1 Introduction
Since the coumarin system is found in the composition of many natural compounds, derivatives of coumarin have excited considerable attention. Coumarins constitute an important class of compounds, with several types of pharmacological agents possessing anti-cancer, anti-HIV, anticoagulant, spasmolytic and antibacterial activity, among others [1a–c]. Of the many actions of coumarins, antioxidant and antiproliferative effects stand out. A large number of structurally novel coumarin derivatives have ultimately been reported to show substantial cytotoxic activity in vitro and in vivo [1d,1e]. On this basis, medicinal preparations have been obtained and developed with a wide range of biological activities (Psoralen, Angelicin, Xanthotoxin, Bergapten, Nodakenetin, etc.). Imperatorin exhibits an ability for inhibiting malicious tumor growth [2a]. Osthole is effective in inhibiting the migration and invasion of breast cancer cells by wound healing and Transwell® assays and effectively inhibits matrix metalloproteinase promoters and enzyme activity [2b]. Also, unsubstituted coumarin exhibits cytotoxic activity [2c]. On the other hand, selenium has attracted great interest as an essential element and certain diseases have been eradicated by dietary supplementation of this element [3a–c]. Selenium is essential for cell metabolism as a component of glutathione peroxidase and of other enzyme systems. Current interest lies in the prevention of certain cancers by supplementation with selenium [3d–k]. Selenium appears to operate by several mechanisms depending on the chemical form of selenium, the nature of the carcinogenic process, and its dosage. There was no significant difference in the potencies of selenate, selenite, selenium dioxide, selenomethionine and selenocysteine to inhibit the development of mammary tumors, drug-resistant and drug non-resistant human ovarian tumor cells [4a]. We reported previously the synthesis and cytotoxic activity of selenopheno[3,2-c]- and -[2,3-c]quinolones [4b] and 3-substituted benzo[b]selenophenes [4c]. Taking into account the importance of selenium as a trace element in the organism and our previous research [4d–f], the present studies are connected with the elaboration of synthetic methods, cytotoxic activity studies, human matrix metalloproteinases (MMPs), angiogenesis on matrigel in vitro and in vivo inhibition ability, and antioxidant activity in a series of selenopheno[3,2-c]- and [2,3-c]coumarins.
2 Results and discussion
During the last decade, benzo[b]selenophenes have attracted increasing attention in both medicinal chemistry and materials science. From a synthetic point of view, the chemistry of selenium is usually quite complicated [5]. Particularly, the preparation of 2-arylbenzo[b]selenophenes requires multistep protocols, complex reaction conditions and the use of toxic and hazardous chemicals [6]. Recently, we reported a novel approach for the cyclization of arylalkynes with selenium(IV) bromide prepared in situ by dissolving selenium(IV) oxide in concentrated hydrobromic acid using cyclohexene as a bromine molecule scavenger, as well as the utilization of others selenium(II) and (IV) halides in the same reaction [7].
Our synthetic strategies for the insertion of selenophene fragment to coumarins are presented in Scheme 1. We started with the incorporation of an ethynyl substituent in positions 3 or 4 of 3-bromocoumarin (1) and 4-(trifluoromethanesulfonyl)coumarin (2), correspondingly, by a palladium-catalyzed reaction with 2-methyl-2-hydroxybut-3-yne, N-propargylpiperidine, N-propargylmorpholine, and N-propargyl-N′-methylpiperazine under Sonogashira cross-coupling conditions in N-methylpyrrolidone (NMP) or DMF media using bis(triphenylphosphino)palladium(II) chloride or tetrakis(triphenylphosphine)palladium(0) as catalysts in the presence of catalytic amounts of copper(I) iodide and triethylamine as a base. The desired ethynylcoumarins 3–6 and 7–10 were successfully prepared in good to excellent yields.

Synthesis of selenophenocoumarins. Reagents and reaction conditions: a: PdCl2 (10 mol %), Ph3P (20 mol %), CuI (10 mol %), terminal acetylene (1.5 equiv), NMP, Et3N, 55 C, 20 h; b: (Ph3P)4Pd (5 mol %), CuI (20 mol %), terminal acetylene (1.5 equiv), DMF, Et3N, rt, 20 h; c: SeO2 (2 equiv), conc. HBr, dioxane, rt, 24–48 h; d: Pd2dba3 (5 mol %), xantphos (5 mol %), pyridylamine (1.3 equiv), Cs2CO3 (2.7 equiv), xylene, 120 °C, 20 h. 3, 7, 11, 15 (R = CMe2OH), 4, 8, 12, 16 (R = CH2N(CH2)5), 5, 9, 13, 17 (R = CH2N(CH2CH2)2O), 6, 10, 14, 18 (R = CH2N(CH2CH2)2NMe), 19–21 (R = CH2N(CH2CH2)2O), 19 (Ar = 2–Py), 20 (Ar = 3–Py), 21 (Ar = 4–Py). Masquer
Synthesis of selenophenocoumarins. Reagents and reaction conditions: a: PdCl2 (10 mol %), Ph3P (20 mol %), CuI (10 mol %), terminal acetylene (1.5 equiv), NMP, Et3N, 55 C, 20 h; b: (Ph3P)4Pd (5 mol %), CuI (20 mol %), terminal acetylene (1.5 equiv), DMF, Et3N, rt, 20 h; ... Lire la suite
For the formation of the selenophene ring, we used an addition reaction of selenium(IV) bromide to the triple bond. A 1,4-dioxane solution of ethynylcoumarins 3–10 has been treated with in situ prepared selenium(IV) bromide from selenium(IV) oxide and concentrated hydrobromic acid at room temperature. Selenophenocoumarins 11–18 were formed in satisfactory yields (61–72%). It opens a simple and elegant way to the synthesis of a wide range of a various substituted selenophenocoumarin derivatives just in one step.
With the purpose of introducing the amino group in position 3 of the selenophene ring, a Buchwald–Hartwig-type coupling has been examined. After optimization of the reaction conditions, Pd2dba3 as a source of palladium(0) and xantphos as an appropriate ligand were chosen. 3-Bromo-2-morphol-4-yl-methylselenopheno[3,2-c]chromen-4-one (13) has been chosen for the modification as a model compound. According to our results, 2-aminopyridine readily reacts with 13, performing the reaction in xylene at 120 °C using cesium carbonate as a base. The desired 2-morphol-4-ylmethyl-3-(pyrid-2-ylamino)-selenopheno[3,2-c]chromen-4-one (19) was isolated from the reaction mixture in 90% yield. Pyrid-3-ylamino (20) and pyridyl-4-ylamino (21) chromenones were obtained in 70% and 65%, correspondingly.
The structures of the studied compounds 11, 15, 14 and 21 are shown in Fig. 1. The crystal structures of these compounds are different (Table 1). The molecular packing of 11 is characterized by strong intermolecular halogen bonding between bromide and carbonyl oxygen atoms. The length of Br18···O19 is equal 3.267(4) Å, the angle C3–Br18···O19 is 162.3(2)°. In the crystal structure, molecules associate with centrosymmetric dimers by means of halogen bonds. Besides, there is a strong intermolecular hydrogen bond of OH···O-type between the hydroxy group and the pyrane oxygen, with a length of 2.828(5) Å (H15···O5 = 2.19 Å, O15–H15···O5 = 130°). By means of these hydrogen bonds, the chains along the crystallographic axis b form in the crystal structure. The graph set of these chains is C(8), in accordance with the classification of hydrogen bond motifs in crystals [8]. In the crystal structure of 15, there is a strong intermolecular OH···O hydrogen bond between the hydroxy group and the carbonyl oxygen (O15···O19 = 2.871(3)Å, H15···O19 = 2.01 Å, O15–H15···O19 = 151°). By means of these bonds, the chains with graph set C(8) form along the crystallographic axis a. In this crystal structure, unlike in 11, there is no sigma-hole interaction with the bromine atom. In molecule 14, the chair conformation occurs for the piperazine cycle. Also, this conformation is observed for the morpholine cycle in 21. In the crystal structure of 11, there are only weak intermolecular CH···O interactions. A moderate intermolecular NH···O-type hydrogen bond between the amino group and the morpholine oxygen occurs in the crystal structure of 21. Chain formation [with graph set C(9)] along the monoclinic axis b is determined by hydrogen bonds (N21···O18 = 3.108(2)Å, H21···O19 = 2.18 Å, N21–H21···O18 = 165°).
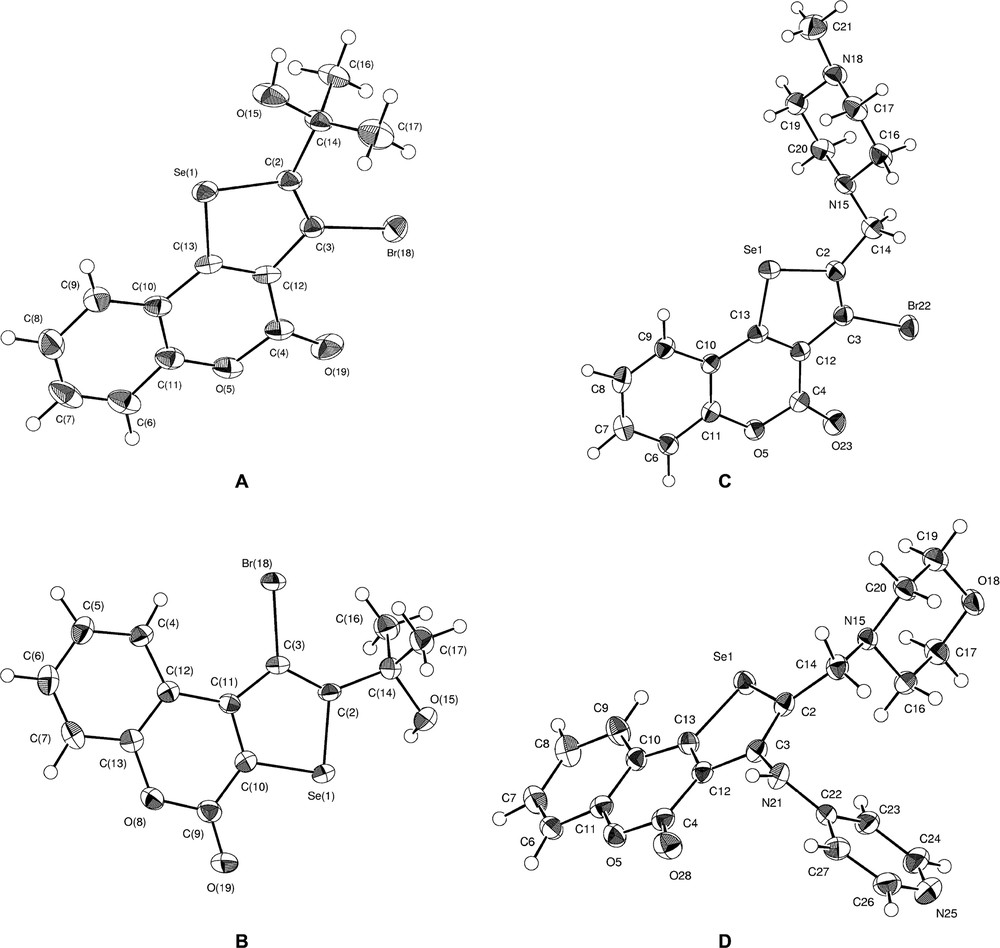
Oak Ridge Thermal-Ellipsoid Plot Program (ORTEP) molecular structures of 11 (A), 15 (B), 14 (C), 21 (D).
Crystal data for the studied compounds.
11 | 15 | 14 | 21 | |
Brutto-formula | C14H11BrO3Se | C14H11BrO3Se | C17H17BrN2O2Se | C21H19N3O3Se |
Formula weight | 386.09 | 386.09 | 440.20 | 440.36 |
Crystal system | Triclinic | Monoclinic | Monoclinic | Monoclinic |
a, [Å] | 6.7735(5) | 12.3871(4) | 9.4797(2) | 9.6710(2) |
b, [Å] | 9.4759(7) | 6.7767(2) | 18.4684(4) | 16.4909(3) |
c, [Å] | 10.5577(9) | 15.9261(5) | 9.6250(3) | 12.1335(3) |
α, [°] | 90.465(3) | 90.0 | 90.0 | 90.0 |
β, [°] | 96.238(3) | 91.430(1) | 94.217(1) | 98.296(1) |
γ, [°] | 94.580(6) | 90.0 | 90.0 | 90.0 |
V, [Å3] | 671.39(9) | 1336.48(7) | 1680.53(7) | 1914.84(7) |
Space group | P 21/n | P 21/c | P 21/c | |
Z | 2 | 4 | 4 | 4 |
μ, [mm−1] | 5.77 | 5.80 | 4.62 | 1.99 |
Density (calc.) [g/cm3] | 1.910 | 1.919 | 1.740 | 1.527 |
2θmax for data [°] | 57.0 | 59.0 | 57.0 | 59.0 |
Reflection collected | 3838 | 6022 | 7507 | 9208 |
Independent reflections | 3395 (Rint = 0.029) | 3590 (Rint = 0.033) | 4202 (Rint = 0.028) | 5228 (Rint = 0.029) |
Reflections with I > 3σ(I) | 2472 | 2661 | 3212 | 3798 |
Number of parameters | 172 | 172 | 208 | 253 |
Final R-factor | 0.036 | 0.035 | 0.050 | 0.034 |
wR2 index for all data | 0.072 | 0.071 | 0.131 | 0.068 |
Temperature, [°C] | –80 | –120 | –100 | –100 |
Using programs | SIR97, maXus | SIR97, maXus | SIR97, maXus | SIR94, maXus |
CCDC deposition number | 913091 | 913093 | 913092 | 913094 |
In vitro antiproliferative activity caused by selenophenocoumarins was tested on monolayer tumor cell lines CCL-8 (mouse sarcoma), MDA-MB-435s (human melanoma), MES-SA (human uterus sarcoma), MCF-7 (human breast adenocarcinoma, estrogen-positive), HT-1080 (human fibrosarcoma) and MG-22A (mouse hepatoma). Using the NIH 3T3 (mouse fibroblasts) cell line the borderline concentration, relevant to the highest tolerated dose, is determined for each compound. The basal cytotoxicity is to be used to predict starting doses for in vivo acute oral LD50 values in rodent [9] (Table 2). Sodium selenite (Na2SeO3) was used as a reference. According to our data 3-bromo-2-(1-hydroxy-1-methylethyl)selenopheno[3,2-c]chromen-4-one (11) and its regioisomer 15 has no in vitro cytotoxic effect on all studied tumor cell lines, besides, these selenophenes are not toxic on NIH 3T3, their acute toxicity being more than 2000 mg/kg (Table 2). More promising results were obtained in a series of aminomethylselenophenocoumarins 12–14 and 16–18. 3-Bromo-2-piperid-1-ylmethylselenopheno[3,2-c]chromen-4-one (12) exhibits the highest antiproliferative activity in vitro on the studied tumor cell lines. The best results were obtained with mouse sarcoma (CCL-8, IC50 = 16 μM) and human uterus sarcoma (MES-SA, IC50 = 26 μM) cell lines. Morpholylmethyl- 13 and N-methylpiperazylmethylcoumarins 14 are less cytotoxic, however, the last one exhibits good activity on MES-SA (IC50 = 9.4 μM), simultaneously with quite high acute toxicity in vitro (LD50 = 334 mg/kg). Compounds have tumor tissue-specific effects. Notably, derivative 14 is more cytotoxic than sodium selenite (IC50 = 29 μM). Also, in accordance with the results of cytotoxic activity selenopheno[3,2-c]coumarins 12–14 are more active against cancer cell growth than the corresponding selenopheno[2,3-c]coumarins 16–18. In a series of 2-morphol-4-ylmethyl-3-(pyridylamino)-selenopheno[3,2-c]chromen-4-ones (19–21), we found that 2-pyridylamino derivative 19 has no cytotoxic activity on studied cell lines, however, 3- and 4-pyridylaminocoumarins 20 and 21 exhibit a medium antiproliferative effect on tumor cell growth. Notably, selenophenocoumarin 21 was able to inhibit human breast adenocarcinoma cells (MCF-7, IC50 = 18 μM). Furthermore, selenophenocoumarins 12–21 (LD50 = 334–2000 mg/kg) are much less toxic than Na2SeO3 (LD50 = 105 mg/kg).
In vitro cytotoxicity in monolayer tumor cell lines CCL-8 (mouse sarcoma), MDA-MB-435s (human melanoma) MES-SA (human uterus sarcoma), MCF-7 (human breast adenocarcinoma, estrogen-positive), HT-1080 (human fibrosarcoma), MG-22A (mouse hepatoma) and normal cell line NIH 3T3 (mouse fibroblasts) caused by selenophenocoumarins.
Number | CCL-8 | MDA-MB-435s | MES-SA | MCF-7 | HT-1080 | MG-22A | 3T3 | LD50 mg/kg |
IC50 | IC50 | IC50 | IC50 | IC50 | IC50 | IC50 | ||
Na2SeO3 | 8 | 12 | 29 | 17 | 1.7 | 64 | 23 | 105 |
11 | 128 | a | a | a | a | 246 | a | > 2000 |
12 | 16 | 45 | 26 | 39 | 47 | 27 | 156 | 660 |
13 | 104 | 47 | a | 111 | a | a | a | > 2000 |
14 | 29 | 40 | 9.4 | 44 | 41 | 29 | 40 | 334 |
15 | 96 | a | 259 | 184 | 93 | 79 | a | > 2000 |
16 | 64 | a | a | a | a | 50 | 2147 | > 2000 |
17 | 70 | 156 | a | 99 | a | 217 | 653 | 1476 |
18 | 38 | 44 | 32 | 35 | 43 | 37 | 123 | 572 |
19 | a | a | 195 | 134 | a | 166 | a | > 2000 |
20 | 36 | 47 | 34 | 41 | 77 | 42 | 97 | 705 |
21 | 41 | 36 | 44 | 18 | 41 | 27 | 46 | 484 |
a No cytotoxic effect.
Matrix metalloproteinases (MMPs), also designated as matrixins, hydrolyze components of the extracellular matrix. These proteinases play a central role in many biological processes, such as embryogenesis, normal tissue remodeling, wound healing, and angiogenesis, and in diseases such as atheroma, arthritis, cancer, and tissue ulceration. Matrix metalloproteinase MMP-2 (gelatinase A) is very active at degrading extracellular matrix [10]. Inhibition of matrix metalloproteinase enzymes by synthesized selenolocoumarins 12–21 were detected on the MMP Inhibitor Fluorimetric Profiling kit using NNGH (N-isobutyl-N-(4-methoxyphenylsulfonyl)glycylhydroxamic acid) as a standard. According to our data, selenolocoumarins 12–18 exhibit no ability to inhibit MMPs (Table 3).
MMP and angiogenesis in vitro and in vivo inhibition caused by selenophenocoumarins 12–21.
Number | MMP 1 | MMP 2 | MMP 3 | MMP 7 | MMP 8 | MMP 9 | MMP 10 | MMP 12 | MMP 13 | MMP 14 | Angiogenesis, % | |
in vitro | in vivo | |||||||||||
NNGHa | 100 | 100 | 100 | 18.0 | 100 | 100 | 100 | 100 | 100 | 100 | ||
12 | 8.5 | 9.0 | 9.4 | 3.1 | 0.0 | 10.6 | 11.3 | 11.6 | 8.1 | 19.3 | ||
13 | 4.5 | 0.0 | 7.5 | 2.0 | 0.0 | 7.8 | 6.5 | 8.7 | 23.2 | 7.3 | ||
14 | 10.1 | 14.7 | 10.0 | 6.4 | 0.0 | 11.2 | 14.0 | 13.3 | 12.2 | 33.5 | 84 ± 14 | 30 ± 4 |
15 | 3.9 | 0.5 | 12.5 | 4.2 | 0.0 | 14.0 | 9.2 | 6.0 | 12.7 | 8.7 | ||
16 | 0.9 | 10.8 | 4.5 | 7.3 | 0.0 | 1.5 | 1.5 | 0.0 | 6.1 | 11.9 | ||
17 | 0.0 | 0.0 | 0.0 | 4.2 | 0.0 | 0.0 | 0.0 | 0.0 | 6.6 | 8.5 | ||
18 | 0.0 | 0.0 | 0.0 | 6.5 | 0.0 | 2.4 | 0.0 | 0.0 | 7.0 | 13.7 | ||
19 | 13.6 | 31.9 | 13.4 | 10.7 | 16.9 | 12.7 | 12.8 | 12.2 | 10.6 | 21.6 | 3 ± 1 | 36 ± 8 |
20 | 18.5 | 26.4 | 14.1 | 14.2 | 15.4 | 15.3 | 16.3 | 13.8 | 23.7 | 24.6 | ||
21 | 12.0 | 54.0 | 15.8 | 12.3 | 12.4 | 17.0 | 12.6 | 14.9 | 14.9 | 40.0 | 95 ± 7 | 9 ± 4 |
a N-isobutyl-N-(4-methoxyphenylsulfonyl)-glycylhydroxamic acid.
However, the introduction of aminopyridyl moiety bromo substituent in 3-bromo-2-morphol-4-yl-methyl-selenolo[3,2-c]chromen-4-one (13) molecule leads to a selective increase of MMP-2 and MMP-14 inhibition. 2–Pyridylamino 19 and 3-pyridylamino derivative 20 are able to inhibit MMP-2 up to 26%. The best results, obtained by introducing a 4-pyridylamino group, selenolocoumarin 21 shows selective 54% inhibition of MMP-2 compared to NNGH. In accordance with MMPs inhibition data, three compounds (14, 19 and 21) were chosen for angiogenesis inhibition studies in vitro and in vivo. An in vitro experiment has been performed on Matrigel (BD Biosciences) human umbilical vein endothelial cell line (HUVECs) tube formation model. According to the obtained experimental results, 3-bromo-2-(4-methylpiperaz-1-yl)-methyl-selenolo[3,2-c]chromen-4-one (14) is able to inhibit angiogenesis by 84%, also 2- and 4-pyridylaminocoumarins 19 and 21 slow down angiogenesis by 3% and 95%, correspondingly. The angiogenesis model in vivo was based on the use of Matrigel implants in Balb/c AnNCrl mice (20 g, 4 weeks of age). It has been shown that N-methylpiperazylcoumarin 14 retard angiogenesis by 30%. However, more interesting results were obtained for pyridylaminocoumarins 19 and 21. 2–Pyridyl derivative 19 shows 36% angiogenesis inhibition level, but 4-pyridylcoumarin 21 almost completely lost its ability in in vivo tests. In our opinion, it could be explainable by coumarin cycle hydrolysis during experiments on a living model. After hydrolysis, compound 19 may form tridentate chelate (pyridyl nitrogen, amino group, and carbonic acid moiety), but with 4-pyridyl derivative 21 only the two-dentate form is obtained. Further research will be connected with profound SAR studies with the purpose to find more active and selective substances.
Nowadays, there is an increased interest in using antioxidants for medical purposes. According to literature data, selenium-containing compounds possess modulating activity of oxidative stress during carcinogenesis [11]. So, we tested selected compounds (Table 4) on free radical, super oxide, nitric oxide, peroxyl and hydroxyl radical scavenging activities. Similar to coumarin and 7-hydroxycoumarin, selenophenocoumarins 11–20 were not able to scavenge free radicals, nitric oxide, and hydroxyl radicals. However, 3-pyridylamino coumarin 20 exhibits low activity on super oxide concentration inhibition (IC50 = 160 μM). Moreover, N-methylpiperazylaminomethyl derivatives 14 and 18 are medium peroxyl radical scavengers (IC50 = 62 and 87 μM, correspondingly). It should be noted that coumarin 20 is only two times less active than 7-hydroxycoumarin in peroxyl radical scavenging (IC50 = 4 μM). Selenium-containing compounds showed high levels of superoxide generation in vitro via the oxidation of reduced glutathione [12]. An inspection of superoxide generation ability data showed activity caused by (1-hydroxy-1-methylethyl)selenophenocoumarins 11 and 15 (627 and 950 RLU, correspondingly). Besides, pyridylamino derivative 20 exhibits an 1875 RLU SOD generation level.
Radical scavenging activity (IC50, μM) caused by selenophenocoumarins.
Compound | Super oxide, IC50 | Peroxyl radical, IC50 | Super oxide generation, RLU |
Coumarin | – | > 1000 | – |
7-hydroxycoumarin | – | 2 | – |
Resveratrol | 17 | 1 | – |
11 | – | – | 627 |
15 | – | – | 950 |
12 | – | 320 | – |
16 | 710 | 598 | – |
13 | – | 1000 | – |
17 | – | 398 | – |
14 | 260 | 62 | – |
18 | – | 87 | – |
20 | 160 | 4 | 1875 |
3 Conclusions
In summary, we have elaborated a simple method for the synthesis of substituted selenopheno[2,3-c] and -[3,2-c]coumarins in the reaction of substituted ethynylcoumarins with selenium(IV) bromide. The antiproliferative activity of novel selenophenocoumarins showed higher activity and lower acute toxicity than Na2SeO3 on various tumor cell lines. Promising result received on mouse sarcoma (CCL-8, up to IC50 = 16 μM) and human uterus sarcoma (MES-SA, up to IC50 = 9.4 μM) cell lines. 3-(4–Pyridylamino)-selenopheno[3,2-c]chromen-4-one 21 possesses the ability to inhibit human breast adenocarcinoma cells (MCF-7, IC50 = 18 μM). Furthermore, selenophenocoumarins (LD50 = 334–2000 mg/kg) are much less toxic than sodium selenite (LD50 = 105 mg/kg).
Selenophenocoumarin 21 shows a selective inhibition ability of metalloproteinase MMP-2 (54%). Methylpiperazyl substituted selenophenocoumarin 14 is able to inhibit angiogenesis by 84%, also 2- and 4-pyridylaminocoumarins 19 and 21 slow down angiogenesis by 3% and 95%, correspondingly. N-Methylpiperazylaminomethyl derivatives 14 and 18 are medium peroxyl radical scavengers (IC50 = 62 and 87 μM, correspondingly). It should be noted that coumarin 20 is only two times less active than 7-hydroxycoumarin in peroxyl radical scavenging (IC50 = 4 μM). Besides, pyridylamino derivative 20 exhibits prooxidant activity (SOD = 1875 RLU).
4 Experimental
4.1 General
1H, 13C and 77Se NMR spectra were recorded on a Varian 400 Mercury spectrometer at 400.0, 100.3 and 39.74 MHz correspondingly at 303 K in CDCl3/TMS or DMSO-d6 solution. The 1H chemical shifts are given relative to TMS, 13C – relative to chloroform or DMSO, and 77Se – relative to dimethyl selenide. The melting points were determined on a “Digital melting point analyser” (Fisher), the results are given without correction. Diffraction data were collected on a Nonius KappaCCD diffractometer using graphite monochromated Mo Kα radiation (λ = 0.71073 Å). The crystal structures were solved by direct methods and refined by full-matrix least squares. The main crystallographic data and refinement parameters of the crystal structures are listed in Table 1. For further details, see the crystallographic data for these compounds deposited with the Cambridge Crystallographic Data Centre as Supplementary Publications. Copies of the data can be obtained, free of charge, on application to CCDC, 12 Union Road, Cambridge CB2 1EZ, UK.
4.1.1 General procedure for the preparation of 3-alkynylchromen-2-ones 3–6
Argon gas was bubbled for 15 min at 40 C in a vial charged with palladium(II) chloride (39 mg, 0.22 mmol), triphenylphosphine (115 mg, 0.44 mmol) and copper(I) iodide (42 mg, 0.22 mmol) in 4 mL of dry NMP. Then a solution of 1 (0.5 g, 2.2 mmol) and terminal acetylene (3.3 mmol) in dry NMP (5 mL), and triethylamine (1 mL) was added to the reaction mixture. After 20 h of heating at 55 °C and usual workup, the crude product was purified by flash chromatography on silica gel using the mixture methylene chloride/methanol as an eluent.
4.1.1.1 3-(3-Hydroxy-3-methyl-but-1-ynyl)chromen-2-one (3): Yield: 46% [13]
4.1.1.1.1 3-(3-Piperid-1-yl-prop-1-ynyl)chromen-2-one (4)
Yield: 42%, mp = 95–97 °C. 1H NMR (400 MHz, CDCl3/TMS) δ (ppm): 1.44–1.49 (2H, m, CH2CH2CH2), 1.60–1.63 (4H, m, NCH2CH2), 2.55–2.58 (4H, m, NCH2), 3.53 (2H, s, CH2N), 7.25–7.32 (2H, m, 6-CH, 8-CH), 7.44 (1H, dd, J = 1.4 Hz, J = 7.6 Hz, 5-CH), 7.50 (1H, ddd, J = 1.4 Hz, J = 7.2 Hz, J = 8.4 Hz, 7-CH), 7.84 (1H, s, 4-CH). 13C NMR (100.6 MHz, CDCl3/TMS) δ (ppm): 23.8 (NCH2CH2), 25.9 (NCH2CH2CH2), 48.5 (NCH2), 53.4 (NCH2CH2), 78.9 (coumarin-C≡C), 92.4 (coumarin-C≡C), 112.9, 116.7, 118.7, 124.7, 127.5, 131.9, 144.8 (CO), 153.2 (4-CH), 159.5 (CO); elemental analysis calcd (%) for C17H17NO2: C 76.38, H 6.41, N 5.24; found: C 76.11, H 6.33, N 5.07.
4.1.1.1.2 3-(3-morphol-4-yl-prop-1-ynyl)chromen-2-one (5)
Yield: 41%, oil. 1H NMR (400 MHz, CDCl3/TMS) δ (ppm): 2.64 (4H, t, J = 4.6, CH2N), 3.56 (2H, s, CH2N), 3.76 (4H, t, J = 4.6, OCH2), 7.27–7.31 (2H, m, 6-CH, 8-CH), 7.45 (1H, dd, J = 1.4 Hz, J = 7.6 Hz, 5-CH), 7.52 (1H, ddd, J = 1.4 Hz, J = 7.2 Hz, J = 8.4 Hz, 7-CH), 7.85 (1H, s, 4-CH). 13C NMR (100.6 MHz, CDCl3/TMS) δ (ppm): 48.1 (NCH2CH2O), 52.3 (NCH2CH2O), 66.8 (NCH2), 79.4 (coumarin-C≡C), 91.2 (coumarin-C≡C), 112.6 (3-C), 116.7, 118.6, 124.7, 127.6, 132.1, 145.1 (CO), 153.2 (4-CH), 159.4 (CO); elemental analysis calcd (%) for C16H15NO3: C 71.36, H 5.61, N 5.20; found: C 71.24, H 5.50, N 5.13.
4.1.1.1.3 3-(3-(4-Methylpiperaz-1-yl)prop-1-ynyl)chromen-2-one (6)
Yield: 40%, oil. 1H NMR (400 MHz, CDCl3/TMS) δ (ppm): 2.30 (3H, s, CH3), 2.40–2.58 (4H, m, CH2NCH3), 2.65–2.74 (4H, m, NCH2), 3.58 (2H, s, CH2N), 7.25–7.32 (2H, m, 6-CH, 8-CH), 7.44 (1H, dd, J = 1.4 Hz, J = 7.6 Hz, 5-CH), 7.51 (1H, ddd, J = 1.4 Hz, J = 7.2 Hz, J = 8.4 Hz, 7-CH), 7.85 (1H, s, 4-CH). 13C NMR (100.6 MHz, CDCl3/TMS) δ (ppm): 45.9 (CH3N), 47.7 (NCH2), 51.9 (NCH2CH2NMe), 54.9 (NCH2CH2NMe), 79.3 (coumarin-C≡C), 91.6 (coumarin-C≡C), 112.7 (3-C), 116.7, 118.7, 124.7, 127.6, 132.1, 145.1 (CO), 153.2 (4-CH), 159.4 (CO); elemental analysis calcd (%) for C17H18N2O2: C 72.32, H 6.43, N 9.92; found: C 72.16, H 6.47, N 9.59.
4.1.2 General procedure for the preparation of 4-alkynylchromen-2-ones 7–10
Argon gas was bubbled in a flask charged with 2 (0.5 g, 1.7 mmol), acetylene (2.5 mmol), tetrakis(triphenylphosphine)palladium(0) (98 mg, 0.085 mmol) and copper(I) iodide (64 mg, 0.34 mmol) in 5 mL of dry DMF, then triethylamine (0.7 mL, 5.1 mmol) was added to the reaction mixture. After 20 h of stirring at room temperature and usual workup, the crude product was purified by flash chromatography on silica gel using the mixture methylene chloride/methanol as an eluent.
4.1.2.1 4-(3-Hydroxy-3-methylbut-1-ynyl)chromen-2-one (7) [14] Yield: 98%
4.1.2.1.1 4-(3-Piperid-1-yl-prop-1-ynyl)chromen-2-one (8)
Yield: 75%, oil. 1H NMR (400 MHz, CDCl3/TMS) δ (ppm): 1.44–1.50 (2H, m, CH2CH2CH2), 1.63–1.69 (4H, m, NCH2CH2), 2.58–2.62 (4H, NCH2), 3.64 (2H, s, CH2N), 6.54 (1H, s, 3-CH), 7.29–7.34 (2H, m, 6-CH, 8-CH), 7.54 (1H, ddd, J = 1.4 Hz, J = 7.2 Hz, J = 8.4 Hz, 5-CH), 7.85 (1H, dd, J = 1.4 Hz, J = 8.2 Hz, 7-CH). 13C NMR (100.6 MHz, CDCl3/TMS) δ (ppm): 23.7 (NCH2CH2CH2), 25.9 (CH2CH2CH2), 48.6 (NCH2), 53.5 (NCH2CH2CH2), 78.9 (coumarin-C≡C), 99.2 (coumarin-C≡C), 116.9, 118.4, 118.9, 124.4, 126.7, 132.2, 137.3 (4-C), 153.5 (CO), 160.1 (CO); elemental analysis calcd (%) for C17H17NO2: C 76.38, H 6.41, N 5.24; found: C 76.27, H 6.44, N 5.19.
4.1.2.1.2 4-(3-Morphol-4-yl-prop-1-ynyl)chromen-2-one (9)
Yield: 76%, oil. 1H NMR (400 MHz, CDCl3/TMS) δ (ppm): 2.66 (4H, t, J = 4.6, CH2N), 3.67 (2H, s, CH2N), 3.79 (4H, t, J = 4.6, OCH2), 6.54 (1H, s, 3-CH), 7.29–7.33 (2H, m, 6-CH, 8-CH), 7.55 (1H, ddd, J = 1.4 Hz, J = 7.2 Hz, J = 8.4 Hz, 5-CH), 7.83 (1H, dd, J = 1.4 Hz, J = 8.2 Hz, 7-CH). 13C NMR (100.6 MHz, CDCl3/TMS) δ (ppm): 48.1 (NCH2), 52.4 (NCH2CH2O), 66.8 (NCH2CH2O), 79.3 (coumarin-C≡C), 97.9 (coumarin-C≡C), 117.0, 118.3, 119.1, 124.4, 126.5, 132.3, 136.9 (4-C), 153.5 (CO), 160.0 (CO); elemental analysis calcd (%) for C16H15NO3: C 71.36, H 5.61, N 5.20; found: C 71.33, H 5.67, N 5.11.
4.1.2.1.3 4-(3-(4-Methylpiperaz-1-yl)prop-1-ynyl)chromen-2-one (10)
Yield: 67%, oil. 1H NMR (400 MHz, CDCl3/TMS) δ (ppm): 2.29 (3H, s, CH3), 2.42–2.57 (4H, m, CH2NCH3), 2.65–2.76 (4H, m, NCH2), 3.66 (2H, s, CH2N), 6.51 (1H, s, 3-CH), 7.25–7.30 (2H, m, 6-CH, 8-CH), 7.52 (1H, ddd, J = 1.4 Hz, J = 7.2 Hz, J = 8.4 Hz, 5-CH), 7.81 (1H, dd, J = 1.4 Hz, J = 8.2 Hz, 7-CH). 13C NMR (100.6 MHz, CDCl3/TMS) δ (ppm): 45.8 (NCH3), 47.5 (NCH2), 51.9 (NCH2CH2NMe), 54.7 (NCH2CH2NMe), 78.9 (coumarin-C≡C), 98.3 (coumarin-C≡C), 116.6, 118.1, 118.7, 124.2, 126.5, 132.0, 136.9 (4-C), 153.2 (CO), 159.8 (CO); elemental analysis calcd (%) for C17H18N2O2: C 72.32, H 6.43, N 9.92; found: C 72.24, H 6.40, N 9.69.
4.1.3 General procedure for the preparation of selenopheno[3,2-c], [2,3-c]-coumarins 11–18
3-Alkynyl-chromen-2-one 3–10 (1.0 mmol) in dioxane was added to a solution of selenium dioxide (0.22 g, 2.0 mmol) in HBr (2 mL), and the mixture was stirred at room temperature for 24–48 h. After the consumption of substrate 3–10 (LC-MS), the reaction mixture was basified by aqueous Na2CO3 and extracted with methylene chloride. The organic phase was washed with brine, dried over anhydrous Na2SO4, filtered, concentrated, and the residue was purified by flash chromatography on silica gel using the mixture methylene chloride/ethylacetate as an eluent.
4.1.3.1 3-Bromo-2-(1-hydroxy-1-methylethyl)selenopheno[3,2-c]chromen-4-one (11)
Yield: 71%, mp = 224–225 °C. 1H NMR (400 MHz, CDCl3/TMS) δ (ppm): 1.84 (6H, s, CH3), 2.86 (1H, br s, OH), 7.26 (1H, ddd, J = 1.4 Hz, J = 7.2 Hz, J = 8.4 Hz, 6-CH), 7.35 (1H, dd, J = 1.4 Hz, J = 8.4 Hz, 8-CH), 7.47 (1H, ddd, J = 1.4 Hz, J = 7.2 Hz, J = 8.4 Hz, 7-CH), 7.57 (1H, dd, J = 1.4 Hz, J = 7.8 Hz, 9-CH). 13C NMR (100.6 MHz, CDCl3/TMS) δ (ppm): 28.7 (C(CH3)OH), 74.8 (C(CH3)OH), 102.7, 117.1, 118.6, 124.2, 124.6, 125.4, 130.8, 150.1, 152.5, 155.8, 156.2 (CO). 77Se NMR (39.74 MHz, CDCl3/TMS) δ (ppm): 605.9. ESI-MS m/z 387 [M + 1]; elemental analysis calcd (%) for C14H11BrO3Se: C 43.55, H 2.87; found: C 43.46, H 2.88.
4.1.3.2 3-Bromo-2-piperid-1-ylmethylselenopheno[3,2-c]chromen-4-one (12)
Yield: 72%, mp = 224–226 °C. 1H NMR (400 MHz, CDCl3/TMS) δ (ppm): 1.46–1.52 (2H, m, CH2CH2CH2), 1.60–1.66 (4H, m, NCH2CH2), 2.58–2.65 (4H, m, NCH2), 3.72 (2H, s, CH2N), 7.25 (1H, ddd, J = 1.4 Hz, J = 7.2 Hz, J = 8.4 Hz, 6-CH), 7.33 (1H, dd, J = 1.4 Hz, J = 8.4 Hz, 8-CH), 7.45 (1H, ddd, J = 1.4 Hz, J = 7.2 Hz, J = 8.4 Hz, 7-CH), 7.55 (1H, dd, J = 1.4 Hz, J = 7.8 Hz, 9-CH). 13C NMR (100.6 MHz, CDCl3/TMS) δ (ppm): 23.9 (NCH2CH2CH2), 26.0 (NCH2CH2CH2), 55.1 (NCH2), 59.1 (NCH2CH2CH2), 117.1, 118.9, 124.2, 124.6, 130.7, 150.1, 155.6, 158.9 (CO). ESI-MS m/z 426 [M + 1]; elemental analysis calcd (%) for C17H16BrNO2Se: C 48.02, H 3.79, N 3.29; found: C 48.00, H 3.95, N 3.01.
4.1.3.3 3-Bromo-2-morphol-4-yl-methylselenopheno[3,2-c]chromen-4-one (13)
Yield: 75%, mp = 215–216 °C. 1H NMR (400 MHz, CDCl3/TMS) δ (ppm): 2.68 (4H, t, J = 4.6, CH2N), 3.75 (4H, t, J = 4.6 Hz, OCH2), 3.78 (2H, s, CH2N), 7.26 (1H, ddd, J = 1.4 Hz, J = 7.2 Hz, J = 8.4 Hz, 6-CH), 7.35 (1H, dd, J = 1.4 Hz, J = 8.4 Hz, 8-CH), 7.47 (1H, ddd, J = 1.4 Hz, J = 7.2 Hz, J = 8.4 Hz, 7-CH), 7.54 (1H, dd, J = 1.4 Hz, J = 7.8 Hz, 9-CH). 13C NMR (100.6 MHz, CDCl3/TMS) δ (ppm): 53.9 (NCH2), 58.8 (NCH2CH2O), 67.0 (NCH2CH2O), 106.7, 117.1, 118.8, 124.2, 124.6, 130.9, 146.9, 150.2, 154.1, 155.5 (CO). 77Se NMR (39.74 MHz, CDCl3/TMS) δ (ppm): 616.4. ESI-MS m/z 428 [M + 1]; elemental analysis calcd (%) for C16H14BrNO3Se: C 44.99, H 3.30, N 3.28; found: C 44.91, H 3.22, N 3.17.
4.1.3.4 3-Bromo-2-(4-methylpiperaz-1-yl)-methylselenopheno[3,2-c]chromen-4-one (14)
Yield: 68%, mp > 230 °C. 1H NMR (400 MHz, CDCl3/TMS) δ (ppm): 2.31 (3H, s, CH3), 2.42–2.56 (4H, m, CH2NCH3), 2.64–2.78 (4H, m, NCH2), 3.77 (2H, s, CH2N), 7.25 (1H, ddd, J = 1.4 Hz, J = 7.2 Hz, J = 8.4 Hz, 6-CH), 7.34 (1H, dd, J = 1.4 Hz, J = 8.4 Hz, 8-CH), 7.46 (1H, ddd, J = 1.4 Hz, J = 7.2 Hz, J = 8.4 Hz, 7-CH), 7.54 (1H, dd, J = 1.4 Hz, J = 7.8 Hz, 9-CH). 13C NMR (100.6 MHz, CDCl3/TMS) δ (ppm): 46.0 (CH3N), 53.6 (NCH2), 55.2 (NCH2CH2NMe), 58.4 (NCH2CH2NMe), 106.2, 117.1, 118.9, 124.2, 124.6, 130.8, 148.1, 150.2, 154.0, 155.5 (CO). 77Se NMR (39.74 MHz, CDCl3/TMS) δ (ppm): 618.2. ESI-MS m/z 441 [M + 1]; elemental analysis calcd (%) for C17H17BrN2O2Se: C 46.38, H 3.89, N 6.36; found: C 46.30, H 3.71, N 6.00.
4.1.3.5 1-Bromo-2-(1-hydroxy-1-methylethyl)selenopheno[2,3-c]chromen-4-one (15)
Yield: 70%, mp > 200 °C. 1H NMR (400 MHz, CDCl3/TMS) δ (ppm): 1.87 (6H, s, CH3), 2.94 (1H, br s, OH), 7.33 (1H, ddd, J = 1.4 Hz, J = 7.2 Hz, J = 8.4 Hz, 6-CH), 7.42 (1H, dd, J = 1.4 Hz, J = 8.2 Hz, 8-CH), 7.52 (1H, ddd, J = 1.4 Hz, J = 7.2 Hz, J = 8.4 Hz, 7-CH), 9.23 (1H, dd, J = 1.4 Hz, J = 8.2 Hz, 9-CH). 13C NMR (100.6 MHz, CDCl3/TMS) δ (ppm): 28.3 (C(CH3)OH), 75.3 (C(CH3)OH), 100.8, 117.9, 118.7, 122.9, 123.8, 126.7, 130.1, 143.9, 153.0, 157.9 (CO), 169.9 (CO). 77Se NMR (39.74 MHz, CDCl3/TMS) δ (ppm): 624.4. ESI-MS m/z 387 [M + 1]; elemental analysis calcd (%) for C14H11BrO3Se: C 43.55, H 2.87; found: C 43.51, H 2.82.
4.1.3.6 1-Bromo-2-piperid-1-yl-methylselenopheno[2,3-c]chromen-4-one (16)
Yield: 64%, mp = 193–195 °C. 1H NMR (400 MHz, CDCl3/TMS) δ (ppm): 1.46–1.52 (2H, m, CH2CH2CH2), 1.62–1.67 (4H, m, NCH2CH2), 2.62–2.67 (4H, m, NCH2), 3.69 (2H, s, CH2N), 7.33 (1H, ddd, J = 1.4 Hz, J = 7.2 Hz, J = 8.4 Hz, 6-CH), 7.43 (1H, dd, J = 1.4 Hz, J = 8.2 Hz, 8-CH), 7.52 (1H, ddd, J = 1.4 Hz, J = 7.2 Hz, J = 8.4 Hz, 7-CH), 9.12 (1H, dd, J = 1.4 Hz, J = 8.2 Hz, 9-CH). 13C NMR (100.6 MHz, CDCl3/TMS) δ (ppm): 23.8 (NCH2CH2CH2), 26.2 (NCH2CH2CH2), 55.4 (NCH2), 60.4 (NCH2CH2CH2), 117.8, 118.5, 123.4, 123.8, 130.0, 143.8, 153.1 (CO), 158.0 (CO). 77Se NMR (39.74 MHz, CDCl3/TMS) δ (ppm): 638.2. ESI-MS m/z 426 [M-1]; elemental analysis calcd (%) for C17H16BrNO2Se: C 48.02, H 3.79, N 3.29; found: C 47.88, H 3.61, N 3.11.
4.1.3.7 1-Bromo-2-morphol-4-yl-methylselenopheno[2,3-c]chromen-4-one (17)
Yield: 65%, mp > 200 °C. 1H NMR (400 MHz, CDCl3/TMS) δ (ppm): 2.73 (4H, t, J = 4.0, CH2N), 3.76–3.79 (6H, m, CH2N, OCH2), 7.34 (1H, ddd, J = 1.4 Hz, J = 7.2 Hz, J = 8.4 Hz, 6-CH), 7.43 (1H, dd, J = 1.4 Hz, J = 8.2 Hz, 8-CH), 7.52 (1H, ddd, J = 1.4 Hz, J = 7.2 Hz, J = 8.4 Hz, 7-CH), 9.12 (1H, dd, J = 1.4 Hz, J = 8.2 Hz, 9-CH). 13C NMR (100.6 MHz, CDCl3/TMS) δ (ppm): 54.2 (NCH2), 60.1 (NCH2CH2O), 67.0 (NCH2CH2O), 103.8, 117.8, 123.3, 123.9, 130.2, 143.5, 153.0, 157.8 (CO), 160.9 (CO). 77Se NMR (39.74 MHz, CDCl3/TMS) δ (ppm): 616.4. ESI-MS m/z 428 [M + 1]; elemental analysis calcd (%) for C16H14BrNO3Se: C 44.99, H 3.30, N 3.28; found: C 44.79, H 3.14, N 3.22.
4.1.3.8 1-Bromo-2-(4-methylpiperaz-1-yl)-methylselenopheno[2,3-c]chromen-4-one (18)
Yield: 67%, mp > 200 °C. 1H NMR (400 MHz, CDCl3/TMS) δ (ppm): 2.34 (3H, s, CH3), 2.46–2.57 (4H, m, CH2NCH3), 2.72–2.80 (4H, m, NCH2), 3.77 (2H, s, CH2N), 7.34 (1H, ddd, J = 1.4 Hz, J = 7.2 Hz, J = 8.4 Hz, 6-CH), 7.44 (1H, dd, J = 1.4 Hz, J = 8.2 Hz, 8-CH), 7.53 (1H, ddd, J = 1.4 Hz, J = 7.2 Hz, J = 8.4 Hz, 7-CH), 9.14 (1H, dd, J = 1.4 Hz, J = 8.2 Hz, 9-CH). 13C NMR (100.6 MHz, CDCl3/TMS) δ (ppm): 45.9 (CH3N), 53.8 (NCH2), 55.2 (NCH2CH2NMe), 59.6 (NCH2CH2NMe), 103.4, 117.8, 118.4, 123.4, 123.9, 127.6, 130.1, 143.5, 153.1, 157.9 (CO), 162.0 (CO). 77Se NMR (39.74 MHz, CDCl3/TMS) δ (ppm): 635.6. ESI-MS m/z 441 [M + 1]; elemental analysis calcd (%) for C17H17BrN2O2Se: C 46.38, H 3.89, N 6.36; found: C 46.27, H 3.81, N 6.20.
4.1.4 General procedure for the preparation of 2-morpholin-4-ylmethyl-3-(pyridinylamino)-selenopheno[3,2-c]chromen-4-ones 19–21
Argon gas was bubbled for 15 min in a vial charged with 13 (0.2 g, 0.47 mmol), Pd2dba3 (21 mg, 0.023 mmol), xantphos (27 mg, 0.046 mmol) and pyridylamine (57 mg, 0.61 mmol) in 5 mL of xylene. Then freshly dried cesium carbonate (0.412 g, 1.26 mmol) was added and the reaction mixture was heated for 20 h at 120 °C. After usual workup, the crude product was purified by flash chromatography on silica gel using the mixture methylene chloride/methanol as an eluent.
4.1.4.1 2-Morphol-4-ylmethyl-3-(pyrid-2-ylamino)selenopheno[3,2-c]chromen-4-one (19)
Yield: 90%, mp = 195–197 °C. 1H NMR (400 MHz, CDCl3/TMS) δ (ppm): 2.61 (4H, t, J = 4.6 Hz, CH2O), 3.64 (2H, s, CH2N), 3.73 (4H, t, J = 4.6, NCH2), 6.68 (1H, d, J = 8.4 Hz, 3-CH–Py), 6.74 (1H, ddd, J = 0.8 Hz, J = 1.2 Hz, J = 7.2 Hz, 5-CH–Py), 7.29 (td, J = 1.2 Hz, J = 7.8 Hz, 6-CH), 7.36 (1H, dd, J = 0.8 Hz, J = 8.2 Hz, 8-CH), 7.46 (1H, ddd, J = 1.6 Hz, J = 7.4 Hz, J = 8.4 Hz, 4-CH–Py), 7.51 (1H, ddd, J = 1.6 Hz, J = 7.2 Hz, J = 8.4 Hz, 7-CH), 7.62 (1H, dd, J = 1.2 Hz, J = 7.8 Hz, 9-CH), 8.04 (1H, br s, NH), 8.17 (1H, dd, J = 1.2 Hz, J = 4.8 Hz, 6-CH–Py) 13C NMR (100.6 MHz, CDCl3/TMS) δ (ppm): 54.1 (NCH2), 58.7 (NCH2CH2O), 67.1 (NCH2CH2O), 110.3, 115.4, 117.1, 119.6, 121.4, 124.0, 124.8, 130.2, 133.9, 137.5, 138.2, 147.8, 149.6, 152.4, 155.8, 158.5 (CO). 77Se NMR (39.74 MHz, CDCl3/TMS) δ (ppm): 547.8. ESI-MS m/z 442 [M + 2]; elemental analysis calcd (%) for C21H19N3O3Se: C 57.28, H 4.35, N 9.54; found: C 57.21, H 4.33, N 9.50.
4.1.4.2 2-Morphol-4-ylmethyl-3-(pyrid-3-ylamino)selenopheno[3,2-c]chromen-4-one (20)
Yield: 70%, mp = 153–155 °C. 1H NMR (400 MHz, CDCl3/TMS) δ (ppm): 2.55 (4H, t, J = 4.6 Hz, CH2O), 3.38 (2H, s, CH2N), 3.71 (4H, t, J = 4.6 Hz, CH2N), 7.09 (1H, ddd, J = 1.6 Hz, J = 2.7 Hz, J = 8.2 Hz, 4-CH–Py), 7.17 (1H, dd, J = 8.2 Hz, J = 4.8 Hz, 5-CH–Py), 7.30 (1H, td, J = 1.2 Hz, J = 7.8 Hz, 6-CH), 7.37 (1H, dd, J = 0.8 Hz, J = 8.2 Hz, 8-CH), 7.47 (1H, br s, NH), 7.49 (1H, ddd, J = 1.6 Hz, J = 7.4 Hz, J = 8.4 Hz, 7-CH), 7.62 (1H, dd, J = 1.2 Hz, J = 7.8 Hz, 9-CH), 8.16 (1H, dd, J = 1.2 Hz, J = 4.8 Hz, 6-CH–Py), 8.21 (1H, d, J = 2.7 Hz, 2-CH–Py) 13C NMR (100.6 MHz, CDCl3/TMS) δ (ppm): 53.9 (NCH2), 57.6 (NCH2CH2O), 67.0 (NCH2CH2O), 117.2, 119.4, 121.2, 123.4, 123.6, 124.0, 124.9, 130.6, 134.2, 136.1, 139.5, 140.5, 141.9, 149.7, 153.3, 158.5 (CO). 77Se NMR (39.74 MHz, CDCl3/TMS) δ (ppm): 542.4. ESI-MS: m/z 442 [M + 2]; elemental analysis calcd (%) for C21H19N3O3Se: C 57.28, H 4.35, N 9.54; found: C 57.28, H 4.37, N 9.43.
4.1.4.3 2-Morphol-4-ylmethyl-3-(pyrid-4-ylamino)selenopheno[3,2-c]chromen-4-one (21)
Yield: 65%, mp = 202–205 °C. 1H NMR (400 MHz, CDCl3/TMS) δ (ppm): 2.59 (4H, t, J = 4.6 Hz, CH2O), 3.46 (2H, s, CH2N), 3.72 (4H, t, J = 4.6 Hz, NCH2), 6.60 (2H, d, J = 5.9 Hz, 3-CH–Py, 5-CH–Py), 7.31 (1H, td, J = 1.2 Hz, J = 7.8 Hz, 6-CH), 7.37 (1H, dd, J = 0.8 Hz, J = 8.2 Hz, 8-CH), 7.44 (1H, br s, NH), 7.50 (1H, ddd, J = 1.6 Hz, J = 7.4 Hz, J = 8.4 Hz, 7-CH), 7.62 (1H, dd, J = 1.2 Hz, J = 7.8 Hz, 9-CH), 8.33 (2H, d, J = 5.9 Hz, 2-CH–Py, 6-CH–Py). 13C NMR (100.6 MHz, CDCl3/TMS) δ (ppm): 53.9 (NCH2), 57.6 (NCH2CH2O), 67.0 (NCH2CH2O), 110.2, 117.3, 119.3, 121.4, 124.1, 124.9, 130.8, 134.2, 138.4, 149.8, 150.3, 150.9, 153.4, 158.0 (CO). 77Se NMR (39.74 MHz, CDCl3/TMS) δ (ppm): 548.5. ESI-MS m/z 442 [M + 2]; elemental analysis calcd (%) for C21H19N3O3Se: C 57.28, H 4.35, N 9.54; found: C 57.10, H 4.39, N 9.37.
4.2 In vitro cytotoxicity assay
Monolayer tumor cell line: CCL-8 (mouse sarcoma), MDA-MB-435s (human melanoma) MES-SA (human uterus sarcoma), MCF-7 (human breast adenocarcinoma, estrogen-positive), HT-1080 (human fibrosarcoma), MG-22A (mouse hepatoma) and normal cell line NIH 3T3 (mouse fibroblasts) were cultured in standard medium Dulbecco's modified Eagle's medium (DMEM) without an indicator (“Sigma”) supplemented with 10% heat-inactivated fetal bovine serum (“Sigma”). After the ampoule was thawed, the cells from 1 to 4 passages were used. About 2–5 × 104 cells/mL (depending on line nature) were placed in 96-well plates immediately after compounds had been added to the wells. The control cells without test compounds were cultured on separate plates. The plates were incubated for 72 h, 37 °C, 5% CO2. The number of surviving cells was determined using tri(4-dimethylaminophenyl)methyl chloride (Crystal Violet) or 3-(4,5-dimethylthiazol-2-yl)-2,5-diphenyltetrazolinium bromide (MTT). MTT-test: after incubating with preparations, the culture medium was removed and 200 μL of fresh medium with 10 mM HEPES were added in each well of the plate, than 20 μL MTT (2 mg/mL in HBSS) were added. After incubation (3 h, 37 °C, 5% CO2), the medium with MTT was removed and 200 μL of DMSO and 25 μL of glycine buffer (pH 10.5) were added at once to each sample. The samples were tested at 540 nm on Anthos HT II photometer. CV-test: after incubating with preparations cell culture was removed and 100 mL of 1% glutaraldehide in HBSS was added to each well. After incubation (15 min) the HBSS with glutaraldehide washed off H2O (1 time) and 0.05% crystal violet were added. After incubation with dye (15 min), the samples were washed off with H2O (3 times), and citrate buffers (pH 4.2) and ethanol (1:1) were added. The samples were tested at 540 nm.
4.3 MMP assay
Inhibitors of matrix metalloproteinase enzymes were detected with the use of MMP Inhibitor Fluorimetric Profiling kit (Biomol, USA) accordingly to the manufacturer's instructions. MMP activity assays were performed in 96-well plates using the recombinant human MMP-1–10, 12, 13 and 14 catalytic domains and OmniMMP™ fluorogenic substrate Mca-Pro-Leu-Gly-Leu-Dpa-Ala-Arg-NH2. The test compounds (20 μM) were dissolved in DMSO. The compound NNGH (N-isobutyl-N-(4-methoxyphenylsulfonyl)-glycylhydroxamic acid) was used as a prototypic control inhibitor. The rate of substrate hydrolysis was determined by fluorescence intensity measurements for 10 min at 37 °C using a fluorescence plate reader (Tecan infinite M1000, Austria) with excitation at 328 nm and emission at 420 nm. Data analysis was performed using program GraphPad Prism® 4.0.
4.4 In vitro angiogenesis assay on matrigel
Human umbilical vein endothelial cells (HUVEC) were purchased from BD™ Biosciences and cultured in Vascular Cell Basal Medium (ATCC PCS-100-030) containing Endothelial Cell Growth kit (ATCC PCS-100-040) with growth factors and 2% fetal bovine serum. Cells were grown at 37 °C in a humidified atmosphere of 5% CO2–95% air. HUVECs used for the experiments were between passage 2 and 4.
The in vitro Angiogenesis Assay Kit (Item. No. 10009964, Cayman Chemical Company) was used in addition to the HUVEC proliferation assay. The BD Matrigel (BD Matrigel ™ Basement Membrane Matrix, High Concentration) was thawed at 4 °C and mixed to homogeneity using cooled pipette tips. Cell culture plates (96-well) were bottom-coated with a thin layer of BD Matrigel (40 μL/well), which was left to polymerize at 37 °C for 60 min. HUVEC (1 × 104 cells) were stimulated with 0.25 μM PMA (phorbol 12-myristate 13-acetate) in 80 μL of medium and added to each well on the solidified BD Matrigel. The culture medium was added to each well in the presence or in the absence of compounds. The plates were incubated at 37 °C for 18–20 h and the endothelial tubes were quantified using a fluorescent microscope after staining with Calcein AM. Three microscope fields were selected at random and photographed. Tube formation was analyzed with an imaging system (Image-Pro 4.1). Each experiment was performed at least three times.
The murine Matrigel plug assay can be used to evaluate the anti-angiogenic effect. Female Balb/c AnNCrl mice (20 g, 4 weeks of age) were obtained from Charles River Laboratories. Before injection, heparin was incubated with bFGF, VEGF and with or without tested compounds for 5 min, then diluted into phenol red-free Matrigel™ (BD Basement Membrane Matrix High Concentration, Catalog No. 354248) on ice for a final concentration of 60 units/mL heparin, 10 μg/mL bFGF, 100 ng/mL VEGF. Then, mice were subcutaneously injected under anesthesia (setona/ketamine) with 0.5 mL of Matrigel™ alone or with Matrigel™ containing bFGF, VEGF and tested compounds. PBS was used as a negative control, and VEGF as a positive control. Ten days after injection, mice were euthanized, and the Matrigel plugs were removed, embedded in OCT (Tissue-Tek), and frozen. Forty-micron sections (n = 5 sections per plug) were stained with hematoxylin/eosin and analyzed by confocal microscopy (Nicon). The Image-Pro Plus analysis system was used to quantify the percentage of area occupied by the vessel-like structures in each field.
4.5 Free radical scavenging activity [15]
The ability to scavenging the stable free radical, DPPH, was measured as a decrease in absorbance at 517 nm by the method of Brand–Williams et al. with some modifications. Several concentrations of test compounds in DMSO were prepared. The compound solution (0.1 mL) was added to the ethanol DPPH solution (0.1 mL, 0.2 mM) and the mixture was kept in the dark for 120 min. The absorbance at 517 nm was then measured by Tecan infinite M1000 (Tecan) fluorometer. The percentage of scavenging activity was calculated using the following formula:
The IC50 values for each compound as well as for the standard preparation were calculated by computer program GraphPad Prism 3.0.
4.6 Superoxide free radical scavenging activity [16]
Ten microliters of a riboflavin solution [0.1 mg/mL], 10 μL of an EDTA solution [12 mM], 10 μL of methanol and 5 μL of a NBT (nitro-blue tetrazolium) solution [1 mg/mL] were mixed in a test tube and the reaction mixture was diluted up to 150 μL with a phosphate buffer [50 mM]. The absorbance of the solution was measured at 590 nm using a phosphate buffer as a blank after illumination for 5 min. This was taken as a control. Several solutions with different concentrations of test compounds in DMSO or water were prepared. To each of these, 10 μL of riboflavin, 10 μL of EDTA, 10 μL of methanol, 5 μL of NBT and 15 μL of the test compounds were mixed in a 96-well plate and further diluted up to 150 μL with a phosphate buffer.
Absorbance was measured after illumination for 5 min at 590 nm on fluorometer Tecan infinite M1000 (Tecan). The percent of scavenging activity was calculated using the following formula:
The IC50 values for each compound as well as for the standard preparation were calculated by computer program GraphPad Prism 3.0.
4.7 Nitric oxide radical scavenging
At physiological pH, nitric oxide generated from an aqueous sodium nitroprusside (SNP) solution interacts with oxygen to produce nitrite ions, which may be quantified by the Griess reaction. The reaction mixture contained 10 mM SNP, phosphate buffered saline (pH 7.4) and various doses (4–1000 μM) of the test solution in a final volume of 0.09 mL. After incubation for 30 min at 25 °C, 0.150 mL of Griess reagent (1% sulfanilamide, 2% H3PO4, 0.1% napthylethylenediamine dihydrochloride) was added and the mixture was incubated for 20 min at 25 °C. The absorbance of the chromophore formed was measured at 546 nm on Tecan infinite M1000 (Tecan) spectrometer. Curcumin was used as the positive control. The percentage of scavenging activity was calculated using the following formula:
The IC50 values for each compound as well as for the standard preparation were calculated by computer program GraphPad Prism 3.0.
4.8 Peroxyl radical scavenging [17]
Analyses were conducted in a phosphate buffer (pH 7.4 at 37 °C). Peroxyl radicals were generated using 2,2′-azo-bis(2-amidinopropane)dihydrochloride, which was freshly prepared for each run. Fluorescein was used as the substrate. Fluorescence conditions were as follows: excitation at 485 nm and emission at 520 nm. Sodium fluorescein was dissolved in phosphate buffer solution (PBS) (75 mM, pH = 7.0) to obtain a stock solution of 4.8 mM. The working solution (60 nM) was obtained by subsequent dilution in PBS. A solution of AAPH (2,2′-azo-bis(2-amidinopropane)dihydrochloride) was prepared at a concentration of 40 mM. All measurements were performed in triplicate. A blank was run with each assay. The analysis was performed using black 96-well microplates (NuncTM) and a Tecan infinite M1000 (Tecan) multilabel counter. The sample (20 μL) was mixed with sodium fluorescein (120 μL) and incubated for 15 min at 37 °C. An AAPH (60 μL) solution was then added to the mixture and the microplate was shaken. The fluorescence (λexcitation = 485/14 nm; λemission = 535/25 nm) was registered every 2 min for 1 h. The quantitation of the antioxidant capacity was based on the calculation of the area under the curve (AUC).
4.9 Superoxide anion generating activity [11]
The control chemiluminescent (CL) assay cocktail without substrates or GSH was made using a 0.05 M sodium phosphate buffer (pH 7.4) containing 0.025 μg lucigenin/mL. The assay cocktail with thiol contained 7 mg GSH/mL. To aliquots of thiol containing the assay cocktail was added each selenium compound in various doses (4–1000 μM) of the test solution in DMSO. Chemiluminescent data were recorded at 25 °C in 30-s integrated units over a period of up to 20 min with Tecan infinite M1000 (Tecan). There was a 3-s instrumental delay between integrations.
Acknowledgments
The financial support for this work provided by Latvian Council of Science (447/2012 and 593/2014), and National Research Program 2010–2013 (BIOMEDICINE) is gratefully acknowledged.