1 Introduction
Catalysis has proved to be very effective in making processes faster, with a reduced number of synthetic steps, more selective, and characterized by simpler waste disposal and improved safety. Apart from increasing selectivity, the use of catalysts results in easier isolation and purification of final products. Catalysis is an important tool in both the development of sustainable process for chemical transformations and waste minimization during these transformations [1–3].
Among various types of catalysts, polymer-supported ones play an important role in synthetic chemistry. They are associated with a number of advantages over conventional homogenous catalysts. The ease of separation of the supported species from a reaction mixture by filtration and washing, the reuse of a catalyst after regeneration, their adaptability to continuous flow processes and hence, the use in automated synthesis, the reduced toxicity and odor of supported species compared with low molecular weight species, chemical differences, such as prolonged activity or altered selectivity of a catalyst in supported form compared with its soluble analogue are some of them [4–9]. The polymeric catalysts also represent one of the most powerful tools for “green” sustainable chemistry, in the sense that they can be easily recovered and reused several times [10]. But the disadvantages include high cost and low loading of commercially available polymer supports and polymer-supported catalysts. These two drawbacks prevent their widespread applications in industrial processes. In this communication, we describe the development of a high-loading polymer-supported catalyst derived from one of the cheapest polymers available, i.e. poly(vinyl chloride) (PVC).
PVC is the most important of the vinyl thermoplastics, considering the volume of production and field of applications [11]. PVC is widely used for many purposes, ranging from building materials to healthcare products [12,13]. But, the applications of PVC in organic synthesis and as a support for the catalysts are rarely reported in the literature, but are gaining interest nowadays [14–19]. In this article, the preparation of a copper complex using PVC as a support is reported and this polymer-supported metal complex was found to be catalytically active in one of the most important reactions in organic chemistry, i.e. the Mannich reaction. As a classical reaction in organic chemistry, the Mannich reaction found widespread applications in synthetic and medicinal chemistry [20–29]. Even though a number of catalysts were reported for Mannich reactions, only few of them are polymer-supported. One important example of polymer-supported catalysts for the Mannich reaction was the polystyrene-supported BINAP-Pd complex reported by Fujii and Sodeoka [30]. Another example of polymer-supported catalysts for Mannich reactions was reported by Lee and Lee and includes ytterbium triflate supported on a poly(4-vinylpyridine/styrene) copolymer [31]. They used the catalysts in the synthesis of a small library of β-aminoketones from aldehydes, amines and preformed enols and the yield of the products vary from 84 to 97%. Other examples of polymer-supported catalysts used for Mannich reactions include dicyanoketene acetals supported on an ethyleneglycol dimethacrylate polymer, polystyrene-supported sulphonic acids, polystyrene-supported hydroxyprolines and polystyrene-supported scandium triflate [32–35]. But most of these methods included preformed enols or Schiff-bases as the reactants. Additionally, the supports used were either commercial polystyrene or polymers prepared from costly monomers. The present work attempts to eliminate these challenges and uses a combination of a reusable catalyst made from a cheap polymer and ecofriendly solvents for three-component Mannich reactions. The catalyst is a PVC-supported Schiff-base copper complex. The Schiff-base metal complexes are a class of compounds that have been studied extensively because of their attractive chemical and physical properties and their wide-ranging applications, especially in catalysis [36–38].
2 Experimental
2.1 Materials
Benzaldehyde, salicylaldehyde and cyclohexanone were purchased from S. D. Fine Chemicals (India) and were purified according to the standard procedures. 3-Nitro benzaldehyde and 4-nitro aniline were purchased from Lancaster Synthesis (UK) and were used as received. PVC (high molecular weight, K value 69–71) was purchased from Fluka and used as received. All the other chemicals and solvents were obtained from commercial sources and used as received.
2.2 Characterization
FTIR spectra were recorded with a JASCO model 4100 FTIR spectrometer on KBr pellets. 1H NMR spectra were recorded on a Bruker 300 MHz or 400 MHz instrument with TMS as the internal standard in CDCl3 (from NMR Research Centre, IISC, Bangalore). TG–DTA analysis was done using a PerkinElmer Diamond model TG/DTA system using platinum as the standard. ICP-AES spectra were recorded with a Thermo Electron IRIS Intrepid II XSP DUO model spectrometer. SEM images were recorded on a Jeol Model JSM-6390LV microscope. The sample was mounted on a carbon tape and coated with a thin film of gold before analysis.
2.3 Preparation of the polymer with amino groups (3)
PVC (1) (2 g, 31 mmol Cl) was suspended in ethylene diamine (2) (10.7 mL, 159.8 mmol) taken in a 50-mL round-bottom flask. The reaction mixture was stirred at room temperature for 2 h (Scheme 1). The light yellow colored polymer (3) was collected by filtration and washed with water (20 mL × 5 times) and a water–methanol mixture (2:1 v/v, 20 mL × 3 times). It was dried under vacuum at room temperature overnight. Yield = 1.93 g.
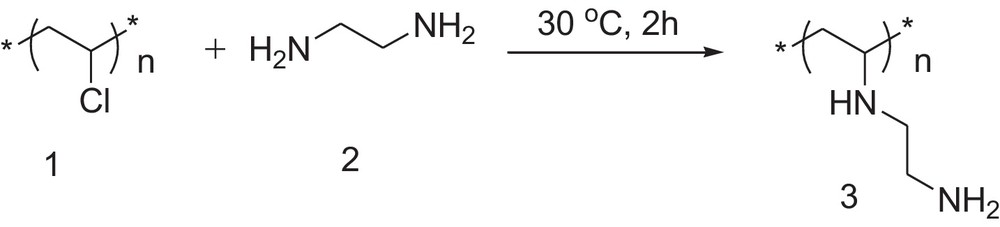
Synthesis of poly(vinyl chloride) with pendant amino groups.
FTIR (KBr) νmax cm−1: 3350, 3310 (NH2), 2900 (CH2), 1590 (NH) and 1465 (CH2).
2.4 Preparation of the polymer-supported Schiff-base (5)
The polymer (3) (2 g, 22 mmol NH2 groups) was suspended in absolute ethanol (20 mL) taken in a 100-mL round-bottom flask. Salicylaldehyde (4) (2.6 mL, 24 mmol) was added to it, followed by acetic acid (0.2 mL). The reaction mixture was refluxed under stirring for 24 h (Scheme 2). The bright yellow polymer (5) was collected by filtration and washed with hexane (5 mL × 5 times), water–methanol (2:1 v/v, 20 mL × 3 times) and water (20 mL × 5 times). It was dried under vacuum at room temperature overnight. Yield = 1.90 g.
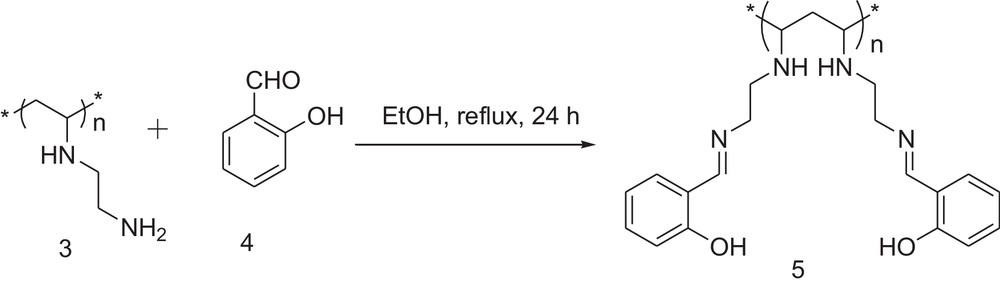
General method for the synthesis of the polymer-supported Schiff-base.
FTIR (KBr) νmax cm−1: 3600 (OH), 3064 (aromatic), 2900 (CH2), 1680 (CN) and 1463 (CH2); 1H NMR (300 MHz, CDCl3): δ 1.27–1.76 (CH2, polymer chain), 2.02 (NH, br), 2.56–2.86 (CH, polymer), 3.00 (CH2, CH2NH), 3.59 (CH2, CH2NCH), 5.5 (OH), 6.73–7.42 (aromatic), 8.0 (CHN),
2.5 Preparation of the copper (II) complex of the polymer-supported Schiff-base (6)
The polymer-supported Schiff-base (5) (2 g, 10 mmol), Cu(CH3COO)2 (0.90 g, 5 mmol) and 20 mL of methanol were added into a 50-mL round-bottom flask. The reaction mixture was refluxed for 12 h (Scheme 3). The yellowish polymer with a blue tinge (6) was collected by filtration, washed with water (20 mL × 5 times), a water–methanol mixture (2:1 v/v, 20 mL × 3 times) and dried under vacuum at room temperature overnight. Yield = 1.75 g.
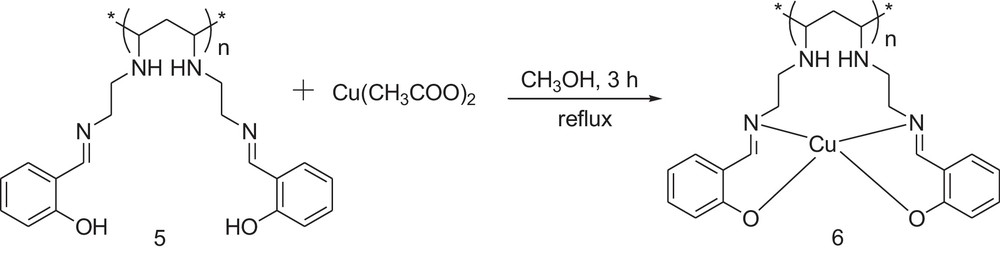
General method for the synthesis of the polymer-supported copper complex.
FTIR (KBr) νmax cm−1: 3064 (aromatic), 2900 (CH2), 1642 (CN), 1462 (CH2) and 635 (Cu-N); 1H NMR (300 MHz, CDCl3): δ 1.27–1.76 (CH2, polymer chain), 2.02 (NH, br), 2.56–2.86 (CH, polymer), 3.00 (CH2, CH2NH), 3.59 (CH2, CH2NCH), 6.73–7.42 (aromatic), 8.0 (CHN).
ICP-AES analysis of the complex showed that the loading of the metal ion was 4.26 mmol/g of the polymer.
2.6 General procedure for three-component Mannich reaction
A 50-mL round-bottom flask was charged with aldehyde (7) (5 mmol), aniline (8) (5.1 mmol), ketone (9) (5 mmol) and ethanol (5 mL). To it, the polymer-supported catalyst (6) (58.67 mg, 5 mol% of Cu) was added. The reaction mixture was stirred at room temperature (Scheme 4). The progress of the reaction was monitored by thin layer chromatography on a silica gel plate using a hexane–ethyl acetate mixture (25:1 v/v) as the mobile phase. After the completion of the reaction, the catalyst was filtered and washed with ethanol. The filtrate and washings were combined. The solvent was removed under vacuum and the pure product was isolated by column chromatography on silica gel using the hexane–ethyl acetate mixture (20:1 v/v) as the eluent. The β-amino ketones (10) were previously reported compounds and were characterized by FTIR and 1H NMR spectroscopy. The characterization data of some of the representative compounds are given below. Characterization data of other compounds are given as supplementary material.

Three-component Mannich reaction catalyzed by the polymer-supported copper complex.
2.7 2-[1′-(N-phenylamino)-1′-(phenyl)]methylcyclohexanone (Table 3, Entry 1)
FTIR (KBr) νmax cm−1: 3328, 3021, 2932, 2858, 1702, 1590, 1520, 1495, 1300; 1H NMR (400 MHz, CDCl3): δ 1.73–1.81 (m, 2H), 1.91–1.98 (m, 4H), 2.36–2.38 (m, 1H), 2.45–2.49 (m, 1H), 2.73–2.77 (m, 1H), 4.63 (d, J = 6.81 Hz, 1H), 4.74 (s, 1H), 6.52 (d, J = 7.73 Hz, 2H), 6.63 (t, J = 7.26 Hz, 1H), 7.06 (t, J = 7.37 Hz, 2H), 7.24 (t, J = 6.30 Hz, 1H), 7.33 (t, J = 6.76 Hz, 2H), 7.40 (d, J = 7.09 Hz, 2H).
Three-component Mannich reactions catalyzed by the polymer-supported copper complex.
Entry | R1 | R2 | Ketone | Time (h) | %Yielda,b |
1 | C6H5 | H | Cyclohexanone | 10 | 75 |
2 | 4-OCH3C6H4 | H | Cyclohexanone | 10 | 85 |
3 | 4-ClC6H4 | H | Cyclohexanone | 10 | 81 |
4 | 3-NO2C6H4 | H | Cyclohexanone | 8 | 77 |
5 | 4-OCH3C6H4 | 4-OCH3 | Cyclohexanone | 6 | 86 |
6 | 4-OHC6H4 | 4-Cl | Cyclohexanone | 10 | 76 |
7 | C6H5 | 4-OCH3 | Cyclohexanone | 10 | 72 |
8 | C6H5 | 4-NO2 | Cyclohexanone | 10 | 55 |
9 | C6H5CHCH2 | 4-OCH3 | Cyclohexanone | 6 | 82 |
10 | C4H3S | H | Cyclohexanone | 8 | 78 |
a Reaction conditions: 5 mmol of aldehyde, 5 mmol of cyclohexanone, 5.2 mmol of aniline, 5 mL of ethanol, catalyst (58.67 mg, 5 mol% of Cu).
b Isolated yield of the product.
2.8 2-[1′-(N-phenylamino)-1′-(4-nitrophenyl)]methylcyclohexanone (Table 3, Entry 4)
FTIR (KBr) νmax cm−1: 3386, 3021, 1673, 1527, 1348; 1H NMR (400 MHz, CDCl3): δ 1.58–1.66 (m, 2H), 1.75–1.79 (m, 1H), 1.95–2.08 (m, 3H), 2.34–2.42 (m, 2H), 2.86–2.89 (m, 1H), 4.59 (s, br, 1H), 4.84 (d, J = 5.2 Hz, 1H), 6.52–6.54 (d, J = 8.0 Hz, 2H), 6.66–6.70 (m, 1H), 7.08–7.12 (t, J = 8.0 Hz, 2H), 7.45–7.49 (t, J = 8.0 Hz, 1H), 7.77–7.80(m, 1H), 8.07–8.09 (d, J = 8.0 Hz, 1H), 8.24–8.26 (d, J = 7.6 Hz, 1H).
2.9 General procedure for recycling the catalyst
The catalyst (6) used for first cycle of reaction was washed carefully with ethanol (5 mL × 5 times) and water (5 mL × 5 times) and was dried under vacuum at room temperature for 24 h. It was reused for the successive reaction cycles.
3 Results and discussion
3.1 Synthesis of the polymer with pendant metal complex
The polyethylene chain having a pendant Schiff-base metal complex was prepared using a three-step synthetic method, which was reported by us previously [14]. A more detailed characterization of the catalyst is included in the following section.
In the first step, commercially available PVC (1) was converted into a polymer carrying pendant primary amino groups (3) using a standard procedure. The reaction is shown in Scheme 1. For this, PVC was treated with an excess amount of ethylene diamine (2) at room temperature. An excess amount of ethylene diamine could prevent cross-linking between different polymer chains as well as the formation of cyclic derivatives by the condensation of the two primary amino groups of single ethylene diamine molecule with neighboring chlorine atoms. After this reaction, the color of the polymer was changed from white to light yellow. The FTIR spectrum of the aminated polymer (3) showed the appearance of peaks at 3350, 3310 and 1590 cm−1, which were assigned to be due to the stretching and bending vibrations of the primary amino groups, respectively. CHN analysis and estimation of the primary amino groups by acid–base titration showed that the polymer contained 5 mmol of NH2 groups per gram of resin.
The aminated polymer (3), on reaction with salicylaldehyde (4) in the presence of catalytic amounts of acetic acid in ethanol under refluxing conditions, gave the Schiff-base of salicylaldehyde attached to the polyethylene backbone (5). The reaction is shown in Scheme 2. The color of the polymer turned from light yellow to dark yellow in the course of the formation of the Schiff-base. FTIR spectra of (5) showed that the peaks corresponding to the primary amino groups in the starting polymer had disappeared and that a new peak appeared at 1680 cm−1 due to CN stretching vibrations. 1H NMR spectra of (5) clearly showed peaks in the aromatic region and a broad peak at 5.2 ppm due to the OH group of the salicylaldehyde moiety (Fig. 1). Elemental analysis also supported the fact that all of the primary amino groups were converted into the Schiff-base.
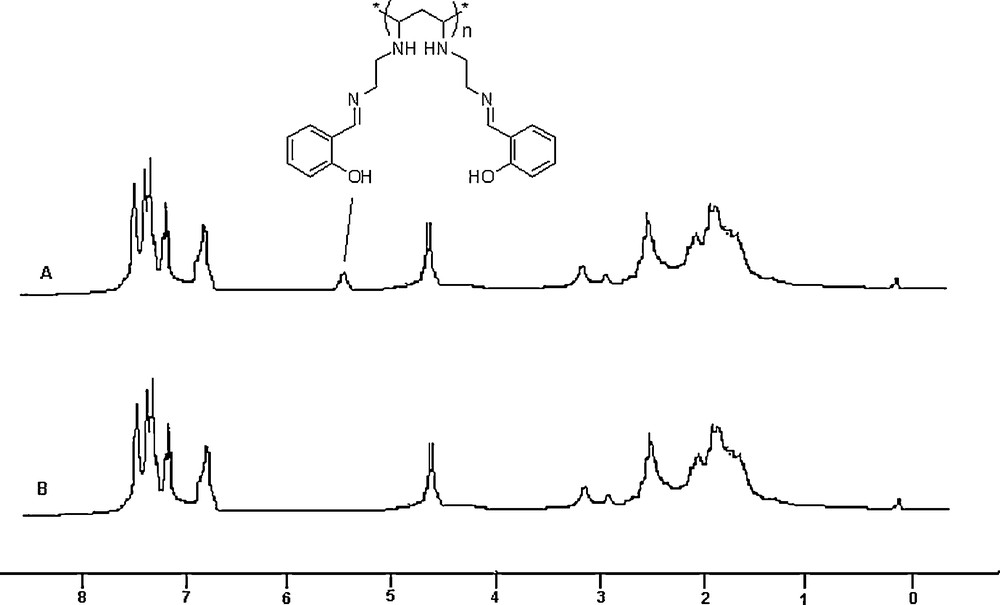
NMR spectra of the polymer-supported Schiff-base before (A) and after (B) formation of the complex.
Reacting the polymer (5) with Cu(CH3COO)2 in methanol under reflux gave the polymer carrying the pendant Schiff-base–Cu(II) complex (6). The reaction is shown in Scheme 3.
Comparison of the FTIR spectra of the polymers (5) and (6) revealed that the peaks corresponding to the hydroxyl group of salicylaldehyde disappeared in the spectrum of (6). Additionally, the peak due to CN stretching underwent a blue shift of 52 cm–1 and occupies a new position at 1642 cm−1 in the case of (6). The appearance of a new band at 635 cm−1 due to the CuN bond also confirmed the formation of the Cu complex [38]. 1H NMR analysis of the polymer (6) (Fig. 1B) showed that the peak corresponding to the phenol group of salicylaldehyde disappeared while the other peaks remained unaltered compared to the spectrum of the polymer (5). The electronic spectra of the complex showed two bands at 422 and 543 nm, which were assigned to the charge-transfer transitions and d–d transitions, respectively. The thermogravimetric analysis showed that the polymer (6) underwent decomposition in the 160–175 °C temperature range, while PVC remained thermally stable up to a temperature of 200 °C. The thermal stability of (6) around 100 °C clearly indicates that there is no water molecules associated with the copper complex. From the thermal analysis data, it was observed that after the formation of the metal-containing pendant group, the thermal stability of the polymer decreased, which might be due to the thermal decomposition of the pendant group. From these analytical data, the structure of the copper complex attached to the polymer backbone is assumed to be a square planar one, as shown in Scheme 3. A SEM image of the polymer (6) is shown on Fig. 2. It clearly indicates a highly coiled structure of the polymer with high surface area. The solubility profile of the polymer (6) showed that it is insoluble in solvents, like water, ethanol and methanol, while it is soluble in chloroform.

SEM image of the polymer-supported catalyst.
3.2 One-pot three-component Mannich reaction catalyzed by the polymer-attached Cu complex
The polymer-attached copper complex (6) was used as a heterogeneous catalyst in the one-pot three-component Mannich reaction between aldehyde (7), amines (8) and ketones (9) to obtain β-amino ketones (10). The Mannich reaction between benzaldehyde, aniline and cyclohexanone was carried out as a model reaction in ethanol. Since the catalyst is insoluble in ethanol, carrying out the reaction in ethanol helps to exploit the advantages of heterogeneous catalysis. Initially, the model reaction was carried out in the presence of various amounts of the catalyst. The results are summarized in Table 1. It can be seen that only 5 mol% of the catalyst is required to get good yield in a short period of time.
Effect of catalyst loading on the Mannich reaction.
Entry | Amount of catalyst (mol% of Cu) | Time (h) | %Yielda,b |
1 | 1 | 24 | 50 |
2 | 2 | 24 | 62 |
3 | 3 | 12 | 65 |
4 | 4 | 12 | 70 |
5 | 5 | 10 | 75 |
a Reaction conditions: 5 mmol of benzaldehyde, 5 mmol of cyclohexanone, 5.2 mmol of aniline, 5 mL of ethanol.
b Isolated yield of the product.
The results of an investigation on the role of solvent in catalysis are summarized in Table 2 and showed that the reaction proceeded quantitatively in solvents, like ethanol, methanol, and chloroform. Since the catalyst is more soluble in CHCl3, it is expected that homogeneous catalysis is more pronounced here. Under such situation, it became difficult to separate the catalyst from the reaction mixture by simple filtration. In other organic solvents, like 1,4-dioxane and THF, the catalyst showed poor performance. The reaction gave quantitative yield when water was used as the solvent. But the catalytic activity of the present polymer-supported complex is lower in water compared to other polymer-supported catalysts reported in the literature [32,33].
Effect of the solvent on catalysis.
Entry | Solvents | %Yielda,b |
1 | Ethanol | 75 |
2 | Methanol | 73 |
3 | Water | 70 |
4 | CHCl3 | 70 |
5 | CHCl2 | 67 |
a Reaction conditions: 5 mmol of benzaldehyde, 5 mmol of cyclohexanone, 5.2 mmol of aniline, 5 mL of solvent, catalyst, 10 h.
b Isolated yield of the product.
In order to prove the efficiency of the catalyst, a family of aldehydes and anilines were used as substrates along with cyclohexanone to synthesize a number of β-amino carbonyl compounds (Scheme 4). The results are summarized in Table 3. The reaction proceeded smoothly within 6 to 10 h to give the products in good yield in the case of all the substrates studied. Aromatic aldehydes, carrying electron-withdrawing groups on the benzene ring, gave good yields. On the other hand, anilines, carrying electron-donating groups on the benzene ring, gave better results, while those carrying electron-withdrawing groups, like NO2 gave comparatively poor yield. Heterocyclic aldehyde, like thiophene aldehyde also gave good yields. All the products were isolated by column chromatography and characterized using FTIR and 1H NMR spectroscopies.
Catalyst recycling was investigated with the model reaction under optimized reaction conditions. After the completion of each reaction cycle, the catalyst was filtered and washed extensively with ethanol and water and dried under vacuum at room temperature. This catalyst was used in the next cycle. It was found that the catalyst remained active up to four reaction cycles without considerable loss of activity. There is a small loss of catalytic activity during the fifth cycle (Table 4).
4 Conclusion
In conclusion, the copper complex of PVC-supported salicylaldehyde Schiff's base was prepared and characterized. This polymer-supported complex was found to be an efficient heterogeneous catalyst in the three-component Mannich reaction between aldehyde, anilines and ketones. Various factors influencing the catalytic activity of the polymer were studied and optimized. A small library of β-keto amines were prepared to prove the generality of the catalysis. The catalyst was found to be reusable.
Acknowledgement
The authors acknowledge the Defence Research and Development Organization, India, for financial support.