1 Introduction
Water pollution due to organic compounds remains a serious environmental and public problem. The presence of micropollutants in the environment, the so-called emerging contaminants, has raised a great concern among the scientific community during the last few years [1]. Indeed, to date, the removal of organic pollutants to the microgram per liter (μg·L−1) and nanogram per liter (ng·L−1) levels remains a challenge to scientists, local governments, and industry [2].
Therefore, there is a need to develop technologies that can remove these pollutants from wastewaters. Different technologies and processes have been reported and evaluated. Biological treatments [3], membrane processes [4], chemical and electrochemical techniques, advanced oxidation processes (AOPs) [5] and adsorption procedures are the most widely used for removing metals and organic compounds from industrial effluents [6]. Among all the existing treatments, adsorption onto solid materials is one of the more popular methods for the removal of pollutants from wastewater [6].
The characteristics of an effective adsorbent include selectivity, high adsorption capacity, long lifetime for use, and availability in large amounts at low cost [6]. The extending number of studies on the adsorption of organic compounds by modified adsorbents shows that there is a recent increasing interest in the synthesis of new low-cost adsorbents. Much effort has recently been focused on various materials based on polysaccharides like chitin, chitosan or cyclodextrins (CDs) [7,8]. CDs are a family of cyclic oligosaccharides that are composed of α-1,4-linked glucopyranose subunits [9]. The most common CDs are of three types: α-cyclodextrin (α-CD), β-cyclodextrin (β-CD) and γ-cyclodextrin (γ-CD), composed of six, seven and eight glucosyl units, respectively. CDs have a hydrophilic outer surface and a hollow hydrophobic interior (Fig. 1) [10].

(Color online). Chemical structure of α-CD (n = 6), β-CD (n = 7) and γ-CD (n = 8).
This cavity can receive another molecule (guest) provided that it has the correct size and shape (Fig. 2). The potential use of a support containing CD molecules in the reduction of environmental pollution is based on the formation of inclusion complexes between CDs and the unwanted substances [7]. Since CDs are water-soluble they cannot be used directly for water treatment purposes. As a result, bifunctional linking reagents were used to synthesize water-insoluble CDs. Since β-CD is the most accessible, both in cost and reactivity, it was used extensively in this study.

(Color online). Cyclodextrins structure and inclusion complex formation.
The aim of this work was to prepare CD polymers from cross-linking agent and β-CD, and to compare its efficiency vis-à-vis an emerging AOP: photocatalysis. AOPs have emerged as promising water and wastewater treatment technologies for the degradation or mineralization of a wide range of organic contaminants. Among many semiconductors, there is a general consensus among researchers that the commercial TiO2 photocatalyst (Degussa P25) is superior because of its high activity, large stability to light illumination, low price and nontoxicity. However, the lifetime of reactive oxygen species is relatively short under UV irradiation, which often led to a partial mineralization of organic pollutants [11]. Mesoporous TiO2 has been considered to be a promising photocatalyst owing to its advantages such as high specific surface area and multidimensional framework [12–14]. Kim et al. have reported that the photocatalytic activity of mesoporous TiO2 was 5.8 times higher than that of Degussa P25 TiO2 in the degradation of methylene blue [14]. Moreover, the modification of TiO2 with precious metals can alter the charge-transfer properties between TiO2 and the surrounding environment, thus improving the performance of TiO2 nanomaterial-based devices [15–17].
In this study, mesoporous TiO2 prepared by a templating sol–gel method was modified with precious metals such as Ag, Au, and Pd. Adsorption on β-CD polymers and photocatalytic activity of doped and undoped mesoporous TiO2 were compared on real wastewater effluents sampled in the output of two wastewater treatment plants (WWTPs) located in the North of France.
2 Materials and methods
2.1 Reagents
β-cyclodextrin (β-CD) was obtained from Wacker. DMF, toluenediisocyanate (TDI), isophoronediisocyanate (IPDI) hexamethylenediisocyanate (HMDI), carbonyldiimidazole (CDI) (Aldrich), titanium butoxide (Aldrich, 97%), acetic acid (20%) (Fluka), Pluronic P123 (Aldrich, 80%), methanol, ethanol (Aldrich), silver nitrate (Aldrich, 99.8%), palladium nitrate (Aldrich), aqueous solution of tetrachloroauric acid (HAuCl4) (Aldrich) were used as received.
2.2 Wastewater analysis
The sampling campaigns were performed two times in output of the WWTPs. Water sample analyses were carried out on the total fraction by liquid–liquid extraction. Gas chromatography coupled with mass spectrometry (GC–MS) or static-headspace gas chromatography (HS–GC–MS) analyses were realized for the identification of organic pollutants.
Water samples have been collected in amber glass bottles, previously detergent-washed and heated at 450 °C for 8 h. The samples were filtered on glass fiber filters (GFF) (pore size 0.45 mm) immediately after collection, and liquid–liquid extraction with dichloromethane (DCM) was performed during the day of sampling.
GC–MS analyses were performed with a Varian CP3800 Quadrupole MS/MS 1200 device equipped with a VF-5ms column. Temperature conditions were as follows: initial temperature of 70 °C for 2 min, then increased to 310 °C at 5 °C/min.
HS–GC–MS measurements were conducted with a Varian Genesis headspace autosampler coupled with a GC–MS Varian STAR 3400CX Saturn 4D. Sample solutions of 10 mL were introduced into 20-mL headspace vials and sealed using silicone septa and aluminum foil. The vials were then thermostated at 30 ± 0.1 °C. After the equilibrium was established (30 min), 1 mL of vapor from the above solution was withdrawn from the vial using a gas-tight syringe and injected directly in the chromatographic column via a transfer line (200 °C). Temperature conditions were as follows: initial temperature of 40 °C for 4 min, then increased to 220 °C at 5 °C/min.
2.3 Synthesis and characterization of β-cyclodextrin polymers
β-CD polymers were prepared as reported previously [18]. Briefly, 100 mL of anhydrous dimethylformamide (DMF) were placed in a round-bottom flask and 5 g of anhydrous β-CD (4.405 mmol) were added to achieve complete dissolution. Then appropriate amounts (ratio 1:4) of bifunctional cross-linker were added and the solution was allowed to react for 4 h at 70 °C. The polymer formed was then precipitated by the addition of excess methanol, filtered, washed with methanol, dried overnight in an oven at 60 °C and subsequently ground in a mortar. Four β-CD polymers were prepared using toluene diisocyanate (TDI), isophorone diisocyanate (IPDI), hexamethylene diisocyanate (HMDI) and carbonyl diimidazole (CDI) as a cross-linker (Scheme 1).
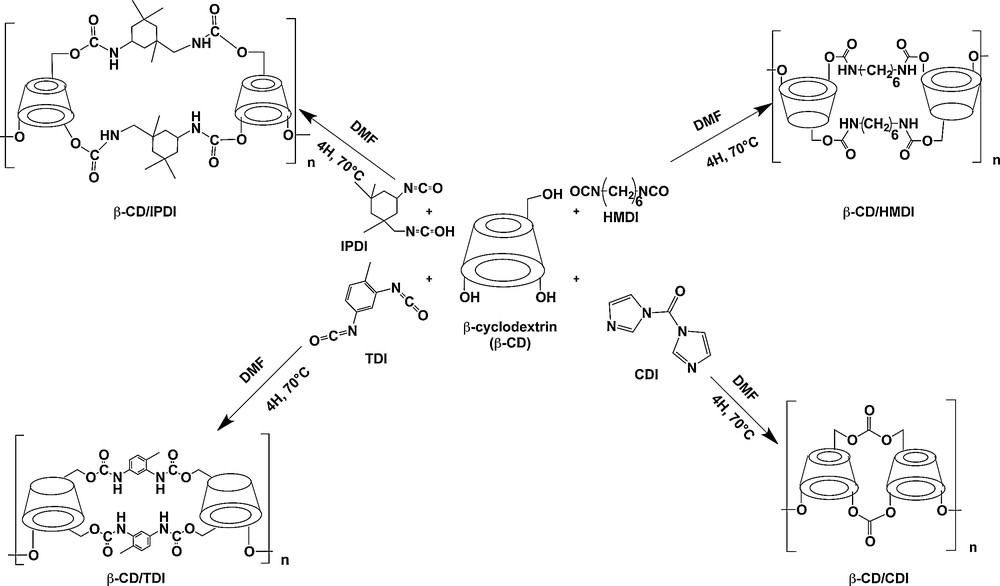
Synthetic pathways for the preparation of CD polymers.
FTIR spectra were obtained in the frequency range between 4000 and 400 cm−1 using a Fourier Transform Infrared spectrometer (FT/IR-6300, JASCO, Japan). FTIR samples were prepared in the form of potassium bromide pellets.
2.4 Retention of VOC by cyclodextrin polymers
The percentage of retention was determined at 30 °C for a weight of 30 mg for CD and CD polymers. For each adsorbent, measurements were done in triplicate. The oven-dried adsorbents were weighed in glass vials and 10 mL of distilled deionized water were added in the vial. After addition of the sorbate (1 ppm) with a mycrosyringe, the vial was sealed using silicone septa and aluminum and analyzed as described in Section 2.2.
The percentage of retention (r) of the studied VOC by the different CD polymers is expressed as follows:
(1) |
2.5 Batch experiments
Samples from the two WWTPs were treated with different amounts of β-CD polymer. After 24 h of agitation, the samples were centrifuged to eliminate the polymers and the solution was analyzed by using a Shimadzu TOC-VCSH analyzer to determine the residual amount of organic pollutants.
2.6 Preparation of undoped and doped TiO2
The synthesis of mesoporous TiO2 was carried out using the following procedure: 5 g of titanium butoxide was added to 30 mL of an aqueous solution of acetic acid (20%) under vigorous stirring. The mixed solution was sealed and kept under stirring for 4 h to obtain solution A. In a separated beaker, 3 g of block copolymer (Pluronic P123) was dissolved in 20 mL of ethanol thoroughly under vigorous stirring to obtain solution B. Then, solution B was slowly added to solution A. The reaction was then carried out at room temperature for 24 h. The solution was then transferred into a Teflon sealed container, which was then heated in an oven at 100 °C for 48 h. After reaction, the precipitate was collected and dried in the air at 80 °C overnight without further washing. The final powder was finally calcined at 500 °C for 4 h to remove organic surfactants and improve the crystallinity of the products [19]. A 3wt% of Ag or Pd was introduced on calcined mesoporous TiO2 via the incipient wetness technique using silver nitrate or palladium nitrate as the sources of Ag and Pd. The calcined TiO2 was thoroughly mixed with an aqueous solution of silver nitrate or palladium nitrate for 1 h and dried in a rotary evaporator at 60 °C. A 3wt% of Au/TiO2 catalyst was prepared by the deposition–precipitation method: an aqueous solution of tetrachloroauric acid (HAuCl4) (3.65·10−4 M) was added under stirring to an aqueous suspension of TiO2 and an aqueous solution of urea (3.65·10−3 M) in excess. The solution is heated at 80 °C to decompose urea and obtain pH = 6–7. The mixture was filtered and washed with deionized water at 60 °C several times in order to eliminate the chloride ions, until there were no more residual Cl− ions, which was testified to using AgNO3, dried during 24 h at 80 °C and finally calcined in the air for 4 h at 400 °C.
2.7 Characterization of undoped and doped TiO2
Powder small- and wide-angle XRD patterns of the calcined samples were recorded on a Bruker AXS D5005 diffractometer equipped with monochromatized Cu Kα radiation (λ = 1.5418 Å) at 40 kV, 30 mA.
The surface area and pore size analysis of the samples were carried out by adsorption–desorption of nitrogen on a Quantachrome NOVA 1000 instrument (–196 °C). Prior to N2 adsorption, the samples were degassed under vacuum at 250 °C for at least 2 h.
The UV–Vis absorption spectra of the materials were recorded on a Cary 100 Scan UV/Vis spectrophotometer (Varian).
2.8 Photocatalytic experiments
Photocatalytic experiments were carried out in a Multirays photoreactor (Heliosquartz) equipped with ten UV lamps of λ = 254 nm (15 W each). Ten milligrams of catalyst were added to the vessel containing 30 mL of wastewater. The catalyst suspension was maintained under stirring in the dark for 30 min until the adsorption/desorption equilibrium was reached. After this, irradiation was started and aliquots of solution were collected at 15-min intervals. The aliquots were immediately filtered to separate TiO2 and analyzed using a Shimadzu TOC-VCSH analyzer.
3 Results and discussion
3.1 Wastewater analysis
The main compounds identified in the wastewater effluents were toluene and methylene chloride for VOC, carbamazepine, caffeine, diethylphthalate, cholesterol, and cresol for non-volatile compounds. These compounds were found in very low amounts (< μg·L−1). Caffeine was found only in the WWTP 1 output, while cholesterol was found only in the WWTP 2 output. The TOC content was 8.71 mg·L−1 and 7.27 mg·L−1 for WWTP 1 and 2, respectively.
3.2 Characterization of the CD polymers
The formation of the polymers was monitored by IR spectroscopy through the disappearance of the isocyanate peak at ν = 2273 cm−1. Similarly, the successful incorporation of the linker was supported by the appearance of a peak corresponding to C = O at ν = 1600 cm−1. Other bands at ν = 2930 cm−1, ν = 1530 cm−1 and ν = 3460 cm−1 corresponding to C–H, NH(CO) and OH stretching were also observed in the polymer [20].
3.3 Characterization of the catalysts
3.3.1 X-ray diffraction
X-ray diffraction (XRD) patterns of Ag/TiO2, Au/TiO2 and Pd/TiO2 were obtained and compared with the mesoporous TiO2 diffraction pattern. Fig. 3 showed the diffraction patterns of undoped and doped TiO2. The peaks at approximately 25°, 38° and 48° are the diffraction peaks of (101), (004) and (200) of anatase, respectively [21–23]. The X-ray diffraction patterns of noble metal/mesoporous TiO2 almost coincide with that of pure TiO2 and showed no diffraction peaks due to the noble metal species, thus suggesting that the metal particles are well dispersed on the TiO2 surface or are present in too low quantity [24].

(Color online). XRD patterns of mesoporous TiO2, Ag/TiO2, Au/TiO2 and Pd/TiO2.
3.3.2 N2 adsorption–desorption isotherms
The N2 adsorption–desorption isotherms (a) and pore size distribution (b) of mesoporous TiO2 calcined at 500 °C are presented in Fig. 4. A typical irreversible type-IV adsorption isotherm with a H1 hysteresis loop, as defined by IUPAC, was observed [25]. This clear H1-type hysteresis loop, situated in the range 0.5 < P/P0 < 0.9, suggested that the material had regular mesoporous channels with narrow Gaussian pore size distribution. The textural properties of mesoporous TiO2 are listed in Table 1.
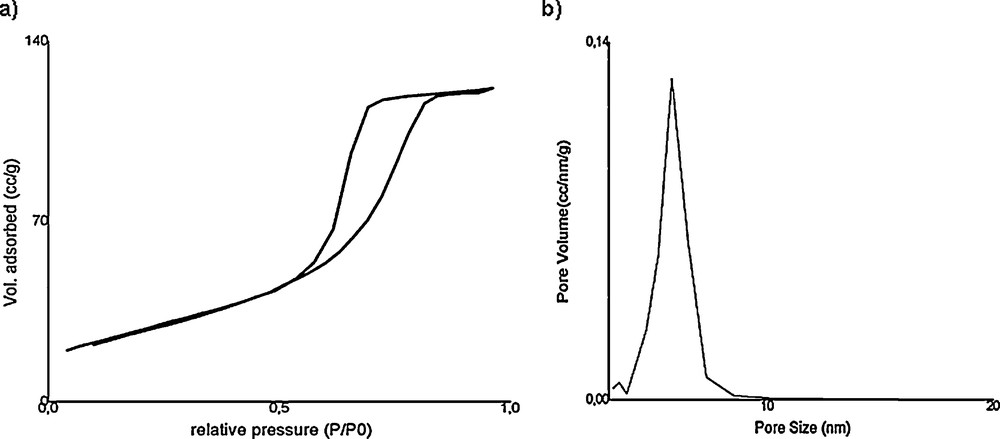
a: nitrogen adsorption and desorption isotherms of mesoporous TiO2; b: pore size distributions of mesoporous TiO2.
Textural properties of mesoporous TiO2.
Catalyst | SBET (m2·g−1) | V (cc·g−1) | d (nm) |
TiO2 | 102 | 0.202 | 5.7 |
3.3.3 Diffuse reflectance ultra-violet visible spectroscopy
The UV–Vis diffuse reflectance measurements were carried out to obtain information about the surface electronic property of TiO2 and noble metal/TiO2. The DR (diffuse reflectance) UV–Vis spectra of TiO2 and noble metal/TiO2 are shown in Fig. 5A. The spectrum of TiO2 consists of a broad intense absorption below ca. 400 nm, usually ascribed to a charge-transfer process from the valence band to the conduction band [26]. The UV–Vis spectra of the noble metal based catalysts showed that Au, Pd and Ag increase the absorption efficiency of TiO2 in visible light, which is favorable to the enhancing of the photocatalytic activity of TiO2 when applying the wastewater treatment. Beside the support's contribution, the UV–Vis spectrum of the gold-based catalyst shows a new band at about 580 nm. This band is due to the surface plasma absorption of the nanosized Au.
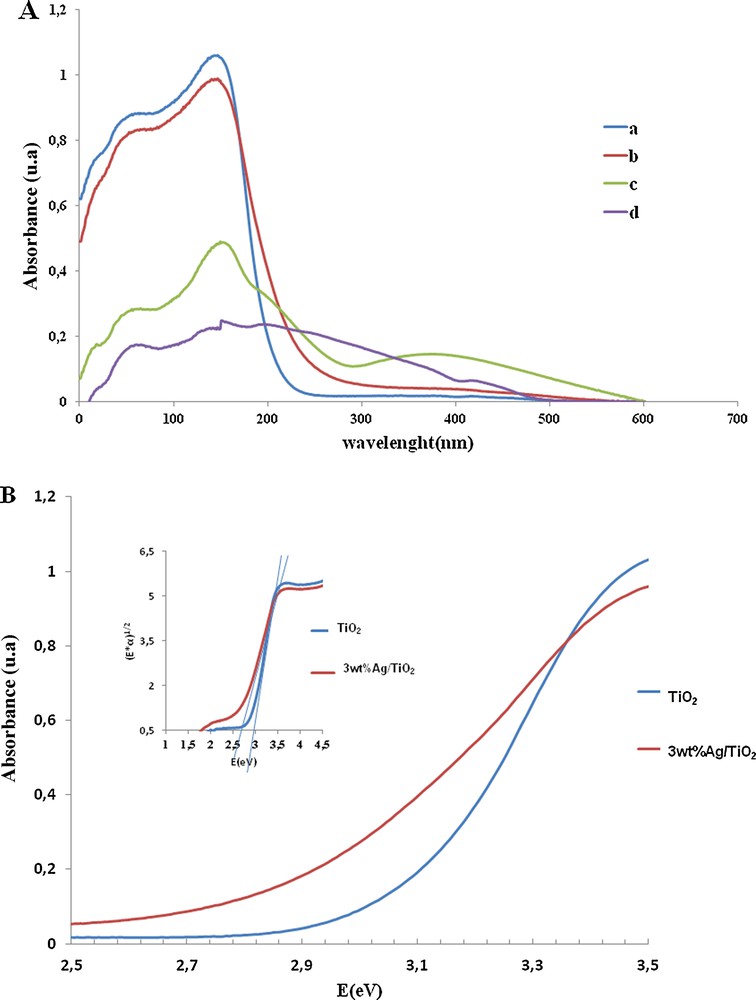
(Color online). A. DR/UV–VIS spectra of the solids: (a) TiO2, (b) 3wt%Ag/TiO2, (c) 3wt%Au/TiO2 and (d) 3%wtPd/TiO2. B. Representative UV–Vis spectra for TiO2 and 3wt%Ag/TiO2. Inset: evolution of (α*E)1/2 as a function of the energy. The extrapolation of the edge down to α = 0 provides the value of the band gap Eg for indirect band gaps [28].
It can be seen from Fig. 5B that the band gap value of Ag/TiO2 is lower than that of undoped TiO2 (2.7 eV and 3.02 eV, respectively). This decrease is due to the fact that the metallic clusters introduce localized energy levels in the TiO2 band gap [27,28].
3.4 Adsorption of organic compounds by β-CD polymers
Adsorption of model VOC by β-CD and the four β-CD polymers was performed in order to choose the best adsorbent for further studies. The results obtained are presented in Fig. 6.

Retention of VOC by β-CD and β-CD polymers.
We can see that β-CD polymers had a better affinity for VOC than native β-CD, and that aromatic derivatives are better recognized by CDs that aliphatic ones. These results are in good agreement with the values of the formation constants of the inclusion complexes (9, 60, 128, 142 and 392 M−1 for dichloromethane, chloroform, benzene, toluene, and ethylbenzene, respectively) [29–31]. The core of the β-CD/TDI macrocyclic structure presents a hydrophobic cavity able to reversibly act as the host to various guest molecules leading to the formation of inclusion complexes. Moreover, pollutants can also be trapped between the polymer network. β-CD/TDI and β-CD/CDI are more effective for toluene trapping than epichlorohydrin polymer [32].
β-CD/TDI polymer gave the best results; therefore, we chose to work with this polymer for batch experiments. After 24 h, we could observe a decrease of the TOC content of the effluent from 8.71 mg·L−1 to 0.68 mg·L−1 for WWTP 1 output and from 7.27 mg·L−1 to 2.53 mg·L−1 for WWTP 2 output (Fig. 7).
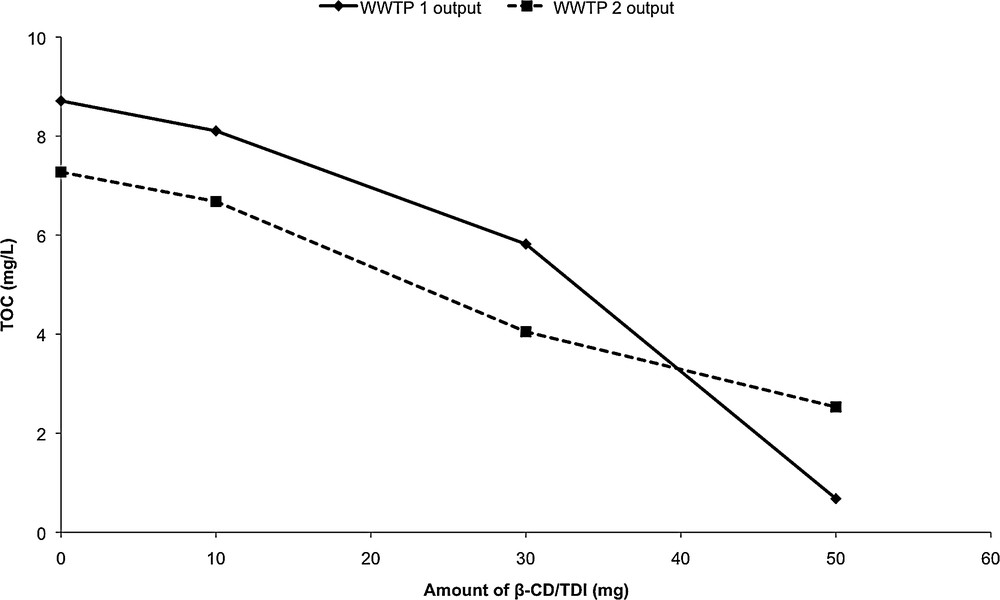
Variation of the TOC content of the wastewater samples after adsorption on various amount of β-CD/TDI polymer.
This decrease in the TOC content (92% and 65% for WWTP 1 output and WWTP 2 output respectively) could be attributed to the adsorption of pollutants by β-CD/TDI polymer. The sorption capacity was found to range between 60.6 mg·g−1 and 160.6 mg·g−1 for WWTP 1 output and between 59.4 mg·g−1 to 94.9 mg·g−1 for WWTP 2 output, depending on the amount of polymers used during batch experiments (Fig. 7). These results showed that sorption onto cross-linked β-CD material is an interesting process to remove organic pollutants before the release of wastewater into the environment. After the adsorption process, the adsorbent material could be regenerated by ethanol washing or eliminated by incineration, thus avoiding the need for fastidious and expensive regeneration [7]. Adsorption capacities were preserved after three adsorption/regeneration cycles (around 90% of the initial capacities, data not shown). These results are in good agreement with those obtained with similar polymers for the removal of parabens [33].
3.5 Photocatalytic treatment
The photocatalytic treatment of wastewater from WWTP 1 output by mesoporous TiO2 and noble metal/mesoporous TiO2 was followed by a record of the TOC content as a function of time (Fig. 8).
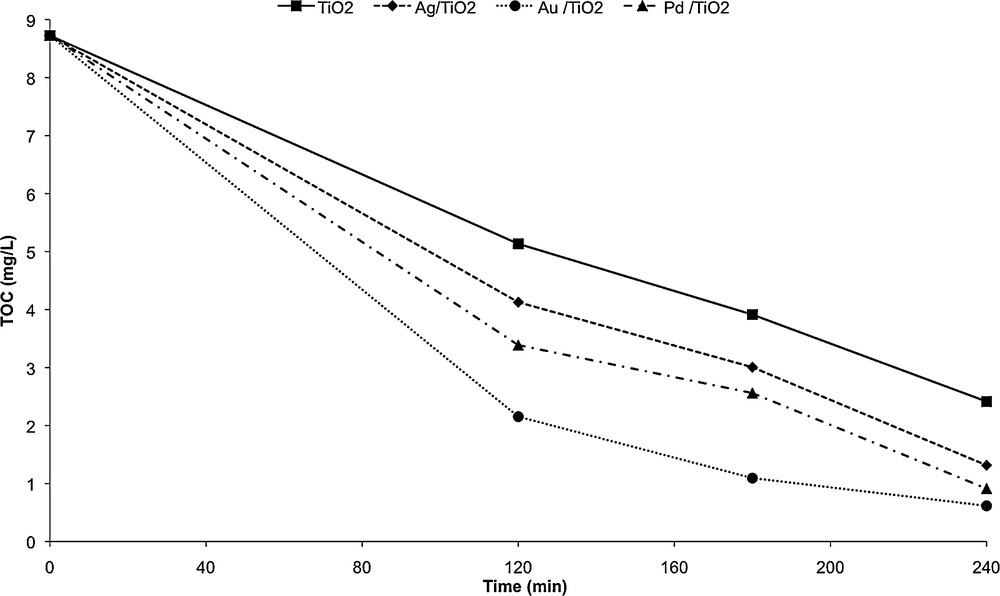
Photocatalytic degradation of wastewater by mesoporous TiO2 and metals doped TiO2 under UV irradiation.
It can be seen from Fig. 8 that the photocatalytic activity of the samples varied in the following order: Au/TiO2 > Pd/TiO2 > Ag/TiO2 > TiO2, confirming that noble metal loading improved the photocatalytic activity of TiO2. The photonic efficiency of Au/TiO2 is higher than that of Pd/TiO2 due to its high electron affinity behavior and suitable work function [34]. Among the noble metal/TiO2 catalysts, Ag/TiO2 exhibited the lowest photocatalytic activity. This could be explained by the size of the Ag particles. Indeed, the UV–Vis spectrum of Ag/TiO2 did not show any band due to the surface plasma absorption of the nanosized Ag, which implies that the Ag particles are quite big. Ismail has reported recently that Ag/TiO2 films with smaller Ag nanoparticles exhibited a higher photocatalytic activity than those with larger Ag particles [35]. This is consistent with our results.
The best TOC abatement obtained after photocatalytic treatment was 93%, and after adsorption on polymer, it was 92%. If these values are similar, a major difference is that photocatalytic treatment led to the mineralization of the organic compounds, while in the case of adsorption the pollutant is not mineralized.
4 Conclusion
This study clearly demonstrated the suitability of the β-CD cross-linked polymer to be used as an adsorbent for the removal of organic pollutants from wastewater. Polymers have good reusability together with a simple regeneration procedure. Doped TiO2 photocatalysts present a better activity than undoped ones. Therefore their photocatalytic activities have to be investigated under visible light irradiation, to lower the cost of the process. These two processes (adsorption and photocatalysis) could be used consecutively. Adsorption could be used to concentrate the pollution and photocatalysis to mineralize pollutant after regeneration of the adsorbent.
Acknowledgments
Authors are grateful to SMCO for the financial support of S. Khaoulani's PhD thesis and to PHC Maghreb No. 27959PD for financial support.