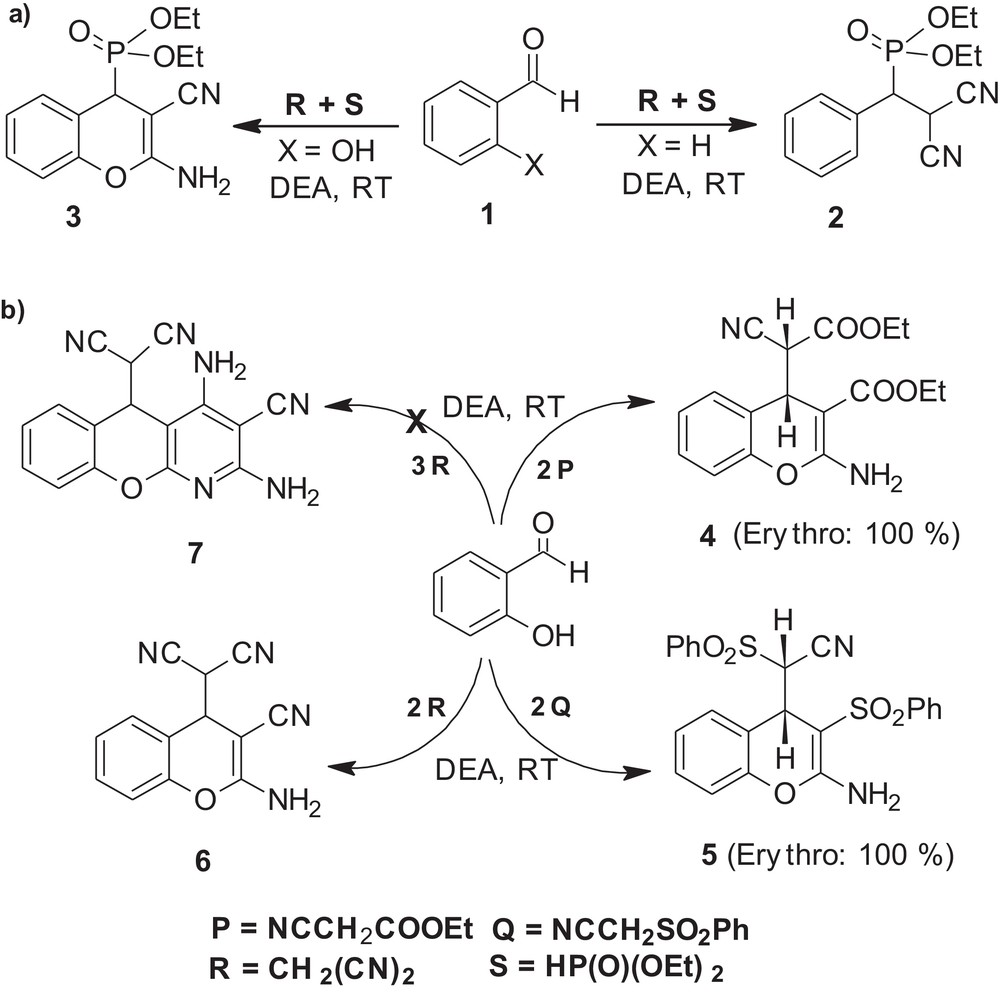
Diethylamine-catalyzed synthesis of Bcl-2 protein antagonists and its analogs.
As a model compound, we initially planned to undertake the synthesis of ethyl 2-amino-4-(1-cyano-2-ethoxy-2-oxoethyl)-4H-chromene-3-carboxylate, 4a. Accordingly, a template reaction was performed between salicylaldehyde and ethyl cyanoacetate (1:2 equiv) in the presence of diethylamine as a catalyst, at ambient temperature (Scheme 1b). Upon addition of the catalyst, an exothermic reaction took place and after completion of the reaction (TLC), the desired cyanoester, 4a, was obtained in almost quantitative yield simply by decomposition of the reaction mixture using water followed by filtration of the resultant solid. It was further gratifying to note that the resultant product, 4a, was pure and was obtained as a single diastereoisomer (1H-NMR). By comparison of the 1H-NMR data with that reported earlier, it was assigned an erythro configuration. As the reaction conditions employed for the synthesis of the model compound, 4a, were very close to those defined for an “ideal synthesis” by Wender [18], further optimization of the reaction conditions was surmised unnecessary. So as to demonstrate the scope of the developed protocol, under the same reaction conditions, salicylaldehydes bearing electron-withdrawing as well as electron-donating groups were then allowed to react with ethyl cyanoacetate (2 equiv). In all the cases studied, it was noticed that, under solvent-free condition, possibly due to solubility problems of starting aldehydes, reactions did not go to completion. However, with the use of a minimum amount of ethanol as an ecofriendly solvent, corresponding chromene derivatives, 4b–h, were obtained in excellent yields and purity (Table 1). In fact, none of them required purification using conventional techniques and diastereoselectivity was also found to be independent of the electronic properties of the substituents present on the salicylaldehyde.
Diethyl amine catalyzed synthesis of 2-amino-4H-chromenesa.
No. | R | R1 | dr | Time (h) | Yield (%) | ||
1 | 4a | H | H | 100 | 1.5 | 96 | |
2 | 4b | Cl | H | 100 | 2.0 | 95 | |
3 | 4c | Cl | Cl | 100 | 1.5 | 95 | |
4 | 4d | Br | H | 100 | 2.5 | 93 | |
5 | 4e | Br | Br | 100 | 2.0 | 96 | |
6 | 4f | OCH3 | H | 100 | 1.5 | 92 | |
7 | 4g | NO2 | H | 100 | 2.0 | 95 | |
8 | 4h | H | CH | 100 | 2.5 | 94 | |
9 | 5a | H | H | 100 | 3 | 93 | |
10 | 5b | Cl | H | 100 | 3.5 | 91 | |
11 | 5c | Cl | Cl | 100 | 8 | 93 | |
12 | 5d | Br | H | 100 | 4 | 92 | |
13 | 5e | Br | Br | 100 | 6 | 95 | |
14 | 5f | NO2 | H | 100 | 3 | 91 | |
15 | 5gb | H | H | 100 | 8 | 92 | |
17 | 6a | H | H | – | 3.0 | 98 | |
18 | 6b | Cl | H | – | 2.5 | 94 | |
19 | 6c | Br | H | – | 3.0 | 92 | |
20 | 6d | OCH3 | H | – | 2.5 | 94 | |
21 | 6e | NO2 | H | – | 3.0 | 90 | |
22 | 6f | H | CH3 | – | 2.5 | 92 |
a Reaction conditions: salicylaldehyde (2 mmol), ethyl cyanoacetate/phenylsulfonyl acetonitrile/malononitrile (4 mmol), ethanol (3 mL), diethylamine (10 mol %), RT.
b Starting from 2-hydroxy-4-methoxy benzaldehyde.
During the development of protocols, especially towards diastereoselective synthesis of bio-active molecules, scalability is one of the important aspects. Hence, under the established reaction conditions, the synthesis of the Bcl-2 protein antagonist, 4d, (A, HA-14-1, Fig. 1), was then carried out on 5 g scale using 5-bromo salicylaldehyde and ethyl cyanoacetate as substrates. To our delight, the desired product, 4d, was once again obtained as a single diastereoisomer, in excellent yield as well as purity.
Encouraged by this initial success in diastereoselective and scalable synthesis of Bcl-2 protein antagonist, 4d, we planned to extend these studies towards the synthesis of other chromene derivatives.
The compounds containing the sulfone moiety often serve as intermediates in the preparation of chemically significant and biologically active compounds and they have been a subject of an extensive investigation [19,20]. Taking into account the well-established biological activity of 4d (HA-14-1, Fig. 1) as a tumor antagonist, we surmised that replacement of carbethoxyl group in 4 with phenylsulfonyl group would lead to New Chemical Entities (G, Fig. 1) with possible biological activities. Accordingly, [2-amino-3-(phenylsulfonyl)-4H-chromen-4-yl] (phenylsulfonyl) acetonitrile, 5a, was chosen as a representative compound and under solvent-free conditions a model reaction was performed between salicylaldehyde and phenylsulfonyl acetonitrile (1:2 equiv) using diethylamine as the catalyst (Scheme 1b). The reaction did not go to completion, which indicated that the use of a solvent was essential.
Owing to our particular interest in performing the organic transformations using a minimum amount of solvent and due to the poor solubility of phenylsulphonyl acetonitrile in ethanol medium, after careful studies, dioxane-ethanol (1:10, v/v) was identified to be an appropriate reaction medium to drive the model reaction to completion at ambient temperature in acceptable time (3 h). Upon completion of the reaction (TLC), the desired 2-amino-4H-chromene, 5a, was obtained as a yellowish solid simply upon decomposition of the reaction mixture with water followed by filtration of the resultant solid. From spectral studies, it was identified to be a single diastereoisomer (erythro, 1H-NMR). Under the same reaction conditions, various substituted salicylaldehydes also furnished the desired disulfones, 5b–f, in almost quantitative yield and as a single diastereoisomer.
Taking clues from the results obtained in the synthesis of 4 as well as of 5, we then turned our attention towards the synthesis of trinitriles, 6 (Scheme 1b). As yet, although we have not come across any reports on the biological potential of trinitriles, 6, the silent objective behind their synthesis was the possible extension of the protocol towards the synthesis of pyrano-pyridine, D (Fig. 1) by pseudo four-component condensation between salicylaldehyde and malononitrile (3 equiv). Initially, a pseudo three-component reaction was performed between salicylaldehyde and malononitrile (2 equiv) under solvent-free conditions (Scheme 1b). Upon addition of diethyl amine, an appreciably exothermic reaction took place and the resultant product was noticed to be a mixture (TLC). During optimization of the reaction conditions, it was very interesting to note that, rather than lowering the reaction temperature, the use of ethanol as a solvent proved to be beneficial to the synthesis of the desired trinitrile, 6a, in excellent yield as well as purity. Without modification of the reaction conditions, various substituted salicylaldehydes also furnished the corresponding trinitriles, 6b–f in excellent yields (Table 1) and none of them required purification employing conventional purification techniques. Subsequently, a pseudo four-component reaction was also carried out between salicylaldehyde and malononitrile (1:3 equiv) (Scheme 1b). However, against the expected pyrano-pyridine, D, the resultant product was identified to be 6a (NMR and mp) and modifications of the reaction conditions were not found to be useful in synthesis of D, exclusively.
Lately, it was thought worthwhile to explore the potential of the developed protocol towards the synthesis of B as well as of C (Fig. 1). Accordingly, two model reactions were performed between salicylaldehyde, malononitrile and diethyl malonate/ethyl cyanoacetate in the presence of diethylamine as a catalyst. Upon completion of the reactions, possibly due to competitive rates of the Knoevenagel condensation reaction between salicylaldehyde and malononitrile, diethyl malonate as well as ethyl cyanoacetate, resultant products were identified to be a difficult-to-separate mixture and we did not pursuit for their synthesis.
Finally, a comparison was made between the present protocol and others reported earlier for the synthesis of 4d, as a model compound. The results summarized in Table 2 clearly demonstrate the superiority of the present protocol over all the earlier reported protocols in terms of cost, diastereoselectivity, operational simplicity as well as ease of isolation of the product, avoiding conventional purification techniques.
Comparison between various reported methods and the present method for the synthesis of 4da.
Sr. No. | Catalyst | mol %/[gm] | Time (h) | Yield (%) | dr | Reference |
1. | Amberlyst-21 | [2] | 2 | 88 | 4:1 | [13a] |
2. | Ammonium acetate | 100 | 2 | 77 | NMb | [13b] |
3. | Zr(KPO4)2 | 0.050 | 3 | 97 | 100c | [13c] |
4. | Aluminad | [0.4] | 1 | 75 | 4:1 | [13d] |
5. | Molecular sieves | [3] | 14 | 86 | 100 | [13e] |
6. | Electricity | – | 3 | 69 | 2:1 | [14] |
7. | Diethylamine | 10 | 2.5 | 93 | 100 | This work |
a Reaction conditions: 5-bromosalicylaldehyde (2 mmol), ethyl cyanoacetate (4 mmol), catalyst.
b Per mmol of aldehyde.
c After crystallization.
d Using salicylaldehyde and methyl cyanoacetate.
3 Conclusion
In conclusion, we have developed an extremely simple, environmentally benign, and diversity-oriented protocol for the diastereoselective synthesis of medicinally privileged 2-amino-4H-chromene scaffolds by condensation between salicylaldehyde and three different active C–H acids, using diethylamine as a novel organocatalyst. Easy commercial availability of the catalyst at extremely low cost, ambient temperature and avoidance of conventional work-up as well as purification makes this energy-efficient protocol a “near-ideal synthesis”. To the best of our knowledge, the literature to date contains no precedent for such a simple, economical and environmentally conscious protocol for the synthesis of wide range of 2-amino-4H-chromene derivatives.
As regards the synthesis of various 2-amino-4H-chromenes, three observations are noteworthy:
- • the reactions involving the synthesis of 4, 5 as well as 6 are exothermic and, based upon our earlier experiences in phospha-Michael [17a] as well as in aza-Michael addition reactions [17b], we believe that the initial exothermy produced upon addition of the catalyst is one of the driving forces of the reaction;
- • rather than the sequential path, the formation of 2-amino-4H-chromene derivatives follows a cascade Knoevenagel-carba-Michael path;
- • in a base-catalysed synthesis of [4-(functionalized-alkyl)]-2amino-4H-chromene derivatives involving a three-component reaction between salicylaldehyde and two different C–H acids, it is essential that the rates of the Knoevenagel condensation reaction between salicylaldehyde and selected C–H acids should be appreciably different.
In support of all these observations, studies are currently directed towards the synthesis of few more 2-amino-4H-chromene derivatives; these results will be reported in our next communication.
4 Experimental
4.1 Typical experimental procedure for the synthesis of 4/5/6
To a well-stirred solution of salicylaldehyde (2 mmol) and ethyl cyanoacetate, malononitrile or phenylsulphonyl acetonitrile (4 mmol) in ethanol (3 mL)/dioxane-ethanol (1:10, v/v, 3 mL) was added diethylamine (10 mol %) and stirring continued. Upon completion of the reaction, water (10 mL) was added and stirring was continued till a free flowing solid was obtained. It was filtered and washed successively with water and dried. It was then washed with n-hexane and dried. The resulting products were found to be pure (TLC) and did not require any further purification.
Spectral data of all the known compounds is summarized in the supporting information, File 1. Spectral data of the new compounds are summarized below.
4.2 2-Amino-3-benzenesulphonyl-4H-chromen-4-yl–benzenesulphonyl acetonitrile, 5a
White solid; IR (KBr) = 3485, 3266, 2242, 1639, 1402, 1208, 1148, 1085, 852, 762, 629 cm−1; 1H NMR (300 MHz, CDCl3): δ = 4.77 (1H, d, J = 1.8 Hz, CH), 5.02 (1H, d, J = 1.8 Hz, CH), 6.12 (2H, br s, NH2), 7.06–8.06 (14H, m, ArHs); 13C NMR (75.4 MHz, d6-DMSO): δ = 35.04 (CH), 64.10 (CH), 76.19 (=CH), 112.62, 116.37, 118.28, 124.78, 126.14, 128.62, 129.30, 129.88, 130.26, 132.99, 135.13, 138.04, 142.27, 150.49, 160.56 (ArCs) ppm.
4.3 2-Amino-3-benzenesulphonyl-6-chloro-4H-chromen-4-yl–benzenesulphonyl acetonitrile, 5b
Colorless solid; IR (KBr) = 3443, 3332, 2248, 1642, 1396, 1199, 1137, 1085 cm−1; 1H NMR (300 MHz, CDCl3): δ = 4.70 (1H, s, CH), 5.00 (1H, d, J = 1.2 Hz, CH), 6.14 (2H, br s, NH2), 7.01 (1H, d, J = 9.1 Hz, ArH), 7.29–7.79 (10H, m, ArHs), 7.95 (1H, d, J = 7.5 Hz, ArH), 8.04 (1H, d, J = 8.1 Hz, ArH); 13C NMR (75.4 MHz, d6-DMSO): δ = 34.56 (CH), 63.61 (CH), 78.04 (=CH), 112.34, 117.66, 119.64, 126.54, 128.88, 129.43, 129.82, 130.15, 130.22, 130.31, 133.33, 135.06, 137.79, 141.52, 148.84, 159.35 (ArCs) ppm.
4.4 2-Amino-3-benzenesulphonyl-6,8-dichloro-4H-chromen-4-yl–benzenesulphonyl acetonitrile, 5c
Colorless solid; IR (KBr) = 3459, 3329, 2247, 1638, 1396, 1334, 1204, 1085, 859 cm−1; 1H NMR (300 MHz, CDCl3): δ = 4.71 (1H, d, J = 2.1 Hz, CH), 5.00 (1H, d, J = 2.1 Hz, CH), 6.22 (2H, br s, NH2), 7.41-7.81 (8H, m, ArHs), 7.94 (2H, dd, J = 8.0 and 1.5 Hz, ArHs), 8.03 (2H, dd, J = 8.1 and 1.5 Hz, ArHs); 13C NMR (75.4 MHz, DMSO): δ = 35.17 (CH), 63.68 (CH), 75.78 (=CH), 112.32, 121.34, 122.43, 126.22, 128.58, 128.66, 129.44, 129.97, 130.31, 133.30, 135.39, 137.53, 141.81, 145.36, 159.93 (ArCs) ppm.
4.5 2-Amino-3-benzenesulphonyl-6-bromo-4H-chromen-4-yl–benzenesulphonyl acetonitrile, 5d
Colorless solid; IR (KBr) = 3443, 3356, 2247, 1636, 1400, 1335, 1232, 1136, 1086, 923 cm−1; 1H NMR (300 MHz, CDCl3): δ = 4.69 (1H, d, J = 1.8 Hz, CH), 5.01 (1H, d, J = 1.8 Hz, CH), 6.95 (2H, br s, NH2), 7.42 (1H, d, J = 2.1 Hz, ArH), 7.53 (1H, dd, J = 9.1 and 2.1 Hz), 7.56–7.80 (7H, m, ArHs), 7.95 (2H,d J = 7.8 Hz), 8.04 (2H, d, J = 7.8 Hz); 13C NMR (75.4 MHz, d6-DMSO): δ = 35.06 (CH), 63.95 (CH), 75.48 (=CH), 112.66, 116.73, 118.59, 120.69, 126.12, 128.75, 129.60, 130.24, 132.77, 133.02, 133.28, 135.63, 137.61, 142.52, 149.86, 160.44 (ArCs) ppm.
4.6 2-Amino-3-benzenesulphonyl-6,8-dibromo-4H-chromen-4-yl–benzenesulphonyl acetonitrile, 5e
Colorless solid; IR (KBr) = 3457, 3324, 2248, 1639, 1204, 1184 cm−1; 1H NMR (300 MHz, CDCl3): δ = 4.70 (1H, d, J = 2.1 Hz), 4.99 (1H, d, J = 2.1 Hz), 6.25 (2H, br s, NH2), 7.56–7.95 (8H, m, ArCH), 8.01 (2H, d, J = 7.8 Hz), 8.05 (2H, d, J = 7.5 Hz); 13C NMR (75.4 MHz, d6-DMSO): δ = 35.42 (CH), 63.79 (CH), 75.88 (=CH), 111.21, 112.51, 116.77, 122.14, 126.28, 128.83, 129.63, 130.25, 132.24, 133.34, 135.64, 135.77, 137.57, 142.42, 147.11, 160.07 (ArCs) ppm.
4.7 2-Amino-3-benzenesulphonyl-6,8-dibromo-4H-chromen-4-yl–benzenesulphonyl acetonitrile, 5f
Colorless solid; IR (KBr) = 3445, 3329, 2242, 1641, 1529, 1402, 1349, 1218, 1154, 1087, 746, 591 cm–1; 1H NMR (300 MHz, CDCl3): δ = 4.69 (1H, s, CH), 4.90 (1H, s, CH), 7.23 (1H, d, J = 9.0 Hz, ArH), 7.43–7.93 (12H, m, ArH and NH2), 8.17 (1H, d, J = 9.0 Hz, ArH), 8.38 (1H, d, J = 1.8 Hz, ArH); 13C NMR (75.4 MHz, d6-DMSO): δ = 34.98 (CH), 63.61 (CH), 75.56 (=CH), 112.34, 117.75, 119.53, 125.62, 126.23, 126.50, 128.74, 129.49, 130.06, 133.32, 135.50, 137.44, 142.00, 143.90, 154.77, 159.75 (ArCs) ppm.
4.8 2-Amino-3-benzenesulphonyl-5-methoxy-4H-chromen-4-yl–benzenesulphonyl acetonitrile, 5g
Colorless solid; IR (KBr) = 3439, 3350, 2324, 1638, 1394, 1279, 1135, 1080 cm–1; 1H NMR (300 MHz, CDCl3): δ = 3.80 (3H, s, OCH3), 4.69 (1H, d, J = 1.8 Hz), 4.99 (1H, d, J = 1.8 Hz), 6.15 (2H, br s, NH2), 6.58 (1H,d, J = 2.1 Hz, ArH), 6.75 (1H, dd, J = 8.1 and 2.4 Hz, ArH), 7.49–8.03 (12H, m, ArHs); 13C NMR (75.4 MHz, DMSO): δ = 34.33 (CH), 55.40 (OCH3), 64.00 (CH), 78.43 (=CH), 101.76, 109.95, 111.34, 112.57, 126.48, 128. 82, 129.29, 129.74, 131.05, 133.05, 134.83, 138.20, 141.86, 151.09, 159.61, 160.76 (ArCs) ppm.
Acknowledgements
M.A. Kulkarni and U.V. Desai are thankful to UGC, New Delhi for Junior Research Fellowship and financial assistance [F. 34-362-2008], respectively.