1 Introduction
Quinones play a vital role in many biological electron-transfer processes, including respiration and photosynthesis. These compounds are important for chemists, biologists and pharmacologists, as many anticancerous drugs contain quinone functionality [1]. Quinones-hydroquinones provide a prototypical example of redox systems. Electrochemical methods like polarographic, cyclic voltammetric and pulse techniques are being employed to investigate the oxidation/reduction behavior of various quinone systems [2–8]. Due to the fact that a large number of quinones have diverse structures, it is difficult to generalize their action mechanism.
Electrochemical reduction of quinones, including naphthoquinones, has been extensively studied in the past, demonstrating the redox mechanism to depend strongly on the nature of the medium. The results of investigations about the effect of acidic and basic additives reveal a strong influence of hydrogen bonding on different quinone system. The radicals and dianions formed by the reduction of quinones could be stabilized by hydrogen bonding between the anionic oxygen of the quinone moiety and the protons of the phenol functionality present at the α and β positions with respect to quinone oxygen [9]. The electrochemical reduction of juglone, chemically named as 5-hydroxy-1,4-naphthoquinone (HND), has been studied in dimethylsufoxide and acetonitrile both in the presence and in the absence of proton donors [10]. However, the redox behavior of HND in buffered aqueous media is not documented and needs to be investigated, as the properties like antifungal, antiviral and cytotoxicity ones of this class of molecules are attributed to their redox cycling. Therefore, the present work was done to explore the redox behavior of juglone in a wide pH range. The redox behavior of juglone in buffered aqueous media clearly demonstrated a complete switching of the electrode reaction mechanism from CEC to EE, as witnessed by the shift of the reduction potentials back to more positive values, which had never been reported in any of the previous studies on the redox mechanism of quinones/naphthoquinones.
2 Experimental
2.1 Materials and reagents
5-Hydroxynaphthalene-1,4-dione (HND), shown in Scheme 1, was kindly gifted by Prof. Dr. Amin Badshah. Its 2.5 mM stock solution was prepared in analytical-grade ethanol and stored at 25 °C. Working solutions of the compound were prepared with 50% of ethanol and 50% of aqueous supporting electrolytes. All supporting electrolytes whose composition is given in our already published article [11] were prepared using analytical-grade reagents and doubly distilled water. The pH measurements were carried out with a Crison micropH 2001 pH-meter with an Ingold combined glass electrode. All experiments were carried out at room temperature (25 ± 1 °C).
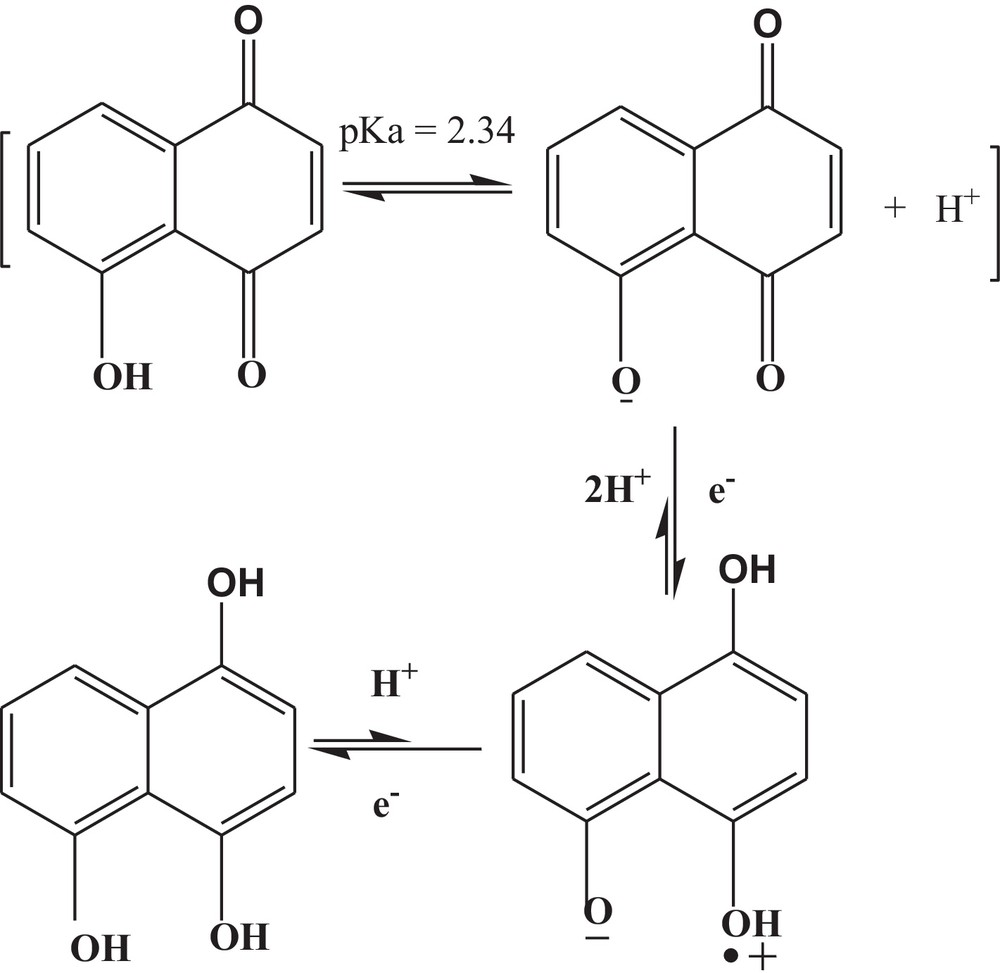
Proposed redox mechanism of 5-hydroxynaphthalene-1,4-dione in the pH range from 1.2 to 5.8.
2.2 Equipments and measurements
Voltammetric experiments were performed using μAutolab running with GPES 4.9 software, Eco-Chemie, The Netherlands. A glassy carbon (GC) with an electroactive area of 0.07 cm2 was used as the working electrode. A Pt wire and a saturated calomel electrode (SCE) were employed as counter- and reference electrodes. Before each experiment, the surface of GCE was polished with diamond powder followed by thorough rinsing with distilled water. All the voltammetric experiments were conducted in a high-purity argon atmosphere. For density functional theory (DFT) optimization and energy calculations, GAUSSIAN 03 W software package was used. An internal default criterion of GAUSSIAN 03 W was used. The computations were performed on P-4 computer.
3 Results and discussion
3.1 Cyclic voltammetry
The cyclic voltammetric response of a 1 mM solution of HND at a clean GCE was investigated in a medium buffered at pH 7.2 between the limits ± 1.5 V (Fig. not shown). Three reduction peaks located at –0.458, –0.956 and –1.25 V and one oxidation signal at –0.091 V were noticed in the cyclic voltammogram of HND. No peak in the positive realm was registered under these conditions, hence, further experimentations were limited to the range of 0.3 to –1.5 V using a starting potential of 0 V (Fig. 1). HND oxidation was not evidenced on GCE due to the possible involvement of –OH group in intramolecular hydrogen bonding with the quinone oxygen of the adjacent ring. From peak clipping experiments, it was established that peak 1a corresponds to peak 1c, while peaks 2c and 3c were found to be due to the reduction of the reduction product of the first cathodic step, giving a cascade mechanism. The blank voltammogram of the solvent system was also recorded for verification of the voltammetric signals to be those of the analyte only (Fig. 1). The extent of adsorption on the electrode surface during the scan was checked by taking successive scans of the same solution without cleaning the electrode surface. The results showed almost no adsorption for the oxidation of the first reduction peaks. However, the second and third reduction peaks were found to be associated with the adsorption phenomenon. In spite of the adsorption in the second and third reduction peaks, there was still the appearance of the signal up to the fifth scan, showing physisorption rather than chemisorptions, as is apparent from Fig. 2. The non-adsorptive character of the first cathodic peak and the adsorptive nature of the second and third peaks explain the difference in their current intensities from one scan to the other.

First CV scan of a 1 mM 5-hydroxynaphthalene-1,4-dione solution overlaid with the blank voltammogram of solvent (50% ethanol and 50% 0.2 M phosphate buffer of pH 7.2) at ν = 100 mV s−1. For interpretation of colors, see the online version of this article.

Successive CVs (scans 1–5) of a 1 mM 5-hydroxynaphthalene-1,4-dione solution recorded in a medium buffered at pH 7.2 and at ν = 100 mV s−1. For interpretation of colors, see the online version of this article.
Further CV studies of HND were carried out in different electrolytes with pHs ranging from 1.2 to 12.7 (Fig. 3). The voltammetric behavior was found to depend strongly on the pH of the medium. In the pH range 1.2–5.8, two reduction peaks were observed in the CVs of HND. Epc showed a drift towards more negative values with rising pHs, indicating that the reduction becomes more and more difficult owing to a lower concentration of H+ ions [12]. Change of pH from 5.8 to 7.2 was accompanied with the appearance of a third reduction peak at a more negative potential. This peak was observable up to pH 12.7 and its potential did not change with pH, indicating that no proton was involved in this cathodic step. Switching pH from 5.8 to 7.2 was accompanied by the dislocation of the anodic signal from –0.349 to –0.088 V and the shifting back of both first and second reduction signals to the anodic side, indicating a mechanism change in neutral conditions. In alkaline media, the first cathodic peak potential remained independent of pH, while the oxidation signal stopped drifting at pH 10.1. The Epc1 vs. pH plot (Fig. S-1A), with a slope of 101 mV pH−1, demonstrated the involvement of an unequal number of electrons and protons in the reduction process. The pKa value showing chemical protonation–deprotonation was found to be 6.5 for the reduction process. The oxidation signal was also observed to display a negative shift as the pH is increased, proving the corresponding ease of oxidation in high pH conditions. Epa vs. pH plots (Fig. S-1B) having two linear segments in the ranges 1.2–5.8 and 7.4–10.1 gave two pKas values of 6.5 and 9.9. CVs of 1 mM HND were also obtained at different scan rates at pH 4.1 using 0.1 M acetate buffers (Fig. 5A). By increasing the scan rate, the potential of the reduction peaks was slightly displaced to more negative values and, correspondingly, the oxidation peak shifted to more positive value. The difference between peak potential Epc1 and the potential at half peak height Epc1/2 of 71 mV indicated the reduction processes to be quasi-reversible in nature. The cathodic shifting of Epc1 with raising the scan rate also evidenced the quasi-reversibility of the process. By increasing the scan rate, the current intensities of all peaks varied linearly with the square root of ν, which is consistent with a diffusion-controlled process of solution species (Fig. 5B). The slope of the log Ipc1 vs. log scan rate plot, with a value of 0.6 (close to the theoretical value of 0.5), also evidenced the reduction process to be diffusion-limited.
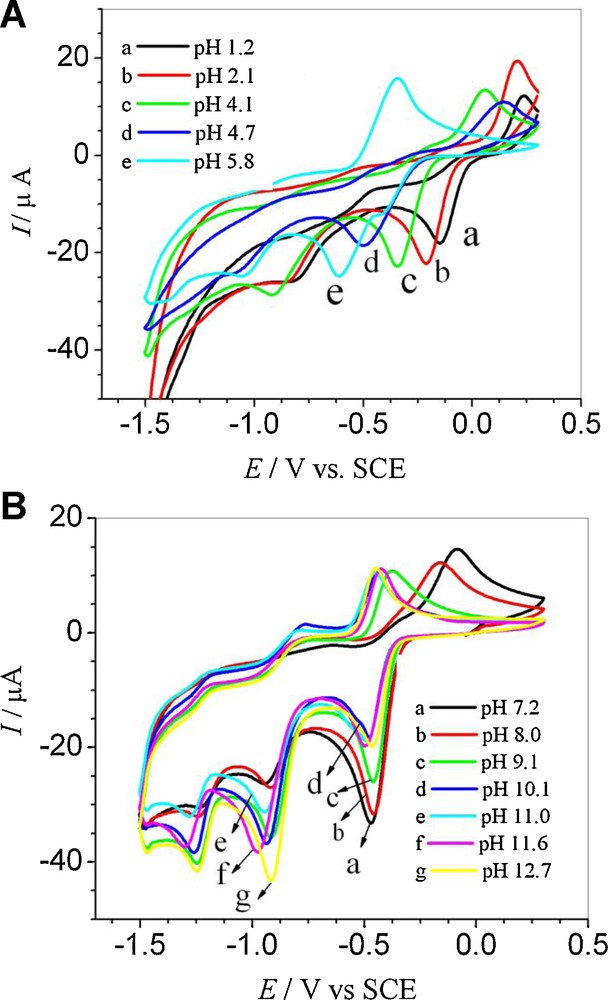
(A) CVs of a 1 mM 5-hydroxynaphthalene-1,4-dione solution recorded at ν = 100 mV s−1 in different supporting electrolytes of pH from 1.2 to 5.8 and (B) from 7.2 to 12.7. For interpretation of colors, see the online version of this article.

(A) Overlaid electronic absorption spectra of 50 μM 5-hydroxynaphthalene-1,4-dione (HND) and PMND obtained at pH 7.4. (B) Absorbance vs. pH plot of HND at 250 nm. For interpretation of colors, see the online version of this article.
By plotting Ipc1 vs. ν1/2, the value of the diffusion coefficient was evaluated by using the equation:
(1) |
(2) |
3.2 Differential pulse voltammetry (DPV)
DP voltammograms of 0.5 mM HND depicted in Fig. 4A were recorded in supporting electrolytes of different pHs. The shift of the peak potential with a rise in pH displayed features quite similar to cyclic voltammetry results. The appearance of two reduction peaks in acidic media and three reduction peaks in neutral and basic conditions supported the results obtained from CV. The number of electron/s (n) involved in the redox process was evaluated from the width at half peak height (W1/2) according to the equation: W1/2 = 90.4/n.

(A) DPVs of 0.5 mM 5-hydroxynaphthalene-1,4-dione (HND) obtained in media of different pHs at ν = 10 mV s−1 (B) SW voltammograms of 0.5 mM HND recorded in a medium buffered at pH 7.2. For interpretation of colors, see the online version of this article.
3.3 SQW voltammetry
The square wave voltammetry (SWV) excels other electrochemical techniques due to its greater speed of analysis, a little consumption of the electroactive species in comparison to DPV and reduced problems with poisoning of the electrode surface. Another advantage of SWV is that the reversibility of the electron transfer process can be checked during one scan only. Since the current is sampled simultaneously in both positive and negative-going pulses, peaks corresponding to oxidation and reduction of the electroactive species at the electrode surface can be obtained in the same experiment. The main objective of SWV is to ensure the reversibility, irreversibility, or quasi-reversibility of the redox process of the analyte.
SWV of 0.5 mM HND was performed in solutions buffered at pHs 4.2 and 7.4. The appearance of two reduction peaks in acidic medium and three at neutral pH authenticated the CV and DPV results. SQW verified the quasi-reversible nature of the first reduction process as the ratio of forward and backward peak currents was not equal to 1 at neutral pHs. However, the ratio of 1.01 at pH 4.2 indicated the reversible nature of the first reduction step. The second reduction peak was reversible at both pH 4.2 and 7.4, while the third one at 7.4 was found irreversible, having no corresponding oxidation peak (Fig. 4B).
3.4 Proposed electrode reaction mechanism
From the cyclic voltammetric results, the slope Epc1 vs. pH plot of 101 mV in the pH range 1.2–5.8 predicted the reduction of the analyte by two protons and a single electron. The participation of one electron in the first reduction peak (appeared in DPV) was determined from the W1/2 value of 91 mV. The appearance of a second cathodic peak, Epc2, showed the addition of another electron to the reduction product, while the slope of 50 mV per pH unit of the Epc2 vs. pH plot indicated the involvement of one electron and one proton.
Literature survey reveals that reduction of naphthoquinones occurs by two electrons and two protons, which may take place in a single step or by a stepwise transfer of electrons depending upon the solvent system used. In protic media, a single reduction peak is observed, corresponding to the transfer of two electrons and of two protons [9], whereas in aprotic media, electron transfer takes place in steps, giving two reduction peaks. The second reduction peak appears at more negative potentials since the addition of a second electron to the negatively charged species is difficult [13,14]. In the case of HND, a stepwise reduction was observed as compared to single-step reduction because of the hydroxyl group attached to the quinone framework at the α position. The hydroxyl group at α and/or β position(s) of quinones has a pronounced effect on the reduction potential, which makes such compounds different from other substituted quinones.
The shift of the peak potential of α and β phenolic semiquinones and quinone dianions to more positive values has been attributed to intramolecular hydrogen bonding by previous researchers [15,16]. Thus, in HND, the intermediate semiquinone is stabilized by intramolecular hydrogen bonding, which results in two separate cathodic peaks with one at lower and the second at a significantly higher negative potential. Therefore, a behavior (i.e. two-step reduction process) similar to that of the aprotic medium is observed in protic solvent.
The semiquinone radical can abstract one proton from the phenolic group, justifying the addition of two protons and one electron, as evidenced from the slope of 101 mV pH−1. The work reported on 5,8-dihydroxy naphthoquinone shows that, due to intramolecular hydrogen bonding, external protonation does not occur [17,18]; hence there must be internal protonation of semiquinone (Scheme 1).
Under neutral conditions, the peak potential becomes independent of pH, indicating the involvement of electrons only. This theoretically predicts the stepwise addition of electrons, giving a semiquinone anion and a quinone dianion for the first and second reduction peaks, respectively. Changing the medium from acidic to neutral pH, a third cathodic peak appears, which corresponds to the reduction of the second cathodic product to a radical anion stabilized by intramolecular hydrogen bonding (Scheme 2). The formation of such trivalent anion and the subsequent reduction of the starting molecule has also been reported by Guin et al. [2].

Proposed redox mechanism of 5-hydroxynaphthalene-1,4-dione in the pH range from 7.4 to 12.7.
The shift of Epc to a more positive value at neutral pH is indicative of a switching of the mechanism from CEC (addition of electron preceded and proceeded by proton transfer) to EE (stepwise addition of electron). Similar switching of the reaction mechanism in case of 2-((prop-1-enyloxy)methyl)naphthalene-1,4-dione is also reported in our published article [19]. The pKa of the reduced HND was found to be at pH 6.5 for the first and second reduction peaks. The equilibrium of the first reduction product is displayed in Scheme 3. The product of the second reduction step also gave the same pKa value, showing a similar acid–base equilibrium.

Acid–base equilibrium of 5-hydroxynaphthalene-1,4-dione.
3.5 Computational study
Computational studies of HND and its structural analogue 5,8-dihydroxynaphthalene-1,4-dione (DND) were carried out in order to support the results obtained from CV. For this purpose, DFT calculations were done for the determination of EHOMO and ELUMO of the compounds. The possible oxidation and/or reduction site(s) of the compounds were predicted from the charge densities and values of EHOMO and ELUMO (Fig. S-2 and Table 1). The DFT studies of HND and DND showed the same trend of their reduction potentials, as obtained from cyclic voltammetry. Reduction of DND was found more facile in comparison to HND, as is obvious from its less negative potential (Fig. S-3). The graphical representation of their LUMO can be seen in Fig. S-4. A more negative ELUMO value signifies an easier uptake of electrons as the orbitals’ energy is decreased.
EHOMO and ELUMO values of HND and DND obtained through density functional theory (DFT).
Compound | EHOMO | ELUMO |
5-hydroxynaphthalene-1,4-dione (HND) | –0.24352 | –0.11031 |
5,8-dihydroxynaphthalene-1,4-dione (DND) | –0.23082 | –0.13785 |
3.6 UV-Visible spectroscopy
HND or juglone is used as a dye and display a color change with the pH of the medium. Its electronic absorption spectroscopy analysis was carried out in the 1.0–11.6 pH range. UV-Visible spectra of HND showed a less intense band at 340 nm and a rather fine structure with maxima at 245 and 250 nm. A shoulder next to this fine structure is present at 260 nm. A literature survey of the absorbance spectroscopy of naphthoquinones and their derivatives reveals that a pair of peaks at 250 and 245 nm corresponds to the benzene moiety [20]. A hump at 260 nm is due to the quinonoid (π→π* transition) structure. A band at 340 nm could be due to the n→π* transition, as it represents a low-energy and less intense transition in comparison to π→π* transitions [21]. It is thought to be associated with the ring structure, as in the reported 1,4-naphthoquinone derivatives [20].
Absorption spectra of juglone in various pH media are represented in Fig. S-5. The pH increment from 1.0 to 6.0 exhibited a decrease in the absorption intensity accompanied with a slight bathochromic shift of all the bands. These peculiar spectral characteristics can be attributed to the interaction of the polar solvent with the excited state of the analyte. Absorption spectra in the pH range between 7.2 and 11.6 displayed an increase in absorbance, which might be due to the fact that alkaline pH values support the deprotonated form of HND and the negative charge on oxygen causes an increase in the intensity of the n→π* and π→π* transitions, as the negative charge enriches the electron density on the ring. The peak at 250 nm was selected as the analytical wavelength for the determination of the pKa value of the phenolic group, due to the pronounced variation in absorbance occurring at this wavelength.
Comparison of the absorption spectra HND with a closely structurally related vitamin K derivative, 2-((prop-1-enyloxy)methyl)naphthalene-1,4-dione, aided to its characterization. The absorption spectra are quite similar, except that there is a bathochromic shift in the second band of 2-((prop-1-enyloxy)methyl)naphthalene-1,4-dione molecule, as illustrated in Fig. 5A. The band is assigned to the π→π* transitions of the quinoid structure. A shift toward longer wavelength is due to the electron-donating nature of the side-group, increasing the electronic density of the ring and thus decreasing the energy of excitation.
To determine the pKa of HND, absorbance vs. pH data was plotted at 250 nm (as a maximum absorbance change was observed at this wavelength). Analysis of Fig. 5B shows a continuous decrease of the shoulder peak at 250 nm from pH 1.0 to pH 6.0, with a slight bathochromic shift indicating that deprotonation must be taking place within this pH range. Hence, such a pH range was selected for the determination of the pKa of the phenolic group. A pKa value of 2.34 was obtained from the graph of absorbance vs. pH. However, literature survey reveals that juglone has a pKa value of 3.65 [22]. The discrepancy can be attributed to solvent effects [23].
4 Conclusion
The voltammetric behavior of α phenolic naphthoquinones in buffered aqueous media was found quite different from usual protic and aprotic solvents. HND was found to reduce in two steps (as witnessed by the appearance of two cathodic peaks) under acidic conditions and three steps (as validated by the generation of three cathodic peaks) in neutral and alkaline media. The different variation of Ep vs. pH of anodic and cathodic peaks established the non-reversible nature of the overall electrochemical process. One pKa for reduction (6.5) and two pKas (6.5 and 9.9) for oxidation were evaluated from Ep vs. pH plots. The results obtained from computational studies were found to be in good agreement with experimental findings. The results are expected to offer a plausible explanation to the reduction mechanism of juglone in protic media, thus throwing light on the action mechanism of this class of compounds in biological systems where their properties are completely attributed to redox cycling. DFT calculations supported the results obtained from cyclic voltammetry. HND was also characterized by UV-Visible spectroscopy and the attribution of the signals was strengthened by the comparison of its spectrum with a closely related compound, 2-((prop-1-enyloxy)methyl)naphthalene-1,4-dione. A pKa value of 2.34 for HND was evaluated from the effect of pH on its electronic absorption spectrum.
Acknowledgments
The authors gratefully acknowledge the funds provided by Quaid-i-Azam University and the Higher Education Commission of Islamabad, Pakistan, for supporting this work.