1 Introduction
The transformation of methanol to hydrocarbons (MTH) to produce high-octane gasoline [1] represents a valuable process involving zeolite or zeotype catalysts, which received considerable industrial interest [2–5]. Besides the Mobil Oil methanol-to-gasoline (MTG) process, Lurgi's methanol-to-propene (MTP) [6], Norsk Hydro/UOP's methanol-to-olefins (MTO) and Haldor Topsøe TIGAS processes [7] are also commercialized [8,9].
The MTO reaction is a competitive option for the valorization of stranded gas reserves. Several studies devoted either to the reaction mechanism or to the technology were therefore undertaken [9–17]. In addition, the worldwide market demand in ethylene and propylene is gradually raising and projected growth rates in coming decades are further expected to remain high [18]. ZSM-5 zeolite catalysts are often employed and several strategies targeting to achieve an improved selectivity toward light olefins have been tempted: operating conditions, change in reactor configuration [19], proper tailoring of catalyst Brønsted acidity [20], development of structured catalyst [12,21].
We have recently investigated the influence of both Brønsted acid sites density and crystal size on activity/selectivity in the MTO reaction [22]. The main goal was to raise and then maintain a high selectivity toward propylene, hence to develop a simple strategy for designing a MTP catalyst with the MFI structure. With these promising results in hand, it was decided to further optimize ZSM-5 zeolites synthesized under fluoride conditions. Indeed, hydrofluoric acid has to be replaced to warrant industrial catalyst implementation. This study will therefore focus on the design of a novel MTP-catalyst generation, synthesized in the absence of HF.
2 Experimental
2.1 Catalyst preparation
Several ZSM-5 zeolites were synthesized via fluoride-mediated syntheses to investigate different parameters: density of Brønsted acid sites, crystal size, chemical composition. One ZSM-5 zeolite prepared via the classical alkaline route was used as the reference material and named MFI-R [22]. MFI-F catalyst was prepared via the fluoride-mediated route according to our previous studies [23]. The same procedure was followed while substituting hydrofluoric acid by either hydrochloric acid or phosphoric acid to maintain a neutral pH (Table 1). These samples were named MFI-FCl and MFI-FP, respectively. All ZSM-5 samples obtained in either NH4- or Na-form were either ion-exchanged and calcined or simply calcined. Cationic exchange was repeated three times, using 20 mL of a 1.0 M aqueous NH4NO3 solution per gram of catalyst. The solution was kept at 348 K for 2 h before catalyst separation by centrifugation. The solid was dried overnight in an oven at 373 K and further calcined in static air during 6 h at 823 K.
Textural properties and catalytic performances of as-prepared catalysts.
Entry | Catalyst | pH-gel | Si/Al/(P)-gel | MeOH conversiona [%[ | SC2b [%] | SC3b [%[ | SC2–C4b [%[ |
1 | H-ZSM-5R | 10 | 25 | 14 | 15 | 27 | 56 |
2 | H-ZSM-5F | 6–7 | 67 | 98 | 7 | 36 | 66 |
3 | H-ZSM-5FCl | 6–7 | 100 | 64 | 26 | 35 | 78 |
4 | H-ZSM-5FP | 6–7 | 100/1/11 | 0 | – | – | – |
5 | H-ZSM-5FP | 7 | 100/1/1 | 13 | 33 | 35 | 83 |
6 | H-ZSM-5FP | 7 | 100/3/2 | 54 | 32 | 33 | 78 |
a After 48 h on stream.
b After 15 min on stream at complete methanol conversion.
2.2 Characterization
Specific surface areas (SSA) of the materials were determined by N2 adsorption-desorption measurements performed at 77 K employing the BET-method (Micromeritics sorptometer Tri Star 3000). X-ray diffraction (XRD) patterns were recorded on a Bruker D8 Advance diffractometer, with a Ni detector side filtered Cu Kα radiation (1.5406 Å) over a 2θ range of 5° to 60°. Scanning electron microscopy (SEM) micrographs were acquired on a JEOL FEG 6700F microscope working at a 9 kV accelerating voltage. The Si/Al ratios of the materials were determined by EDX analysis coupled with the SEM chamber.
Infrared spectroscopy (FTIR) spectra were recorded in controlled atmosphere at 2 cm−1 resolution on a Bruker Vertex 80 FTIR spectrophotometer, equipped with a MCT detector and using a homemade IR cell. FTIR spectra were collected in transmission mode on a thin film prepared by deposition of a zeolite water suspension on a silicon wafer. The difference in the Brønsted acid strength of these samples was derived from CO adsorption. In order to compare the IR band intensities, spectra were normalized to the overtone mode at 2005 cm−1.
2.3 MTO reaction
Prior to use, the catalysts were activated at 823 K with a gradient of 15 K/min in static air. The zeolites were sieved and particles < 250 μm were used in the following experiments. Sixty milligrams of zeolite were introduced in a tubular quartz reactor and packed between quartz wool plugs. A constant nitrogen flow, of 20 mL/min was flown through a methanol saturator, cooled at 273 K, to achieve a WHSV = 1.12 gMeOH/gcat * 1/h. The reactant was subsequently fed to the reactor containing the catalyst at 673 K. GC analysis was performed on a HP GC5890 equipped with a 50-m capillary column (PONA) and a flame ionization detector (FID). The activity of the catalysts was expressed in terms of methanol and dimethylether conversion, calculated from the difference between inlet and outlet concentrations of methanol (DME is considered as an intermediate). The selectivity was defined as the mole ratio of each product referred to the moles of converted methanol and dimethylether. Eqs. (1) and (2) were used to calculate the (1) conversion, (2) the respective selectivity and (3) the C3/C2 ratio:
(1) |
(2) |
(3) |
3 Results
3.1 Characterization of the catalysts
XRD patterns of all as-prepared zeolites exhibited the sole MFI structure. Fig. 1 shows the pattern of the MFI-F sample.
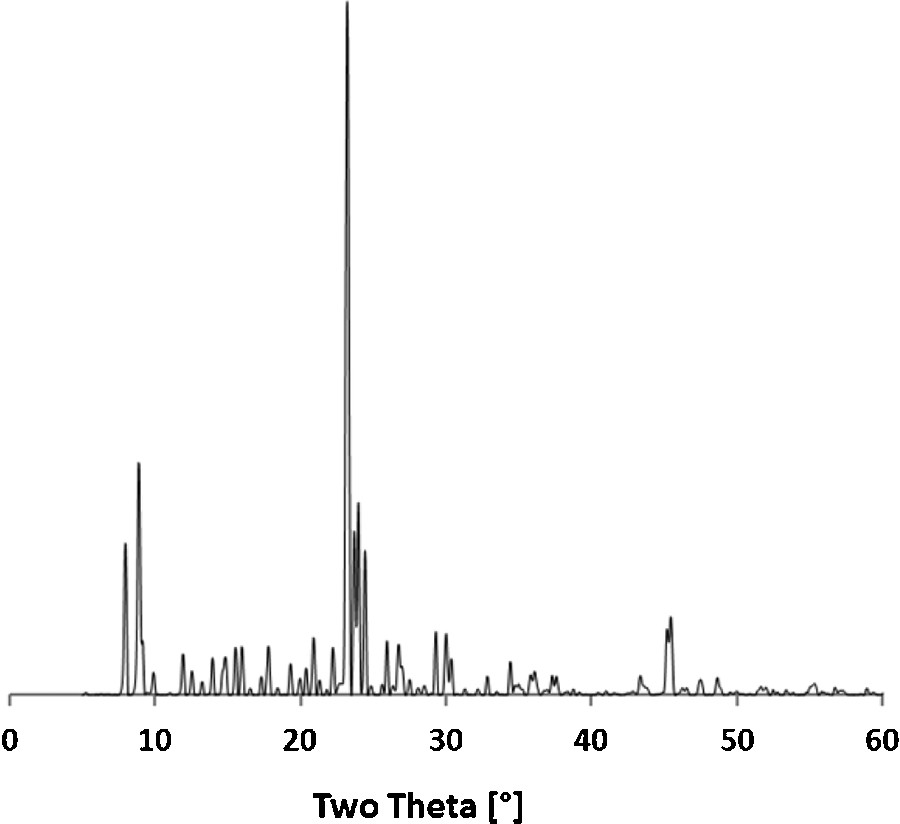
X-ray diffraction pattern of MFI-F zeolite.
SEM analysis was performed to evaluate the crystal morphologies and determine the Si/Al ratios of the different catalysts (EDX analysis). MFI-R exhibited the classical coffin-shape morphology, along with a crystal size of 5 μm. The MFI-F material prepared via fluoride-mediated route led to the formation of giant prismatic crystals having ∼ 15–20 μm in size (Fig. 2a). This material exhibits a very high cristallinity (Figs. 1 and 2a). The two samples synthesized in the absence of HF, MFI-FP (Fig. 2b) and MFI-FCl (Fig. 2c) also exhibit large prismatic crystals ∼ 20–30 μm, along with the presence of amorphous material.
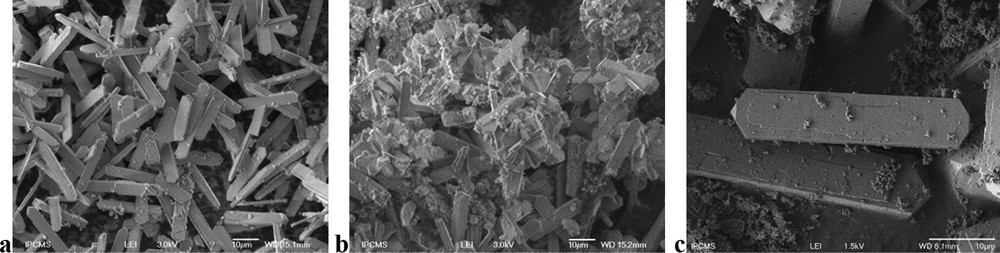
Scanning electron microscopy images of fluoride-mediated materials: a: MFI-F zeolites synthesized in pure fluoride medium; b: MFI-FP in the presence of phosphoric acid; c: MFI-FCl in the presence of hydrochloric acid.
Table 1 presents the Si/Al ratio and the pH of the syntheses as well as reactivity data. The specific surface areas were comprised for all zeolites between 305 and 348 m2/g in line with values usually observed for MFI-type zeolites.
A three-time higher density of BrØnsted acid sites was measured by H/D exchange technique [24,25] over MFI-R when compared with MFI-F zeolite, i.e; 1.00 and 0.31 mmol H+/g, respectively [22]. This result is in agreement with a higher Si/Al ratio observed for MFI-F samples (Table 1). Fig. 3 presents the IR spectra of MFI-F zeolite measured during interaction with CO at 77 K. The peak attributed to the Brønsted acid sites (3610 cm−1) was red shifted to 3305 cm−1 for MFI-F and MFI-R samples upon interaction with CO, thus indicating similar acid strength. The weak signal at 3750 cm−1 indicates that only few defects (silanols) are present in MFI-F sample. When considering the ν(C–O) region (2050–2250 cm−1), the main peak observed at 2174 cm−1 is attributed to an interaction with the Si–OH–Al bridging sites. Furthermore, a significant vibration centered at 2169 cm−1, attributed to CO interaction with extra-framework Al (EFAl) sites, was also observed.

Infrared spectroscopy (FTIR) spectrum of MFI-F pretreated at 873 K for 1 h, following CO adsorption at 77 K. Left panel: the ν(OH) region; right panel: the ν(CO) region. The CO coverage decreases successively from bold gray curve to light gray curve in the spectrum.
3.2 Methanol-to-olefins reaction
Whereas a continuous and drastic decrease in methanol conversion was observed over MFI-R reference catalyst, MFI-F catalyst exhibited a high stability with time on stream [22]. Indeed, the initial methanol full conversion diminished to 20% after 48 h on stream for MFI-R zeolite (Table 1). Besides, only minor changes in product selectivities were observed during the course of the reaction. The propylene-to-ethylene ratio was maintained between 2 and 2.5. In contrast, MFI-F catalyst preserved a complete methanol conversion during the whole test along with the same selectivities toward the reaction products (Table 1).
A high propylene-to-ethylene ratio C3/C2 = 5.5 was achieved and kept constant during 48 h on stream. The global selectivity in propylene was nearly 40%, being thus the major product obtained (Table 1). We have therefore decided to vary the weight hourly space velocity (WHSV) in order to further improve the C3=/C2= ratio for this catalyst. Fig. 4 presents the methanol conversion and the C3=/C2= ratio as a function of MFI-F catalyst weight at constant gas feed.
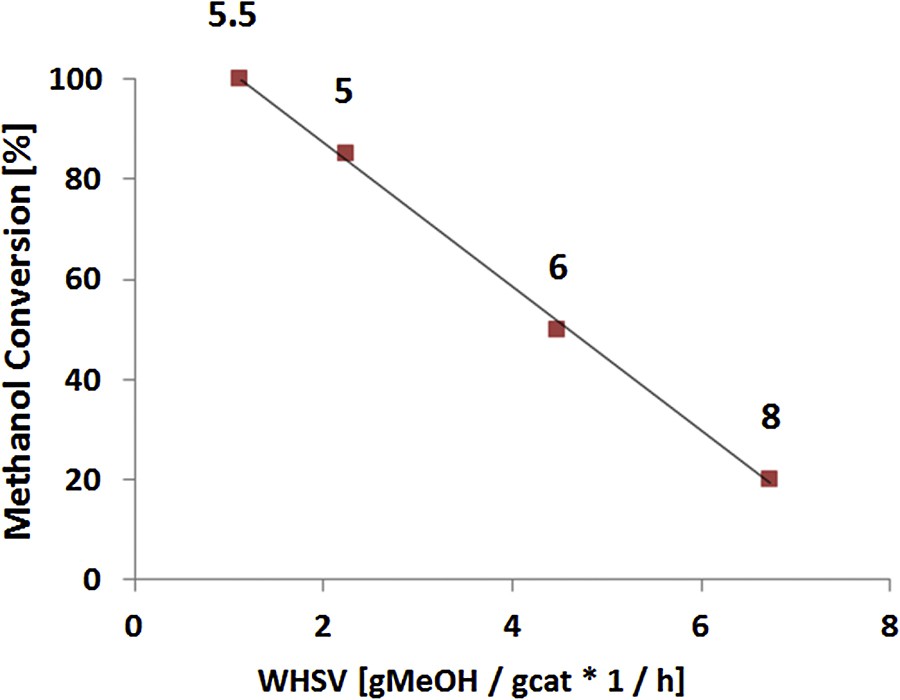
Methanol conversion and propylene/ethylene ratio as a function of weight hourly space velocity for MFI-F catalyst (numbers indicate the propylene/ethylene ratio).
In parallel to a decrease in methanol conversion, it is worthy to note that a six-time raise in the WHSV led to a further enhancement of the propylene-to-ethylene ratio up to 8, albeit at the expense of methanol conversion. The global selectivity in propylene was kept constant at 41%. The major difference between this catalyst (prepared in neutral medium) and the reference catalyst relies on its lower density of Brønsted acid sites. Indeed, less sites are present within these rather giant crystals, thus raising the dispersion of the active sites.
After thorough investigations of promising MFI-F catalyst, we have decided to improve the synthesis methodology by avoiding hydrofluoric acid use. The strategy was to test conventional hydrochloric acid on the one hand and phosphoric acid on the other hand to see whether P may have or not an influence on the catalyst performance, as already mentioned by Hua et al. [20].
Figs. 5 and 6 present the catalytic profiles, methanol conversion and selectivity to light olefins for MFI-FCl and MFI-FP zeolites.

Conversion and selectivities toward ethylene and propylene for MFI-FCl catalyst.

Conversion and selectivities toward ethylene and propylene for two different MFI-FP-catalysts: a: same Si/Al ratio than MFI-F catalyst; b: higher Al content.
As already seen in Table 1, MFI-FCl zeolite allows achieving the same selectivity toward propylene as MFI-F catalyst. In contrast, the propylene-to-ethylene ratio remains rather low at ∼ 1.3. MFI-FCl catalyst remained active during two days on stream (at least). The single substitution of hydrofluoric acid did not drastically alter the catalyst's properties.
Regarding the substitution by phosphoric acid (neutral), a non-competitive MTO-catalyst was produced. Indeed, a continuous and drastic loss in methanol conversion was observed (Fig. 6a). However, by properly tailoring Si/Al/P elemental composition, a competitive catalyst could be prepared (Entry 6, Table 1). This P-containing MFI zeolite exhibited a very slow deactivation and high ethylene selectivity (32%) with a propylene-to-ethylene ratio = 1. It is therefore worthy to mention that the modulation of P/Al/Si in the gel for preparing ZSM-5 zeolites is a critical parameter to design a powerful MTO-catalyst. XRF elemental analysis of the latter H-ZSM-5FP confirmed the presence of P in the zeolite after calcination, i.e. P/Al = 0.24. Whereas no chlorine could be detected after MFI-FCl calcination, nearly one P-atom is present per 4 Al-atoms in more stable H-ZSM-5FP zeolite for the MTO reaction.
To summarize, it is therefore possible to avoid HF use for preparing valuable MTO-catalysts using less harmful HCl or H3PO4 acids.
Besides environmental concerns, a proper combination of Si/Al/P elements in the precursor gel is necessary to achieve a high stability and to maintain a high selectivity toward C2–C4 olefins. Further studies are under progress to evaluate the possible neutralization of acid sites by integration of phosphate tetrahedra within the zeolite framework. Furthermore, this generation of P-catalyst exhibits a high ethylene selectivity, which in turn supports the hypothesis of phosphate integration in the porous network, thus leading to higher steric constraints [20]. Likewise silicoaluminophosphates [26], a possible insertion of phosphate in MFI zeolite crystalline structure could lead to a different behaviour in acid-catalyzed reactions. Hence, MFI-FP type catalysts will be further investigated to see whether P-atoms are integrated or not in the framework by solid-state 31P MAS NMR and EXAFS studies.
4 Conclusion
A novel generation of promising MTO-catalysts was successfully developed by adapting the ZSM-5 zeolite preparation procedure, along with tailored BrØnsted acidity and crystal size.
High propylene-to-ethylene ratios (> 5) were achieved over a ZSM-5 zeolite prepared via the fluoride route. Consequently, it was tried to render the synthesis in fluoride medium more environmentally friendly. The different zeolites obtained exhibited interesting MTO-features, such as high stability when prepared with hydrochloric acid, or high ethylene selectivity for those prepared with phosphoric acid.
Finally, the latter catalysts are probably affected by the presence of phosphorous in the zeolite framework, which may alter the acid-base properties of ZSM-5 zeolite.
Acknowledgements
MB and BL thank the Agence nationale de la recherche (ANR) for supporting financially the ANR-10-JCJC-0703 project (SelfAsZeo). PL would like to thank the National Research Fund Luxembourg for his PhD grant. The technical assistance from Thierry Romero and Jean-Daniel Sauer was highly appreciated. UO and BL are grateful to the Aurora programfor funding 31879RB project.
Vous devez vous connecter pour continuer.
S'authentifier