1 Introduction
Studies on the isolation, characterization and catalytic activities of functionalized carbon nanotubes (CNTs) have received particular attention during the last decade owing to their specific catalytic applications compared to homogeneous complexes. Metal nanoparticles as well as various transition metal complexes such as polymers and porphyrins were used for the functionalization of carbon nanotubes [1–5]. Schiff bases, which are an important class of ligands with extensive applications in different fields [6], also showed excellent catalytic activity when grafted on CNTs [7–10]. Wildgoose et al. reviewed the recent developments in this area by exploring the various techniques allowing one to functionalize the carbon nanotubes with metals and other nanoparticles, and the diverse applications of the resulting materials [11]. However, the applications of CNTs have been impeded by their poor solubility in solvents and polymers, which originated from strong van der Waals attractions among CNTs. Without surface modification, most of CNTs lack sufficient binding sites for anchoring precursor metal ions or metal nanoparticles, which usually lead to poor dispersion and aggregation of metal nanoparticles, especially at high loading conditions. Therefore, the functionalization of CNTs is generally a prerequisite to further applications. According to the nature of the interaction between CNTs and functional groups, the functionalization of CNTs can be categorized into covalent and non-covalent functionalization types.
The palladium-catalyzed carbon–carbon and carbon–heteroatom bond-forming reactions play a key role in the synthesis of many important chemicals, including pharmaceuticals, herbicides, polymers, and so on [12–16]. Recently, the immobilization of Pd nanoparticles on solid supports has received considerable attention as a new generation of heterogeneous catalysts in various scientific fields and Suzuki reaction, because of their superior catalytic performance, good stability, ease of separation and satisfactory reusability in comparison to the traditional homogeneous Pd (OAc)2, PdCl2 catalysts [17–27]. However, the catalytic activity of Pd2+-CNTs decreased gradually when the catalyst was used repeatedly. This could be ascribed to the weak interaction between palladium and the support material, as well as to the agglomeration and accumulation of Pd nanoparticles on the surface of the material [28,29]. Therefore, it is desirable to improve the stability, recyclability, and catalytic activity of heterogeneous Pd nanocatalysts. Owing to these interests and also as part of our ongoing research program on the application of catalysts for the development of useful new synthetic methodologies [30], we report herein the synthesis of a heterogeneous palladium nanocatalyst supported on diethylenetriamine-grafted-SWCNTs (SWCNT-DETA/Pd+2), and the catalytic activity of the prepared catalyst was investigated by employing the Suzuki–Miyaura coupling reaction as a model reaction. The result showed that whereas the new catalyst retains the reactivity characteristic of a homogeneous catalyst, at the same time it is easy to separate off and to reuse.
2 Experimental
2.1 Materials
All the reagents were purchased from Aldrich and Merck and used without any purification. SOCl2, HCl, H2SO4, HNO3, H2O2 (30 wt%, aq), deionized water, NaH (80%), anhydrous dimethylformamide (DMF), CaH2, and metformin hydrochloride were obtained from Sigma Aldrich and Merck.
2.2 Covalent grafting of diethylenetriamine (DETA) to SWCNTs
Pristine SWCNTs (p-SWCNTs) were refluxed under stirring in a mixture of 1:3 (v/v) HNO3 and H2SO4 at 70 °C for 30 h, which was followed by centrifugation and repeated washings with DI water [31]. The dried carboxylated SWCNTs (SCNTs-COOH) were suspended in a solution of thionyl chloride (excess) and DMF (1 mL). The suspension was stirred at 65 °C for 24 h. The solid was then separated by filtration and washed with anhydrous THF (30 mL), and dried in vacuo to obtain MWCNTs-COCl. The final product was then subjected to functionalization with diethylenetriamine. Diethylenetriamine (the amine-to-SWCNT weight ratio was 10:1) was mixed with 10 mL of a solution of DMF and TEA (1 mL), and then stirred for 5 min. The obtained acyl chloride SWCNTs that were dispersed in DMF (20 mL) using an ultrasonic bath for 15 min were subsequently added to the suspension. The reaction mixture was kept at 120 °C for 2 days. The solid was then separated by filtration and washed with CH2Cl2 and deionized water for several times and dried in vacuo.
2.3 Preparation of SWCNT-DETA/Pd2+and SWCNT-DETA/Pd0 nanocatalysts
The SWCNT-DETA (1 g) were dispersed in 30 mL DI water by sonication for 10 min, and an aqueous solution of PdCl2 (10 mL, 0.6 mmol) was added to a dispersion of SWCNT-DETA. The mixture was stirred for 24 h at room temperature to complete the attainment of the coordination. Thus the obtained SWCNT-DETA/Pd2+ nanocatalyst was subjected to centrifugation, washed with DI water and dried in vacuo at 40 °C for 12 h. The overall synthesis of SWCNT-DETA/Pd2+ nanocatalyst is schematically displayed in Scheme 1.
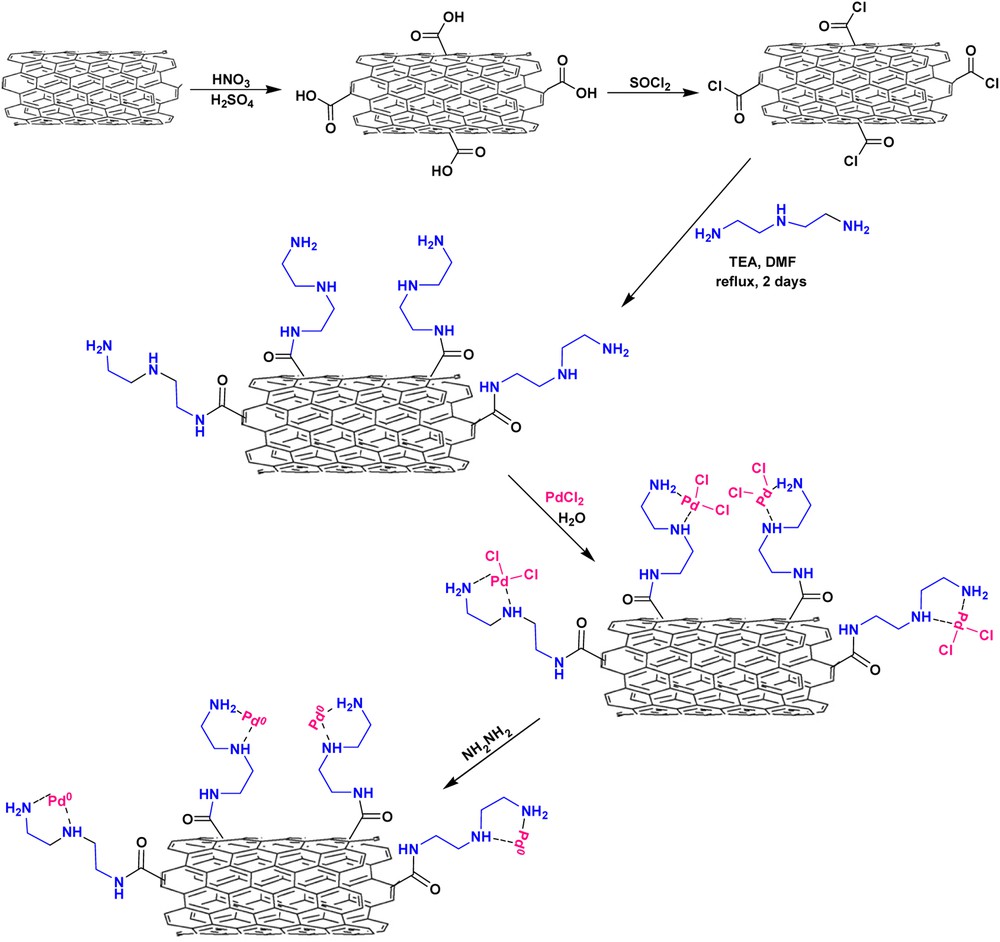
(Color online.) Schematic diagram of the fabrication of SWCNT-DETA/Pd2+ and SWCNT-DETA/Pd0.
The reduction of SWCNT-DETA/Pd2+ by hydrazine hydrate was performed as follows: 30 mg of SWCNT-DETA/Pd2+ were dispersed in 60 mL of water, and then 100 μL of hydrazine hydrate (80%) were added. The pH of the mixture was adjusted to 10 with 25% ammonium hydroxide, and the reaction was carried out at 95 °C for 2 h. The final product SWCNT-DETA/Pd0 was washed with water and dried in vacuo at 50 °C. Scheme 1 depicts the synthesis procedure of SWCNT-DET/Pd2+ and SWCNT-DETA/Pd0. The concentration of palladium in SWCNT-DETA/Pd2+ was 22 wt%, which was determined by ICP-AES and TGA.
2.4 Catalytic activity – Suzuki–Miyaura coupling reaction
In a typical reaction, 10 mg of the catalyst (1 mol%) were placed in a 25-mL Schlenk tube; 1.1 mmol of aryl halide in 5 mL of water/ethanol (1:1) was added 0.134 g (1.1 mmol) of phenyl boronic acid, 0.276 mg of K2CO3 (2 mmol). The mixture was then stirred for the desired time at 60 °C. The reaction was monitored by thin layer chromatography (TLC). After completion of the reaction, the reaction mixture was cooled to room temperature and the catalyst was recovered by centrifugation and washed with ethyl acetate and ethanol. The combined organic layer was dried over anhydrous sodium sulfate and evaporated in a rotary evaporator under reduced pressure.
3 Results and discussion
The diethylenetriamine-functionalized Single-Walled Carbon Nanotubes anchored palladium (II) complex (SWCNT-DETA/Pd2+) was conveniently synthesized from commercially available and cheap materials via immobilization on SWCNTs. The pathways of SWCNT-DETA/Pd2+ fabrication are shown in Scheme 1.
The surface structure of the materials was confirmed using Fourier transform infrared (FTIR) spectroscopy. Fig. 1 shows the FTIR spectra obtained for (a) SWCNT, (b) SWCNT-COOH, (c) SWCNT-COCl, (d) SWCNT-CO-DETA, (e) SWCNT-CO-DETA/Pd (II). As can be seen in curve b, the band at 1728 cm−1 corresponds to the carbonyl stretch of the carboxylic acid group. The conversion of the carboxylic acid groups (SWCNT-COOH) into the acyl chloride intermediate (SWCNT-COCl) by treatment with thionyl chloride was confirmed by the appearance of a peak near 1778 cm−1 stretching in curve c. Curve d shows the spectrum of SWCNT-DETA, and the absorption band at 1651 cm−1 was attributed to the carbonyl stretching of the amide groups (–CONH–). Also, the bands observed around 2950 cm−1 were assigned to the bending vibration of CH2. The prominent IR bands at 3300 and 1579 cm−1 were ascribed to the NH2 stretching vibrations. These results indicated that diethylenetriamine was bonded to the surface of SWCNTs through an amidation reaction. The IR scanning patterns of SWCNT-DETA/Pd0 and SWCNT-DETA samples were almost similar, indicating that the structure of diethylenetriamine bonded to SWCNTs was preserved in the process of coordination and reduction. The shift peak observed at curve e (NH region) displayed the successful coordination of Pd2+ ions within the hybrid material. Also, the scanning electronic microscopy (SEM) analysis for SWCNT before (a) and after (b) functionalization with diethylenetriamine to immobilize palladium ions (Fig. 2) confirmed the successful functionalization of single-walled carbon nanotubes.
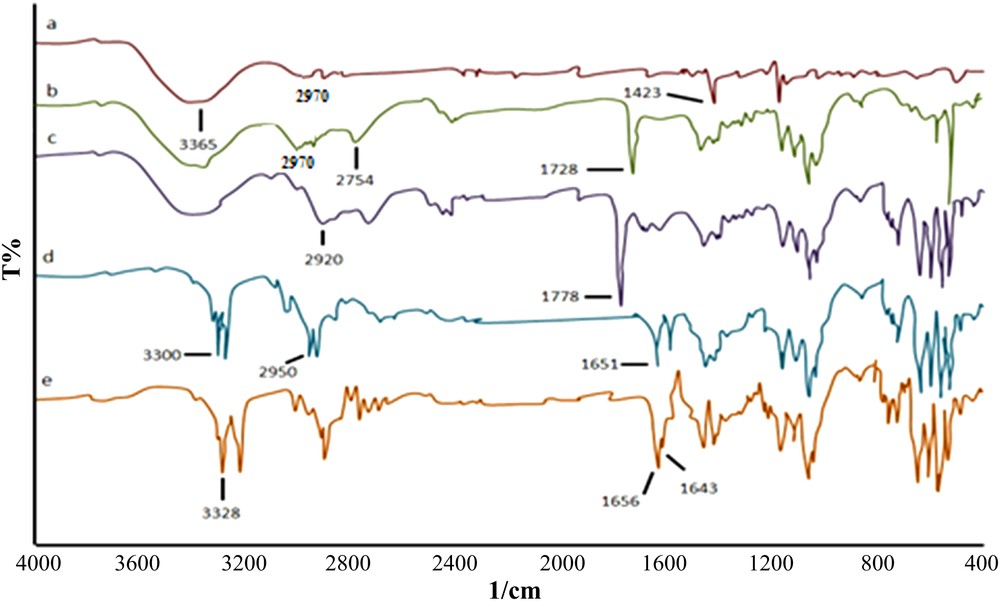
(Color online.) FTIR spectra of (a) SWCNT, (b) SWCNT-COOH, (c) SWCNT-COCl, (d) SWCNT-CO-DETA, (e) SWCNT-CO-DETA/Pd (II).
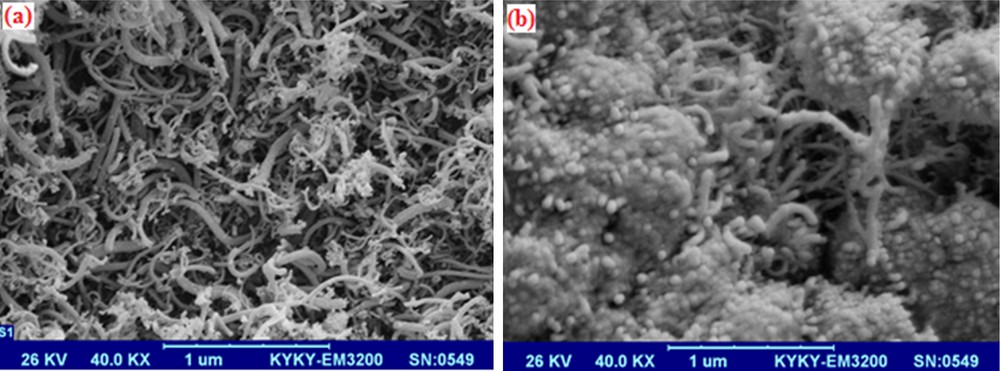
(Color online.) SEM images of SWCNTs before (a) and after (b) functionalization.
Transmission electron microscopy (TEM) investigations are carried out to observe the morphology and distribution of palladium particles supported on SWCNT-DETA/Pd. The existence of Pd nanoparticles, deposited on f-CNTs, was clearly distinguishable as dark spots in Fig. 3. From Fig. 3, we can see that Pd was well dispersed on the surface of polyamine-modified SWCNTs. The results indicated that polyamine plays an important role in improving the dispersibility of Pd. The mean diameter of Pd nanoparticles immobilized on f-CNTs was found to be around 5 nm.

TEM images of SWCNT-DETA/Pd.
Fig. 4 showed the X-ray diffraction spectroscopy (XRD) patterns of SWCNT-DETA/Pd0. As shown in Fig. 4, the wide diffraction peak at 2θ = 25° can be indexed to disorderedly stacked hexagonal graphite structure [31]. The well-defined peaks around 39°, 47°, 68° and 82° can be assigned to (111), (200), (220) and (311) crystal planes of Pd0 [14]. Thus the XRD results indicate efficient immobilization of fcc structured Pd nanoparticles on f-CNTs.

The XRD patterns for SWCNT-DETA/Pd0.
The thermogravimetric analysis (TGA) in inert atmosphere (N2) was used for the quantification of the diethylenetriamine on the surface (Fig. 5). These curves are related to SWCNT (curve “a” in Fig. 5), DETA (curve “b” in Fig. 5), SWCNT-DETA/Pd+2 (curve “c” in Fig. 5) and SWCNT-DETA/Pd0 (curve “d” in Fig. 5). The results showed that loss of solvent (such as water) starts at 100 °C, whereas carbon tubes and amorphous carbon decompose over ∼800 °C. Curves “c” and “d” show that several weight losses were observed for SWCNT-DETA/Pd+2. The first mass loss occurs in the temperature range from 100 to 250 °C, which is related to the loss of solvent trap in the complex. The second mass loss occurs in the temperature range from 450 to 550 °C, which is related to the loss of ligand (diethylenetriamine) in the complex; the third mass loss occurs at ∼800 °C and is related to the decomposition of CNT. The actual weight loss, corresponding to the combustion of supported diethylenetriamine, was determined at 24% by thermogravimetry as the weight loss between 450 and 550 °C at curves c and d. It is interesting to note that the calculated amount of ligand groups on the SWCNT-DETA hybrid material by thermogravimetric analysis was in good agreement with the loading of palladium nanoparticles.

(Color online.) Weight loss measured by TGA for (a) SWCNT, (b) DETA, (c) SWCNT-DETA/Pd+2, (d) SWCNT-DETA/Pd0.
To explore the catalytic activity, the Suzuki–Miyaura cross-coupling reaction of aryl halides with arylboronic acids was conducted with the present catalyst. The coupling rate depends on a variety of parameters such as solvent, base, temperature, and catalyst loading. For optimization of the reaction conditions, we chose the cross-coupling of 4-bromotoluene with benzeneboronic acid under aerobic conditions as the model reaction. The influence of various reaction parameters such as base (Et3N, AcONa and K2CO3), solvent (nonpolar, protic and aprotic), and catalyst amount on the reaction were tested. Among the bases evaluated, K2CO3 was found to be the most effective, and other bases were substantially less effective. We also investigated the effect of solvents on the Suzuki–Miyaura cross-coupling reaction and found that H2O/EtOH (1:1) was the best choice. We found that SWCNT-DETA/Pd+2 (10 mg, 1 mol% Pd) gave the optimum results using H2O/EtOH (1:1) as the solvent and 60 °C as the reaction temperature. Thus, the optimum conditions selected are: 4-bromotolune (1 mmol), phenylboronic acid (1.1 mmol), SWCNT-DETA/Pd+2 (10 mg, 1 mol% Pd), H2O/EtOH (2 mL, 1:1), K2CO3 (2 mmol) and 60 °C as the reaction temperature.
To survey the generality of the catalytic protocol, we investigated the reaction using various aryl halides coupled with arylboronic acids under optimized conditions. The results are summarized in Table 1. When benzeneboronic acid was coupled with aryl iodides and bromides containing both electron-donating and electron-withdrawing groups in para position, the corresponding products were obtained in excellent yields. The ortho-substituted aryl iodide, 2-iodoanisole (Table 1, entry 6), and aryl bromide, 2-bromoanisole (Table 1, entry 7) gave the corresponding products in slightly lower yields due to steric effects. The coupling reaction of phenyl chloride with benzeneboronic acid required extended reaction time vs. aryl iodides and bromides, giving the desired product in moderate yield (Table 1, entry 3).
Suzuki cross-coupling reaction of aryl halides with phenylboronic acid.
Entry | Aryl halide | Time (h) | Yield (%)a |
1 | 0.35 | 98 | |
2 | 0.7 | 98 | |
3 | 10 | 45 | |
4 | 0.5 | 96 | |
5 | 1.3 | 96 | |
6 | 0.5 | 85 | |
7 | 1.5 | 80 | |
8 | 0.75 | 95 | |
9 | 2 | 90 | |
10 | 1 | 95 | |
11 | 3 | 90 | |
12 | 0.8 | 98 | |
13 | 1 | 93 | |
14 | 1.75 | 92 | |
15 | 0.5 | 98 | |
16 | 1.5 | 96 | |
17 | 0.75 | 96 | |
18 | 1.5 | 95 |
Notably, when 2-iodothiophene and 2-bromothiophene were used as coupling partners, the desired product was obtained and no poisoning of palladium catalyst occurred (Table 1, entries 17, 18).
The activity of the catalyst was also superior with other reported heterogeneous catalysts for the reaction of aryl halides under aerobic condition. From Table 2, it can be seen that the present catalyst exhibited higher conversions and yields compared to the other reported systems [20,32–35].
Comparison of the activity of different catalysts in the Suzuki cross-coupling reaction.
Reaction | Catalyst | Reaction conditions | Yield (%) | Reference |
SWCNT-DETA/Pd+2 | K2CO3, H2O/EtOH, 60 °C, 22 min | 98 | This study | |
iodobenzene + phenylboronic acid | GO–NH2–Pd2+ | K2CO3, H2O/EtOH, 60 °C, 30 min | 87 | [32] |
Cell–OPPh2–Pd0 | K2CO3, EtOH, 78 °C, 20 min | 85 | [34] | |
Pd–ZnFe2O4 | K2CO3, EtOH, 78 °C, 4 h | 92 | [35] | |
Chitosan–Schiff base–Pd (II) | K2CO3, xylene, 130 °C, 6 h | 34 | [20] | |
SWCNT-DETA/Pd+2 | K2CO3, H2O/EtOH, 60 °C, 2 h | 90 | This study | |
p-nitrobromobenzene + phenylboronic acid | GO–NH2–Pd2+ | K2CO3, H2O/EtOH, 60 °C, 4 h | 80 | [32] |
PS–dtz–Pd (II) | K2CO3, H2O, 70 °C, 5 h | 80 | [33] | |
Chitosan–Schiff base–Pd (II) | K2CO3, xylene, 130 °C, 6 h | 76 | [20] |
The recyclability of the catalyst was determined for Suzuki reactions up to seven consecutive cycles (Table 3). The result indicated that the palladium leaching of the catalyst was low. Also, the results demonstrated that diethylenetriamine plays a key role in improving the stability of the SWCNT-DETA/Pd+2 catalyst.
Recyclability studya.
Run | 1st | 2nd | 3rd | 4th | 5th | 6th | 7th |
Conversion | 98 | 98 | 95 | 90 | 88 | 85 | 80 |
a Reaction conditions: bromobenzene (1.0 mmol), phenyl boronic acid (1.1 mmol), K2CO3 (2 mmol), H2O/EtOH (4 mL; 1:1), catalyst (10 mg), 60 °C.
In order to investigate the leaching of Pd during the reaction in our catalytic system, we conducted a filtration test for the Suzuki reaction between iodobenzene and phenylboronic acid using SWCNT-DETA/Pd+2 as a catalyst. After 10 min (the reaction was completed in 22 min), the reaction was stopped and the reaction mixture was centrifuged at 10,000 rpm for 10 min. Then the mixture without the solid catalyst was allowed to continue under the same conditions for another 12 min, and the conversion did not proceed significantly. This suggests that the leaching of Pd species from the solid support is low and the prepared catalyst is stable. On the other hand, atomic absorption spectroscopy of the filtrate also confirmed that the Pd content in the solution was below the detection limit (0.1 ppm).
4 Conclusion
In conclusion, a novel and recyclable palladium catalyst supported on diethylenetriamine-modified SWCNT was fabricated for the first time. The results indicated that the stability of the catalyst was much improved and SWCNT-DETA/Pd+2 was an efficient and recyclable catalyst for the Suzuki–Miyaura cross-coupling reactions in aqueous media under aerobic condition. The catalyst can be readily recovered and reused without significant loss of its catalytic activity. The proposed method can be a promising alternative approach for the preparation of biphenyl compounds.
Acknowledgement
We are thankful to Bu-Ali Sina University, Center of Excellence in Development of Environmentally Friendly Methods for Chemical Synthesis (CEDEFMCS) and Payame Noor University (PNU) for financial support of this study.