1 Introduction
Organic superconductors prepared as single crystals have attracted much attention in the 1980–1990s [1]. For instance, the so-called Bechgaard salts, (TMTSF)2X, have been prepared by electro-oxidation of tetramethyltetraselenafulvalene (TMTSF, Fig. 1) in the presence of an electrolyte, such as [(n-C4H9)4N]X, where X stands for a monovalent inorganic anion (X–: PF6–, AsF6–, ClO4–, ReO4–). Under high current densities (> 60 μA/cm2), needle-shaped crystals of (TMTSF)2ClO4 have been obtained (typical sizes: 10–30 × 0.5–2 × 0.1–1 mm3) [2,3]. Since the 2000s, a new interest has emerged due to the fact that these compounds have been prepared in a more practical usable form, such as thin films or microwires. As an example, (TMTSF)2ClO4 has been grown as microcrystals in a confined environment [4], as thin layers on an organic template [5], as thin films on a (001)-oriented silicon substrate [6], or as micro/nanowires [7].

Molecular formulas for TMTSF and 1-butyl-3-methylimidazolium.
However, at present, spherical nanoparticles of molecule-based conductors are very rare. Indeed, the spherical shape constitutes a “challenging” morphology for compounds, which naturally grow as needles, or wires due to their quasi-one-dimensional character. We have recently obtained roughly spherical nanoparticles of tetrathiafulvalene (TTF)-based conductors protected by a polymer matrix [8], or grown in the presence of an ionic liquid or a long alkyl-chains quaternary ammonium salt [9–11]. In this present communication, we report on the preparation of the first nanoparticles of two Bechgaard salts, namely (TMTSF)2PF6 and (TMTSF)2ClO4.
2 Results and discussion
(TMTSF)2X phases behave as good organic metals with a room temperature conductivity of about 600 S/cm at 300 K (measured along the needle axis). Single crystals of (TMTSF)2PF6 become superconducting at pressures above 6 kbar (critical temperature in the 0.9 − 1.2 K range) whereas those of (TMTSF)2ClO4 are superconducting at ambient pressure (critical temperature between 1.2 and 1.4 K) [1]. As mentioned in the introduction, millimeter-sized single crystals of (TMTSF)2X are grown in an electrochemical cell containing a tetrabutylammonium salt acting as both supporting electrolyte and provider of the X− ion. The absence of stirring is required to get high quality large single crystals for performing structural studies. Ionic liquids containing a 1,3-dialkylimidazolium cation (such as, 1-butyl-3-methylimidazolium BMIM+, Fig. 1) or long alkyl-chains quaternary ammonium salts (such as, [(CH3)(n-C8H17)3N]Cl) in addition to a polar solvent (acetonitrile, acetone) allow the preparation of nanoparticles of TTFCl0.77 [12], TTF·TCNQ [10], or TTF[Ni(dmit)2]2 [11]. In the case of electrochemically grown TTFCl0.77 nanoparticles [12], the ammonium salt plays a triple role: supporting electrolyte, provider of the chlorine counter ion, and entity allowing the control of the growth of the conducting material as nanospheres at the electrode surface.
In this work, we have performed the galvanostatic oxidation (∼ 30 μA/cm2) of TMTSF in the presence of the ionic liquid, [BMIM][PF6], or of the long alkyl-chains quaternary ammonium salt, [(CH3)(n-C8H17)3N]ClO4, as the supporting electrolyte under vigorous stirring. A black solid is obtained on the platinum electrode located in the anodic compartment. Vibrational spectra (infrared and Raman, vide infra) confirm that the powder corresponds to (TMTSF)2PF6 when the electrochemical reaction is performed in the presence of [BMIM][PF6], whereas it corresponds to (TMTSF)2ClO4 when the electrochemical reaction is carried out in the presence of [(CH3)(n-C8H17)3N]ClO4. Infrared and Raman spectra are shown on Figs. 2 and 3, and the corresponding data are gathered in Table 1. The two Raman spectra are very similar and only that for the (TMTSF)2ClO4 powder is shown. Signals are assigned according to references [13–15]. Low intensity features on infrared spectra (in the 3000 − 1300 cm−1 range) are mainly due to the TMTSF molecule (Table 1). C–H stretching modes of the methyl groups are located in the 2920–2840 cm−1 region. These signals confirm unambiguously the presence of the TMTSF moiety within the electrochemically grown powders. Medium and strong intensity signals at lower frequencies are characteristic of the counter ion. Modes for the hexafluorophosphate anion are located at 836 and 556 cm−1, whereas those for the perchlorate anion are located at 1092 and 621 cm−1. Low-frequency Raman peaks (in the 100–1000 cm−1 range) are attributed by the comparison to those for neutral TMTSF [14]. Their positions are not too much affected by the partial charge-borne by the TMTSF molecule. On the contrary, modes assigned to the CC stretches (in the 1450–1600 cm−1 range) display a large shift by comparison to the neutral TMTSF molecule [14,15]. The position of the more intense signal (∼ 1460 cm−1) is in good agreement with a 0.5 formal charge-borne by the TMTSF moiety [15], thus, confirming the 2 TMTSF0.5+/1 PF6− or ClO4− stoichiometry within the powders. For the (TMTSF)2PF6 powder, electron micrographs evidence a majority of roughly spherical nanoparticles, exhibiting diameters in the 20–60 nm range, in addition with some bigger particles (60–75 nm), showing irregular shapes (Fig. 4). For the (TMTSF)2ClO4 powder, nanoparticles exhibit sizes in the 30–75 nm range, most of them being elongated in shape. Thus, the galvanostatic oxidation of the TMTSF molecule dissolved in a mixture of the ionic liquid, [BMIM][PF6], or of the long alkyl-chains quaternary ammonium salt, [(CH3)(n-C8H17)3N]ClO4, and CH2Cl2 provides nanoparticle powders of either (TMTSF)2PF6, or (TMTSF)2ClO4. The literature reports on the preparation of needle-shaped single crystals of (TMTSF)2NbF6 by electro-oxidation of TMTSF dissolved in a mixture of [EMIM][NbF6] (E for ethyl) and CH2Cl2 [16]. In our case, stirring the medium presumably prevent the formation of large single crystals. It also increases the concentration of ionic liquid at the electrode vicinity and favours the growth control of the material as nanoparticles. Moreover, in our case, the use of an imidazolium cation bearing a longer alkyl chain (butyl vs ethyl) is an additional argument to the formation of nanoparticles, as previously reported [9–11]. Furthermore, as stated above, the electro-oxidation of tetrathiafulvalene in the presence of [(CH3)(n-C8H17)3N]Cl affords nanoparticles of TTFCl0.77 [12]. Hence, in the present work, the formation of (TMTSF)2ClO4 as a nanoparticle powder when the electrochemical synthesis is conducted with [(CH3)(n-C8H17)3N]ClO4 is also easily understandable. We can conclude that in a vigorously stirred electrochemical cell, [BMIM][PF6] or [(CH3)(n-C8H17)3N]ClO4 act as the supporting electrolyte, the entity that control the growth as nanoparticles, and the provider of the PF6− or ClO4− anion necessary for the formation of the molecule-based superconductors, i.e., (TMTSF)2PF6, and (TMTSF)2ClO4. (TMTSF)2X (X = PF6, ClO4) nanopowders are soluble in acetonitrile (about 2.5 mg/mL) and insoluble in toluene, hexane, ethanol, tetrahydrofurane, dichloromethane and 1,1,2-trichloroethane. After air exposure (∼ 2 h) and acetonitrile evaporation, infrared spectra and transmission electron micrographs of the solid residue do not exhibit significant changes compared to those of freshly electrodeposited powders. Note that at room temperature, powdered bulk (TMTSF)2PF6 and (TMTSF)2ClO4 have been shown to be soluble in 1,1,2- and 1,1,1-trichloroethane (about 0.2 mg/mL), whereas they are negligibly soluble in tetrahydrofurane or in a toluene/ethanol mixture [17]. Moreover, needle-like (TMTSF)2ClO4 single crystals have been dissolved in acetonitrile at ambient temperature, a further small heating accelerating their solubility [7a]. Therefore, the solubility of our (TMTSF)2X nanopowders in acetonitrile was expected. Furthermore, the good solubility observed for (TMTSF)2X nanopowders in acetonitrile is consistent with that for tetrathiafulvalene- or nickel ethylene tetrathiolate-based conductors as nanoparticles (grown in the presence of a long alkyl chain amine or imidazolium salt), which are very soluble in commonly used polar solvents (tetrahydrofurane, methanol, acetonitrile; 2–5 mg/mL) [18]. Absorption spectra for (TMTSF)2PF6 and (TMTSF)2ClO4 in acetonitrile are almost identical (Table 1), and only that for (TMTSF)2PF6 is shown (Fig. 5). It evidences maxima at 773 nm (∼ 12900 cm−1, strong), 585 nm (∼ 17100 cm−1, weak), and 451 nm (∼ 22200 cm−1, strong). They correspond to intramolecular transitions of the cationic TMTSF moiety [19]. These three characteristic absorptions appear at similar positions for numerous organic conductors [19]. For instance, they are located at ∼ 13500 cm−1 (strong), ∼ 18400 cm−1 (weak), and ∼ 22400 cm−1 (strong) for (BPDT-TTF)3(PF6)2 (BPDT: bis(propylenedithio)tetrathiafulvalene) [20]. Finally, preliminary room temperature conductivity measurements on nanoparticle powders give values of about 0.2 S/cm, of the same order of magnitude than those for bulk powder materials. This bodes that, in the powder, which is actually made of nanoparticles, conduction paths do also exist. For further applications, more interesting would be the deposition of these (TMTSF)2X-based nano-objects onto predefined nanoscale regions of a silicon chip [21]. This study will be soon envisioned. In conclusion, by using a judicious supporting electrolyte, we have prepared the first nanoparticles of the well-known Bechgaard salts. They exhibit sizes in the 20–75 nm range. We are currently investigating the use of different experimental conditions (temperature of the electrochemical growth, potentiostatic conditions) and the addition of a neutral surfactant to the electrolyte solution. Indeed, these latter allow the preparation of very small particles of spin crossover compounds [22]. The preparation of much smaller nanoparticles (≤ 5 nm) than those described in this communication constitutes a challenge for us. This should allow the emergence of a new physics for nano-molecular superconductors, as the recent study of superconductivity in few pairs of (BETS)2GaCl4 (BETS: bis(ethylenedithio)tetraselenafulvalene) molecules on an Ag(111) surface [23].
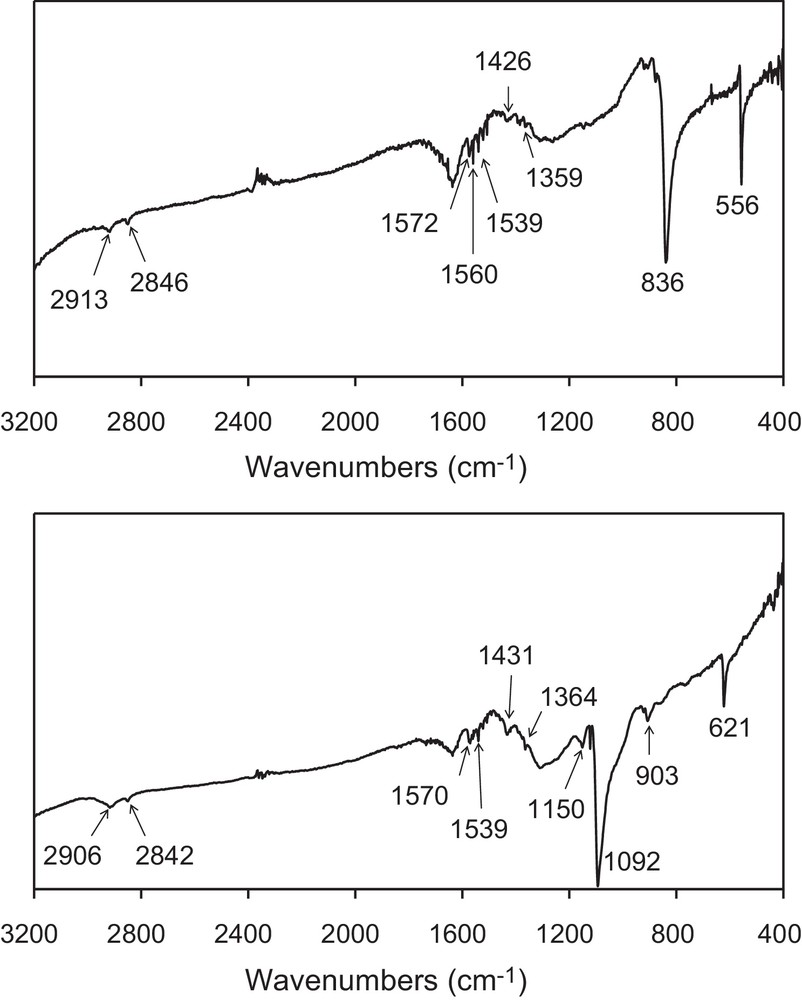
Infrared spectra for (TMTSF)2PF6 (top) and (TMTSF)2ClO4 (bottom) nanoparticles.
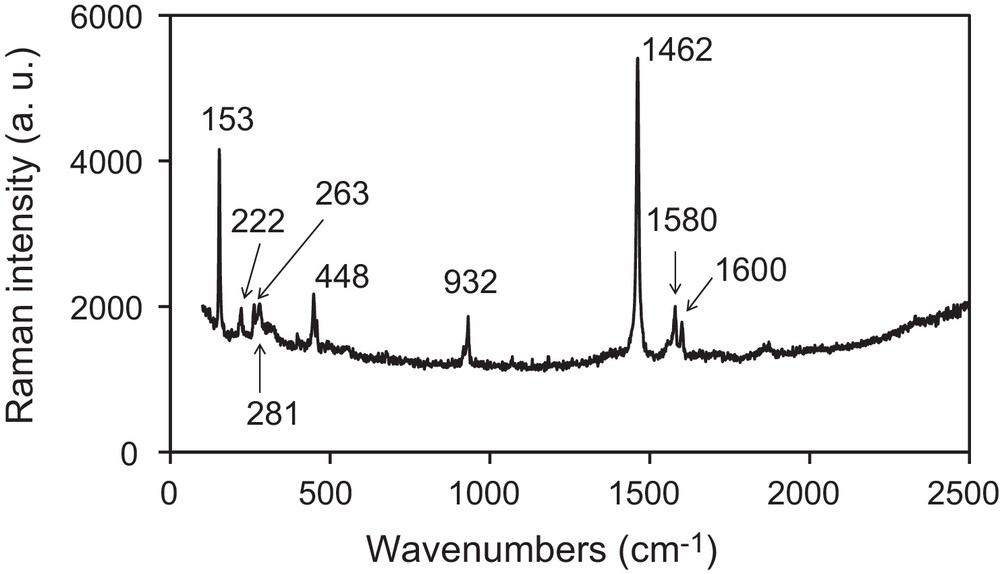
Raman spectrum for (TMTSF)2ClO4 nanoparticles.
Spectral data for nanoparticles of (TMTSF)2PF6 and (TMTSF)2ClO4.
(TMTSF)2PF6 | (TMTSF)2ClO4 | Assignment | |
Infrared spectra, frequencies (cm−1) | 2913 | 2906 | νCH3 asym. |
2846 | 2842 | νCH3 sym. | |
1572 | 1570 | νCC (ν28, b1u) | |
1539 | 1539 | νCC | |
1426 | 1431 | α CH3 asym. | |
1359 | 1364 | α CH3 sym. | |
− | 1092 | ClO4− | |
836 | − | PF6− | |
− | 621 | ClO4− | |
556 | − | PF6− | |
Raman spectra, frequencies (cm−1) | 1601 | 1600 | Peripherical CC stretch (ν3, ag) |
1580 | 1580 | ||
1460 | 1462 | Central CC stretch (ν4, ag) | |
931 | 932 | β CH3 | |
448 | 448 | ν9, ag | |
278 | 281 | ν10, ag | |
262 | 263 | ν11, ag | |
153 | 153 | ν12, ag | |
Absorption spectra, wavelenghts in nm (in brackets, cm−1) | 773 (12,900) | 776 (12,900) | Intramolecular excitations of TMTSF |
585 (17,100) | 589 (17,100) | ||
451 + shoulder at 429 (22,200 + sh. at 23,300) | 451 + shoulder at 431 (22,200 + sh. at 23,300) |
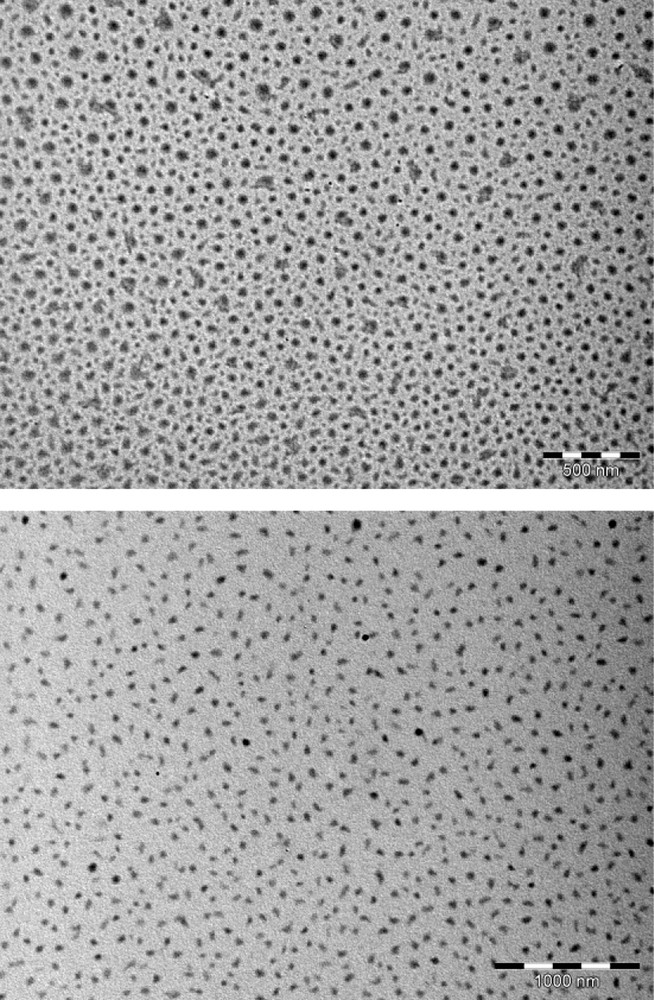
Electron micrographs for (TMTSF)2PF6 (top, bar = 500 nm) and (TMTSF)2ClO4 (bottom, bar = 1000 nm) nanoparticles.
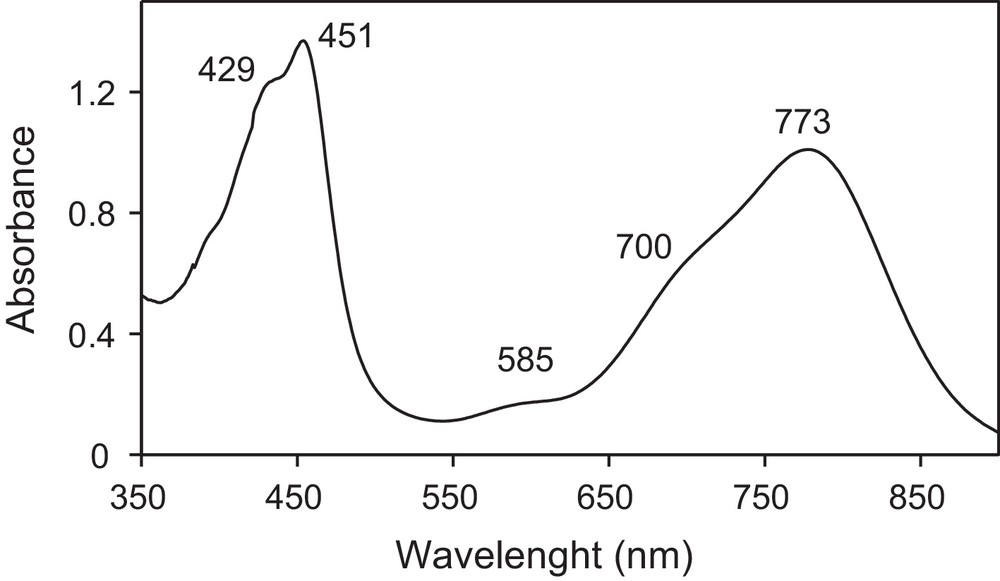
Absorption spectrum for (TMTSF)2PF6 nanoparticles.
3 Experimental
Electrochemical syntheses have been carried out under an argon atmosphere using freshly distilled and degassed dichloromethane. TMTSF is purchased from Aldrich and [BMIM][PF6] from Alfa Aesar. [(CH3)(n-C8H17)3N]ClO4 is prepared as follows: a solution of 160 mg of LiClO4 (1.50 mmol) in 5 mL water is slowly added to a solution of 430 mg of [(CH3)(n-C8H17)3N]Cl (1.07 mmol) in 15 mL of water. The resulting solution is then stirred for 18 h at room temperature. [(CH3)(n-C8H17)3N]ClO4 is extracted by dicholoromethane, dried on MgSO4, and finally, recrystallized from a dicholoromethane/pentane mixture.
A typical electrochemical procedure can be described as follows: nanoparticles synthesis takes place in an H-shaped electrocrystallization cell with a platinum wire (1 cm long; 1 mm diameter) in each compartment, separated by a glass frit in the H cross piece. TMTSF (25 mg; 0.056 mmol), [BMIM][PF6] (80 mg; 0.28 mmol) [or [(CH3)(n-C8H17)3N]ClO4 (130 mg; 0.28 mmol)] and 12 mL dicholoromethane are introduced in the anodic compartment. The same amount of [BMIM][PF6] (i.e. 80 mg; 0.28 mmol) [or [(CH3)(n-C8H17)3N]ClO4 (i.e. 130 mg; 0.28 mmol)] and 12 mL dicholoromethane are introduced in the cathodic compartment, leading to a total molar ratio [BMIM][PF6]/TMTSF [or [(CH3)(n-C8H17)3N]ClO4/TMTSF] of about 10. The synthesis is conducted under galvanostatic conditions (30 μA/cm2) at room temperature in argon atmosphere during 6 days for (TMTSF)2PF6 [or 3 days for (TMTSF)2ClO4]. During the electrochemical synthesis, the content of the cell is vigorously agitated with a magnetic stirrer. The black powder is collected from the anode, washed with 2 × 1.5 mL dichloromethane, and finally dried under vacuum for 24 h (yield: 60% for (TMTSF)2PF6 and 90% for (TMTSF)2ClO4). The black powder is air stable.
The room temperature solubility of the nanopowdered samples in organic solvents is evaluated by the stepwise addition of a determined volume of solvent to the powder under slow stirring (organic solvents: acetonitrile, toluene, hexane, ethanol, tetrahydrofurane, dichloromethane, and 1,1,2-trichloroethane). For transmission electron microscopy observation, powder (0.5 mg) is dispersed in diethyl ether (2 mL) under slow stirring for 1 min. The TEM specimens are then prepared by the evaporation of droplets of suspension deposited on carbon-supported copper grids. The experiments are performed on a JEOL Model JEM 1011 operating at 100 kV. Infrared spectra (in KBr matrix) are taken at room temperature on a Perkin Elmer Spectrum GX spectrophotometer. Raman spectra are recorded on the powder sample at 80 K using a DILOR XY micro-Raman (785 nm laser line). Absorption spectra are taken in the 350–900 nm range on a Perkin Elmer Lambda 35 spectrophotometer (∼ 3 mmol/L in acetonitrile). The room temperature conductivity of the samples is measured on a compressed pellet by two-probe contacts.