1 Introduction
The analysis of urinary stones is currently performed by physical methods, namely X-ray diffraction (XRD) or Fourier transform infrared spectroscopy (FTIR). These methods allow to identify the chemical nature of stone constituents and to provide semi-quantitative evaluation of their respective proportions within a calculus. This global analysis classifies calculi as mainly composed of calcium oxalate (CaOx), calcium phosphate (CaP), uric acid, struvite and cystine, which together represent approximately 98% of all urinary stones, with calcium stones accounting for more than 80% of the total [1]. Although they also allow to identify the crystalline phases of CaOx, namely CaOx monohydrate (COM) or whewellite and CaOx dihydrate (COD) or weddellite, this distinction is not provided in most laboratory records, which generically report ‘calcium oxalate’ without more precision, thus lacking a clinically relevant indication.
Physical methods reliably identify specific forms of nephrolithiasis involving a single component such as struvite, ammonium acid urate, cystine, 2,8-dihydroxyadenine (DHA), xanthine or drugs, because in this case, the chemical nature of the stones by itself defines the type of renal stone disease. However, these cases are infrequent, as they account for only 1–2% of the total in Western countries, whereas in the case of common calcium stones and, sometimes, of uric acid stones, simple identification of the chemical nature of stone constituents does not provide full etiopathogenic information.
Because XRD or FTIR analysis is usually performed on a powdered sample of the whole stone, no indication is provided as to the respective location (in the core or peripheral layers) of the diverse stone constituents in common calcium oxalate or phosphate calculi, which most often are of mixed composition, whereas the nature of the core, the initial site of stone formation, may differ from that of surrounding layers. In addition, the respective abundance and location of components are to be considered in CaOx or CaP mixed stones made of several crystalline phases such as COM, COD, carbapatite or brushite.
Moreover, a same elemental composition such as CaOx in the form of COM or COD as the main component may correspond to very different etiopathogenic conditions, each of which is reflected by a distinct morphology of both the surface and section of calculi, thus emphasizing the importance of morphological examination of stones in complement to analysis of their composition.
Accordingly, we developed, since the early 1980s, a comprehensive method of stone analysis termed morpho-constitutional because it combines the morphological examination of the surface and inner structure of the calculus with constitutional analysis by FTIR, the term constitutional encompassing the chemical nature, crystalline phases, relative proportions and location of the stones' chemical components.
2 The morpho-constitutional method of urinary stone analysis
The method we adopted for the morpho-constitutional stone analysis over the past four decades has been described in detail elsewhere [2]. In short, the standardized protocol comprises two steps:
- - First, a morphologic examination by means of a stereomicroscope (magnification ×10–40) of the surface and section of the calculus, with the identification of the nucleus (or core) and description of the inner organization, is carried out. The main points to be recorded in each stone are size, form, color, aspect (smooth, rough or spiky) of the surface, presence of a papillary imprint (umbilication), presence of Randall's plaque, aspect of the section (well organized with concentric layers and/or radiating organization, or poorly organized and loose structure) and location and aspect of the nucleus.
- - Thereafter, an analysis is performed by FTIR of a sample of each part of the calculus (nucleus, mid-section and surface), whenever allowed by the size of the stone, and in all cases, the determination of the global proportion of components in a powdered sample of the whole stone.
Using this method, urinary stones could be classified into 6 main morpho-constitutional types, themselves subdivided into a variable number of morphological subtypes, each corresponding to a peculiar etiological or pathophysiological condition. Indeed, the morphology of stones is determined by a number of factors, such as the size and shape of the constitutive crystals, the kinetics of stone growth and the location of the first steps of stone formation within the urinary tract [3]. For example, uric acid and struvite, which form large crystals and aggregates in urine, lead to rapidly growing and poorly organized stones. Conversely, COM stones, made of very small crystals, show a dense and organized structure.
The kinetics of stone growth also influence the inner structure of stones. Common COM calculi exhibit a well-organized inner structure with concentric layers and radiating crystallization, dark brown in color, which suggests a slow stone growth due to intermittent peaks of urinary oxalate concentration, whereas in primary hyperoxalurias, the genetic disorder causes a heavy, permanent overexcretion of oxalate ions, resulting in the formation of calculi made of the same COM component but with a very different, poorly organized inner structure and a different surface morphology and color. Indeed, the dark-brown surface of common COM stones results from the incorporation of pigments within the stone during a slow lithogenic process, whereas in primary hyperoxalurias, the permanent deposit of freshly produced COM crystals forms successive thin whitish layers at the surface of stones [3,4].
Correlations between etiopathogenic conditions and the corresponding morphological subtypes of CaOx, CaP and uric acid stones, as well as of mixed stones made of these components were established on the basis of more than 2000 stones analyzed at our laboratory, for which we had full information on patient's history and comorbidity and full evaluation of blood and urine chemistry.
We present below the morphologic characteristics and associated etiopathogenic conditions of the various subtypes of CaOX, CaP, uric acid and other purine stones, with their respective prevalence among a total of 70,000 calculi analyzed at our stone laboratory, located first at Necker Hospital (1985–2010)and now at Tenon Hospital. The main structural characteristics of the stones included in the morpho-constitutional classification of urinary calculi are summarized in Table 1.
Morpho-constitutional classification of urinary calculi.
Type | Main component | Surface | Section |
Ia | Whewellite | Mammillary surface. Frequent umbilication and Randall's plaque indicative of papillary origin. Color: brown. | Section made of compact concentric layers with a radiating organization. Color: brown. |
Ib | Whewellite | Mammillary and rough surface. No umbilication. Color: brown to dark brown. | Compact unorganized section. Sometimes, the presence of gaps. Color: brown to dark brown. |
Ic | Whewellite | Budding surface. Light color, cream to pale yellow-brown, sometimes whitish in children. | Finely granular and poorly organized section. Light color, cream to pale yellow-brown. |
Id | Whewellite | Smooth surface. Color: homogeneous, beige or pale brown. | Compact section made of thin concentric layers. Color: beige or pale brown. |
Ie | Whewellite | Locally budding, mammillary or rough surface. Color: often heterogeneous, pale yellow-brown to brown. | Locally unorganized and loose structure, locally more compact radiating structure. Color: heterogeneous, pale yellow-brown to brown. |
IIa | Weddellite | Spiculated surface showing aggregated bipyramidal crystals with right angles and sharp edges. Color: pale yellow-brown. | Section showing loose radial crystallization. Color: pale yellow-brown. |
IIb | Weddellite | Spiculated surface showing aggregated bipyramidal crystals with blunt angles and ridges. Color: pale yellow-brown. | Section showing compact unorganized crystallization. Color: pale yellow-brown. |
IIc | Weddellite | Rough surface. Color: gray-beige to dark yellow-brown. | Unorganized core with a diffuse concentric compact structure at the periphery. Color: gray-beige to dark yellow-brown. |
IIIa | Uric acid anhydrous | Homogeneous smooth surface. Color: homogeneous, typically orange, sometimes cream, ochre or yellowish. | Homogeneous compact, concentric structure with a radiating organization. Color: typically orange. |
IIIb | Uric acid dihydrate ± uric acid anhydrous | Heterogeneous embossed, rough and porous surface. Heterogeneous color from beige to brown–orange. | Poorly organized section with frequent porous areas. Color: orange. |
IIIc | Urate salts, including ammonium hydrogen urate | Homogeneous or slightly heterogeneous rough and locally porous surface. Color: homogeneous, cream to grayish. | Unorganized porous section. Color: whitish to grayish. |
IIId | Ammonium hydrogen urate | Heterogeneous embossed, rough and porous surface. Heterogeneous color: grayish to dark brown. | Section made of alternated layers, thick and brownish or thin and grayish, locally porous. Sometimes, locally purplish. |
IVa1 | Carbapatite | Homogeneous rough surface. Color: whitish to beige. | Section: poorly organized, or diffuse concentric layers. Color: whitish to beige. |
IVa2 | Carbapatite | Embossed and varnished surface with small cracks. Glazed appearance. Color: homogeneous, pale brown-yellow to pale brown. | Section made of compact alternated layers, thick brown-yellow and thin beige. Often, multiple nuclei (from collecting duct origin). |
IVb | Carbapatite + other calcium phosphates (±struvite) | Heterogeneous, both embossed and rough surface with confluent superficial deposits. Heterogeneous color: cream to dark brown. | Section made of irregularly alternating thick, whitish, and thin, brown-yellow layers. |
IVc | Struvite | Homogenous surface made of amalgamate crystals with blunt angles and edges. | Section: crude radial crystallization. Color: whitish. |
IVd | Brushite | Finely rough or dappled surface. Color: whitish to beige. | Radial crystallization with more or less visible concentric layers. Color: whitish to beige. |
Va | Cystine | Rough surface. Color: yellowish. | Section: poorly organized, sometimes a radiating organization. Color: yellowish. |
Vb | Cystine | Smooth surface. Color: homogeneous, cream to yellowish. | Concentric layers at the periphery, an unorganized core. Color: heterogeneous, cream (periphery) to yellowish (core). |
VIa | Proteins | Matrix soft calculi. Homogeneous surface. Color: cream to pale brown. | Unorganized section. Color: cream to pale brown. |
VIb | Proteins ± drugs or metabolic compounds | Heterogeneous, irregularly rough surface. Locally scaled. Color: dark brown to black. | Crude and diffuse foliated structure. Color: dark brown to black. Other components often present in these stones may alter the structure and the color. |
VIc | Proteins + whewellite | Homogeneous, smooth surface with clefts and scales. Color dark brown. | Section made of a dark brown protein shield surrounding a loose, unorganized light core containing whewellite crystals mixed with proteins. |
VII | Miscellaneous | Various morphologies and colors according to the stone composition (infrequent purines and drugs). | Variable organization and color according to the stone composition. |
3 Calcium oxalate stones
CaOx stones are divided into two types, according to their predominant crystalline form, either COM (type I) or COD (type II). Type I CaOx calculi present 5 morphologically distinct aspects (types Ia to Ie), whereas type II calculi present 3 morphologic aspects (types IIa to IIc). In our experience, type I calculi account for about 20% of all stones in France while type II calculi account for about 10%. Calculi made of a mixture of COM and COD (mixed types I+II) are as frequent as “pure” forms, as they represent nearly 20% of all stones.
3.1 COM calculi (type I)
Fig. 1 depicts the subtypes of type I calculi with surface and section morphologies and corresponding etiological conditions. The common pathogenic factor of type I stones is an elevated concentration of oxalate ions in urine without a concomitant elevation of urinary calcium. The degree and circadian duration of hyperoxaluria determine the morphological aspect of the diverse subtypes (Ia to Ie).
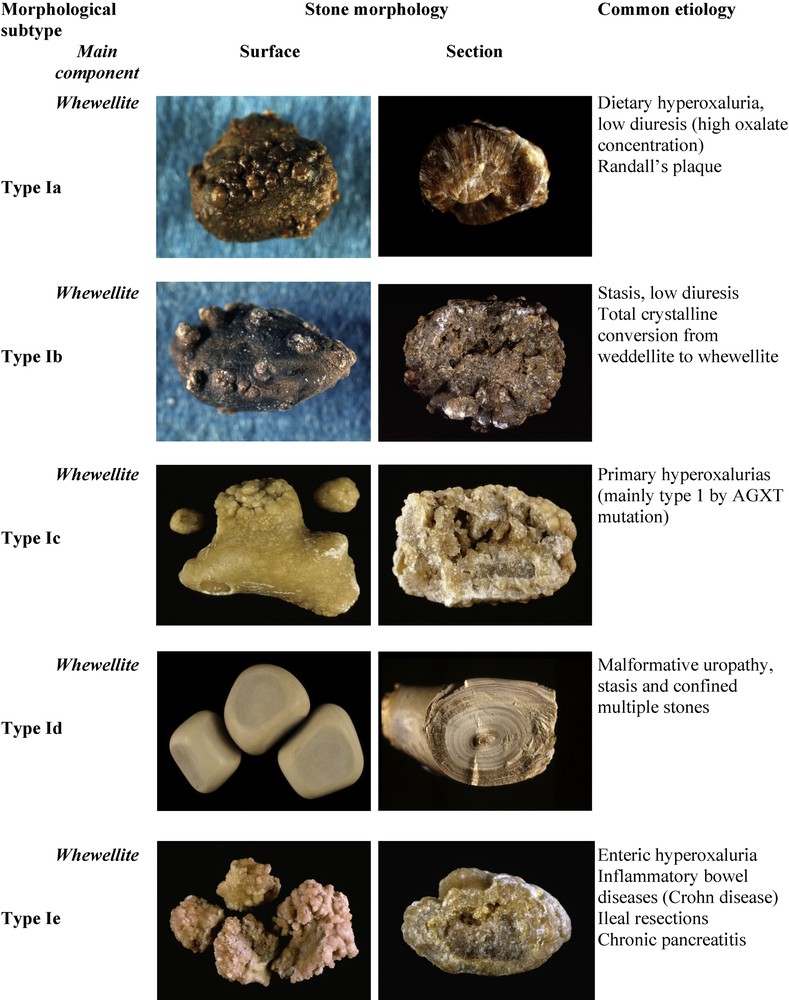
Main relations between type I stones and etiology.
3.1.1 Type Ia
Common, ‘idiopathic’ COM calculi present essentially as type Ia, which represents 88% of the type I group and nearly 18% of all stones in our experience. They are typically spherical, with a smooth, dark-brown surface and a section showing compact concentric layers with a radiating organization starting from a nucleus. Such a structure is indicative of intermittent deposition of COM crystals related to peaks of hyperoxaluria resulting from dietary habits. Three conditions may be involved in the intermittent and moderate excessive concentration of oxalate in urine: the most common cause is a low water intake resulting in low diuresis. Other dietary factors may be:
- - a high consumption of oxalate-rich foods like dark chocolate, beetroots, spinach, rhubarb, sorrel, star fruit, etc;
- - a high consumption of hydroxyproline-rich foods that induce a metabolic synthesis of oxalate by the liver;
- - a low intake of calcium, resulting in an increased absorption of oxalate ions by the gut.
Of note, most Ia stones are dark-brown in color because their growth is intermittent allowing urinary pigments to be fixed on the crystals. However, in some cases, the surface of Ia stones exhibit a very thin and often incomplete white layer made of very small COM crystals. Because the underlying layers are dark-brown, the thin superficial deposit appears as a grayish film, the significance of which being a new deposition of COM crystals as a result of a very recent peak of hyperoxaluria (Fig. 2). Thus, dark-brown Ia stones as shown in Fig. 1 may be considered as quiescent or metabolically inactive. By contrast, grayish Ia stones are discovered in an active stage.
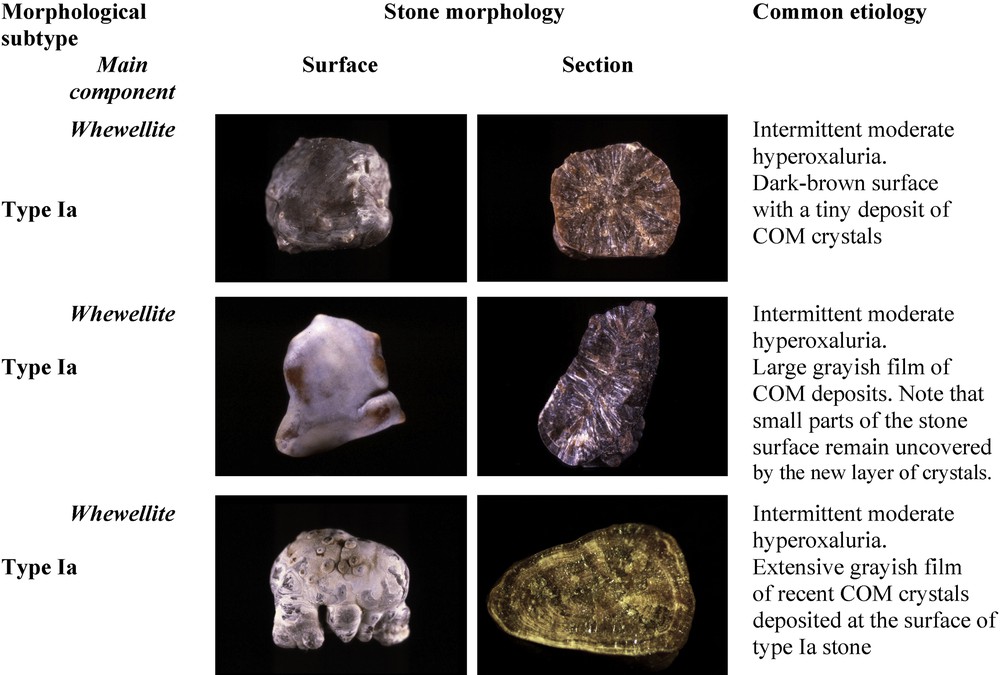
Examples of a grayish thin layer on the surface of type Ia stones.
A number of type Ia calculi exhibit an umbilication, i.e., a small depression at the stone surface which is the footprint of the tip of the papilla where the CaOx calculus formed and was initially attached, and containing a deposit of CaP named Randall's plaque [5–7].
3.1.2 Types Ib and Id
The types Ib and Id of idiopathic COM calculi are much less frequent as they represent respectively only 5.0 and 4.1% of the type I group in our experience (these proportions may be different in other populations or countries). Type Ib calculi have a brown to dark-brown, mammillary, rough surface with an unorganized section and they never exhibit an umbilication. This aspect is observed in conditions of moderate hyperoxaluria, stasis, and/or progressive crystalline conversion from COD to COM. Type Id calculi present a quite different morphology, with a very smooth surface, beige or pale brown in color, and a section showing thin concentric layers without radiations. These calculi often are many, as they form in conditions of stasis in confined cavities such as a diverticulum or hydronephrosis, their plane and smooth surface resulting from rubbing against each other.
The other two subtypes of COM stones (Ic and Ie) are very infrequent (less than 0.5% of cases), but of major clinical relevance.
3.1.3 Type Ic
Type Ic COM stones are, in our experience, pathognomonic of primary hyperoxaluria type I (PH1), the most severe of all forms of nephrolithiasis. Calculi are made of pure COM, similar to a number of idiopathic CaOx stones, but exhibit a very different, peculiar morphology characterized by a pale, yellowish budding surface (without any umbilication) and a loose, unorganized section. Scanning electron microscopy unequivocally confirmed the distinct crystalline structure of common-type idiopathic COM calculi and that of PH1-associated COM calculi [4]. Thus, the unexpected finding of a clear color in a stone made of pure COM should immediately alert to the probability of a PH1 or, at least, of a condition associated with heavy hyperoxaluria. Type Ic account for less than 1% of type I stones.
3.1.4 Type Ie
Type Ie stones are also indicative of specific pathogenic conditions associated with marked hyperoxaluria resulting from an intestinal dysfunction of diverse origins. This ‘enteric hyperoxaluria’ is observed in inflammatory bowel diseases, especially after ileal resection for Crohn's disease, in children with cystic fibrosis with a severe pancreatic deficit [8], and after bariatric surgery such as jejuno-ileal bypass or Roux-en-Y gastrojejunal bypass, which sometimes may result in heavy hyperoxaluria [9–13]. Type Ie calculi exhibit a locally budding, mammillary or rough brown-yellow pale surface, and a heterogeneous structure, with a mixture of poorly organized brown-yellow pale areas and of locally concentric dark brown layers with a radiating organization. Type Ie stones represent 2% of type I stones.
Of note, COM stones are not the unique form of stones that may develop in patients with inflammatory intestinal diseases or after intestinal surgery because stones may develop several years after the causal injury and result from different lithogenic factors [14]. Thus, in patients with past or current intestinal pathology, the morpho-constitutional analysis of stones is of clinical interest in order to identify the lithogenic factors acting in individual patients and guide the optimal therapeutic strategy.
Another pathological condition associated with the formation of type Ie stones is type 2 diabetes. About 6% of stones formed in diabetic patients exhibit such a morphology [personal data], thus suggesting that hyperoxaluria is, at least in a subgroup of these patients, a metabolic disorder associated with type 2 diabetes. In fact, a recent paper pointed out hyperoxaluria as a risk factor for stone disease in diabetic patients [15]. Various metabolic pathways involving carbohydrates and polyols may be entailed in the formation of metabolic precursors of oxalate such as glyoxal or glyoxylate [16–18].
3.2 COD calculi (type II)
Fig. 3 depicts the subtypes of type II calculi, surface and section morphology and associated conditions. The common pathogenic factor of type II stones is an elevated concentration of calcium ions in urine without marked elevation of urinary oxalate.
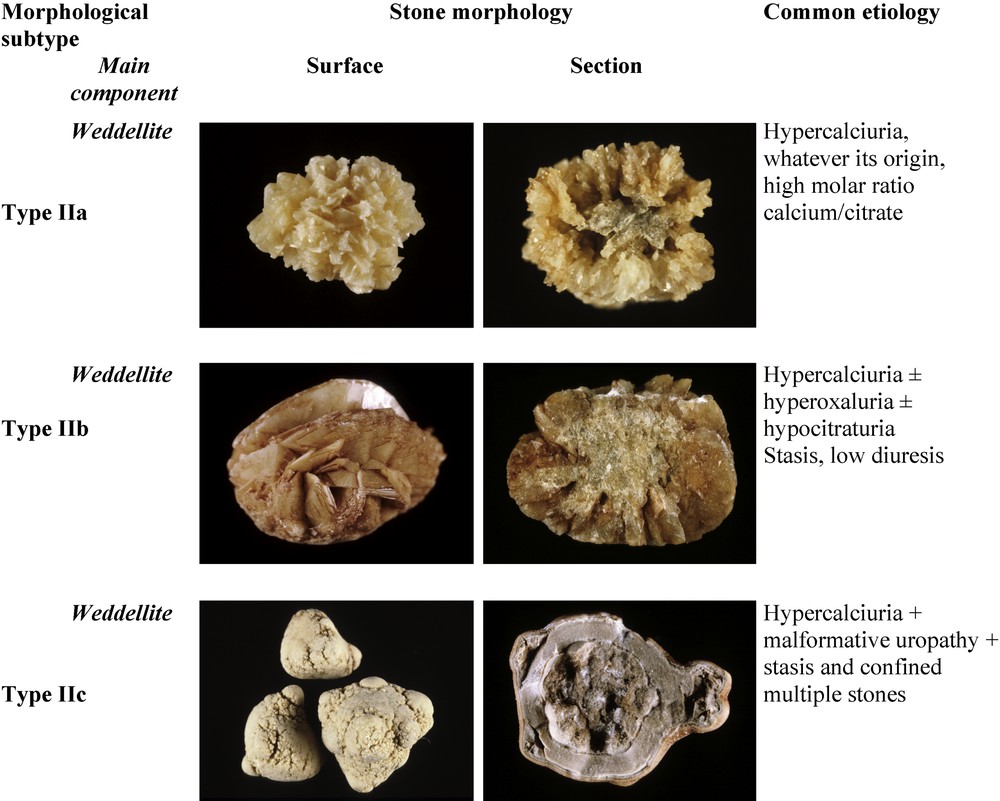
Main relations between type II stones and etiology.
3.2.1 Type IIa
Type IIa stones are the most commonly encountered, as they represent 69% of COD stones in our experience. Type IIa calculi have a yellow or light-brown prickly, spiculated surface due to the presence of aggregated bipyramidal crystals with sharp angles and edges. Their section shows a loose radial crystallization. They are observed in patients with idiopathic hypercalciuria.
3.2.2 Type IIb
Type IIb stones account for 30% of COD stones. They have a yellow or light-brown surface with smooth, long bipyramidal crystals, thus resembling small ‘desert roses’, and with a compact poorly organized crystalline section. They form in patients with both idiopathic hypercalciuria and moderate hyperoxaluria.
3.2.3 Type IIc
Type IIc stones are much less frequent (0.7% of COD stones). They have a rough gray-beige to pale brown surface, with a diffuse concentric structure at the periphery. They are observed in patients with both hypercalciuria and an obstructive anatomic anomaly with multiple stones resulting from stasis conditions.
3.3 Mixed COM and COD stones
Fig. 4 depicts mixed CaOx stones made of a mixture of types Ia and IIa or Ia and IIb. These mixed stones are nearly as frequent as pure CaOx stones; as in our experience, they account for 20.4% of the whole series as compared with 29.4% for ‘pure’ COM or COD stones [19]. They associate large aggregates of COD and COM crystals in variable proportions. They are observed in patients with both hypercalciuria and hyperoxaluria, most often of dietary origin. Because the lithogenic process is intermittent, COD crystals may partly convert to COM, the thermodynamically stable phase of calcium oxalate (Fig. 4C and D) [20]. As a consequence, the stone morphology may present with typical aspects of COD crystals overlapped by COM crystals. In a number of cases, these stones are initiated on carbapatite Randall's plaque at the surface of the tip of papilla (Fig. 4B, E and F). Of note, unlike COD, COM crystals bind specifically to Randall's plaque apatite.
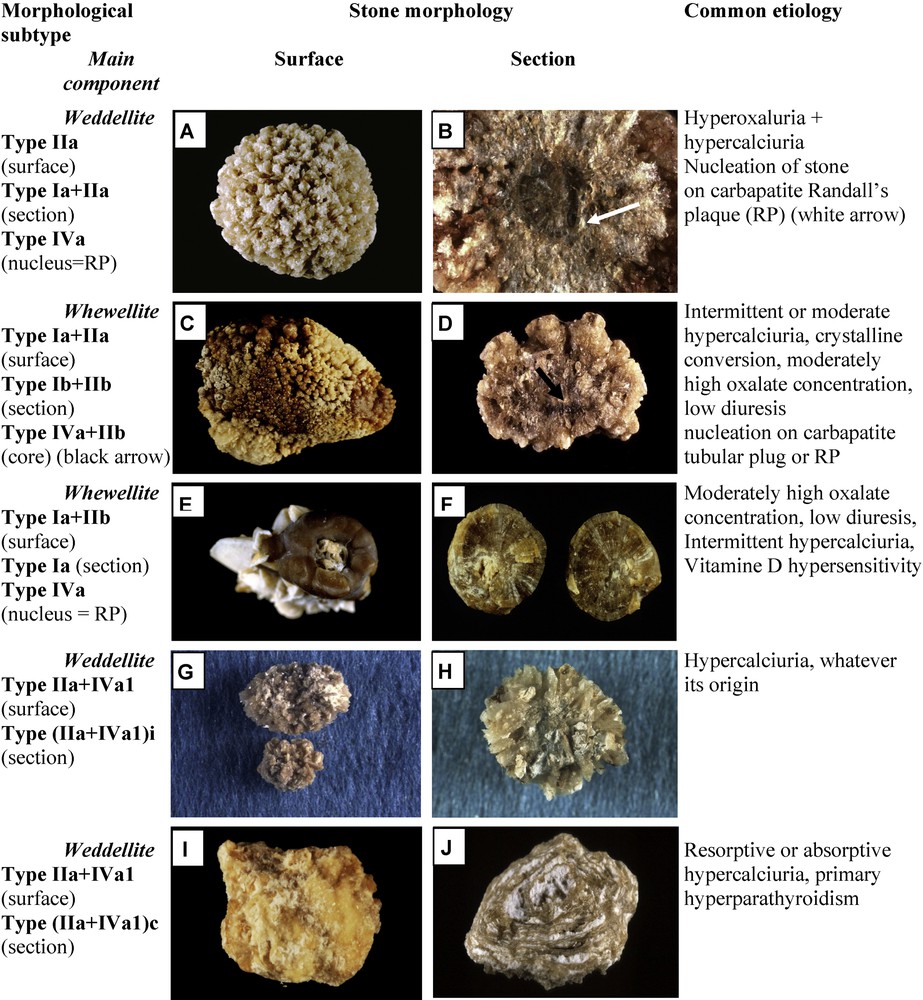
Main relations between mixed types of calcium stones and etiology. Note the presence of scattered pale deposits of carbapatite in the stone section (H) or alternated layers of carbapatite and weddellite in the section of another stone (J).
3.4 Calcium oxalate trihydrate (COT) or caoxite
Caoxite is the third crystalline form of calcium oxalate identified in urinary calculi and crystals [21]. Similar to COM, it is associated with hyperoxaluric states, but its scarcity in urinary calculi suggests that other factors are involved in its formation. Caoxite was observed in two very different situations: (i) CaOx stones induced by a drug prescribed for cardiovascular diseases [22] and (ii) CaOx stones formed in patients with primary hyperoxaluria [personal unpublished data]. In both cases, COT was mixed with COM. From a thermodynamic point of view, caoxite is a very instable form of calcium oxalate which spontaneously and rapidly converts to COM. In in vitro studies, caoxite was shown to transform to COM within a few hours [23]. Thus, the presence of caoxite in urinary calculi suggests that it is stabilized in the urine of affected patients. As suggested by Gardner et al. [24], anionic compounds of urine are able to inhibit caoxite formation, especially when they have a molecular weight above 13,000 Da. An imbalance between the promoters of calcium oxalate formation and macromolecules in urine may explain how caoxite crystals are observed in urine. Of note, in patients who experienced stone formation while on long-term treatment with piridoxilate-containing drugs, only those receiving Myocoril® exhibited caoxite crystals in urine or stones. The drug was a combination of two molecules, piridoxilate and pentaerythritol tetranitrate. By contrast, patients treated with Glyo-6®, a drug containing only piridoxilate, produced common whewellite calculi. The mechanism of stone formation first involved piridoxilate, an equimolar association of pyridoxine with glyoxylate, the direct precursor of oxalate, explaining hyperoxaluria as the main driving force in the urine of all affected patients, even if other metabolic factors were sometimes present such as hypocitraturia or hypercalciuria. In addition, an impairment of macromolecules by pentaerythritol tetranitrate could explain the presence and stability of caoxite in patients receiving Myocoril®.
4 Phosphate stones (type IV)
Stones having CaP as the main component, ie, containing at least 50% of CaP, account in our experience for 8.6% of the whole series and 21.7% of calcium stones. CaP stones are a very heterogeneous group because of their multiple crystalline forms (Fig. 5). At variance with CaOx stones, phosphate stones are pH dependent [25] and they are also calcium dependent [26]. Their common lithogenic factors are a pH of more than 6 and hypercalciuria, both of diverse degrees, resulting in diverse chemical compositions, crystalline phases and morphology.
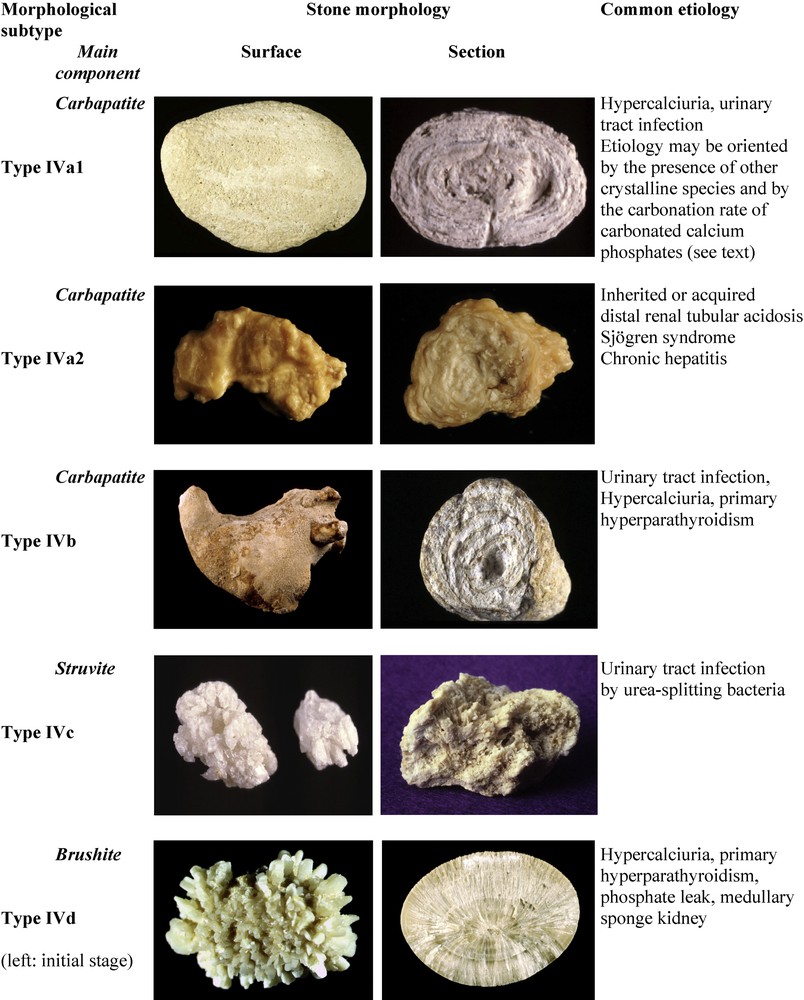
Main relations between type IV stones and etiology.
The most common crystalline phases found in CaP stones are carbapatite (carbonated apatite, often improperly termed apatite) and brushite (dicalcium phosphate dihydrate), respectively present as main components in 67% and 14% of phosphate stones, whereas struvite (magnesium ammonium phosphate hexahydrate), amorphous carbonated calcium phosphate (ACCP), octacalcium phosphate pentahydrate and whitlockite (mixed calcium and magnesium phosphate) are less frequent. With exception of brushite, which forms large crystals, all other phases of calcium phosphates form tiny particles made of nanocrystals less than 15 nm in size [7].
4.1 Common CaP stones
4.1.1 Type IVa1
Common CaP stones (type IVa1) are mainly composed of carbapatite, often admixed with small amounts of other components such as COM or COD. These stones exhibit a whitish, rough homogeneous surface and their section shows a poorly organized structure, with loose concentric layers of the same color as the surface (Fig. 5). Type IVa1 stones are mainly related to hypercalciuria and/or urinary tract infection.
4.1.2 Type IVb
Phosphate stones made of carbapatite admixed with some amounts of other calcium phosphates or struvite exhibit a different morphology (type IVb), with a heterogeneous surface, both embossed and rough, of clear to dark brown color. The section is made of alternate thick whitish and thin brown-yellow layers. These calculi are suggestive of past or latent urinary tract infection, especially if they contain small amounts of ACCP, whitlockite or struvite, which are markers of urinary tract infection (UTI). As a matter of fact, such phosphate stones are more frequent in women, who experience UTI more frequently than men. In some cases, these stones may be related to a minor defect in tubular acidification, or hypercalciuria, especially when they also contain COD.
4.2 Struvite stones
Stones made mainly of struvite (type IVc) account for 1.2% of the whole series and their incidence relentlessly declined over the past decades in industrialized countries [27]. Struvite stones exhibit a whitish surface, homogeneously made of aggregates of large crystals with blunt angles and edges; the section shows a diffuse, loose radial crystallization (Fig. 5). These stones unequivocally result from UTI by urea-splitting microorganisms. Of note, the presence of struvite, even in a small amount, is the feature of UTI by urease producing bacteria. In our experience, we found struvite in 7.2% of all stones analyzed in our laboratory.
4.3 The type IVa2 carbapatite subtype and distal renal tubular acidosis
Certain carbapatite stones exhibit a quite distinct morphology from that of common CaP stones, although having the same chemical composition and similar spectra on FTIR [28,29]. Therefore, we subdivided type IVa into two subtypes in order to differentiate common-type carbapatite stones (type IVa1) from this peculiar subset of carbapatite stones (type IVa2) which in our series represents 11.5% of CaP stones.
Type IVa2 calculi exhibit a very peculiar morphology characterized by a yellow-brown, smooth surface with a glazed appearance and small cracks, and a section showing irregularly arranged thin whitish and thick yellow-brown concentric layers (Fig. 5). Scanning electron microscopy confirmed the very different structure of types IVa1 and IVa2.
This type of CaP stones appears to be specifically formed in the context of defective distal tubular acidification, as it was observed in our experience in 95% of patients with congenital distal renal tubular acidosis (dRTA) and in 65% of CaP stones from patients with Sjögren's syndrome, and also in severe forms of medullary sponge kidneys, which induce injury of collecting ducts and localized acidification defects. However, stone formers suffering from Sjögren's syndrome may form CaOx stones and mixed CaOx+CaP stones. The same is true of the majority of patients with MSK, who often have pleiomorphic stones (personal data).
Patients with dRTA have been shown to form calculi predominantly made of carbapatite [30–32] and plugging of Bellini's ducts with apatite crystals has been evidenced in patients with dRTA [33]. An association between Sjögren's syndrome, distal tubular acidosis and carbapatite has also been reported [32,34,35], but without an indication as to the morphology of calculi.
4.4 Brushite stones (type IVd)
Stones mainly composed of brushite represent 1.3% of the total series and 14.4% of CaP stones. Brushite calculi at their initial stage exhibit large rod-shaped crystals, thereafter evolving toward slightly rough or resembling a cabbage, whitish or beige surface and a section showing radial crystallization with locally concentric layers (Fig. 5). Brushite stones are clearly both calcium dependent and pH dependent [26,36] and are observed in patients with marked hypercalciuria and a urinary pH above 6 (6.4 as a mean in our series). Brushite nephrolithiasis usually presents as idiopathic, but interestingly, type IVd stones were observed in at least 14% of patients with primary hyperparathyroidism [26].
The identification of brushite among CaP stones is meaningful because brushite stones, at variance with common carbapatite stones, are resistant to ESWL and have an especially high activity and recurrence rate [37,38].
4.5 The carbonation rate of carbapatite
The ratio of carbonate ions relative to phosphate ions, or carbonation rate (CR), in carbapatite stones may be determined as the ratio of the intensity of their respective ν3 bands on FTIR spectroscopy. The high carbonate content of struvite stones, induced by urease-producing bacteria, is well established [39].
In carbapatite stones devoid of any struvite, we observed a high CR (≥15%) in calculi containing predominantly carbapatite (type IV) associated with small amounts of ACCP, in which scanning electron microscopy identified bacterial imprints, either rod-like or round, at the surface of spherules of carbapatite, suggestive of the past presence of bacilli or cocci. As a confirmation, a history of UTI was found in these patients [40]. The possible implication of UTI in the formation of CaP stones made of carbapatite without struvite, including by non-urease producing enterobacteria such as E coli, has been suggested in previous studies [41–44].
In contrast, a low CR (7% as a mean) was found in calculi made of mixed carbapatite and COD, without ACCP and bacterial imprints, in hypercalciuric patients devoid of a history of UTI [40]. Thus, a low CR value (<10%) in carbapatite stones suggests a metabolic origin without the participation of UTI, whereas a high CR value (≥15%), especially if associated with the presence of ACCP, strongly suggests that UTI was involved in the lithogenic process.
4.6 Mixed CaOx and CaP stones
Stones composed of a mixture of CaOx (COD and/or COM) and carbapatite (without struvite) are twice more frequent than phosphate stones containing only CaP as the main component. They accounted for 19.7% of the total number of stones in our series, as compared to 8.2% for stones having carbapatite as the main component. The commonest forms of mixed phosphate–oxalate stones are stones exhibiting a dual morphology (IIa+IVa1 or IIb+IVa1), which together accounted for 12% of the total series (Fig. 4), and stones exhibiting a complex heterogeneous morphology (type I+II+IV) which accounted for 6% of the total series (Fig. 6). The dual morphology IIa/b+IVa1 is mainly related to hypercalciuric states, irrespective of their origin. The carbapatite content may influence strongly the inner structure of the stones. Commonly, when it is below 20%, the stone morphology is mainly driven by the poorly organized agglomerates of COD crystals with CaP deposits in the spaces between crystal aggregates. Such a morphology, named (IIa+IVa1)i (“i” for “inorganisé”) is shown in Fig. 4H. By contrast, a high carbapatite content (20 ≤ carbapatite < 70%; these thresholds are not absolute limits) commonly results in another organization, named (IIa+IVa1)c (“c” for “concentrique”), characterized by irregular and alternate layers of carbapatite and weddellite as shown in Fig. 4J.
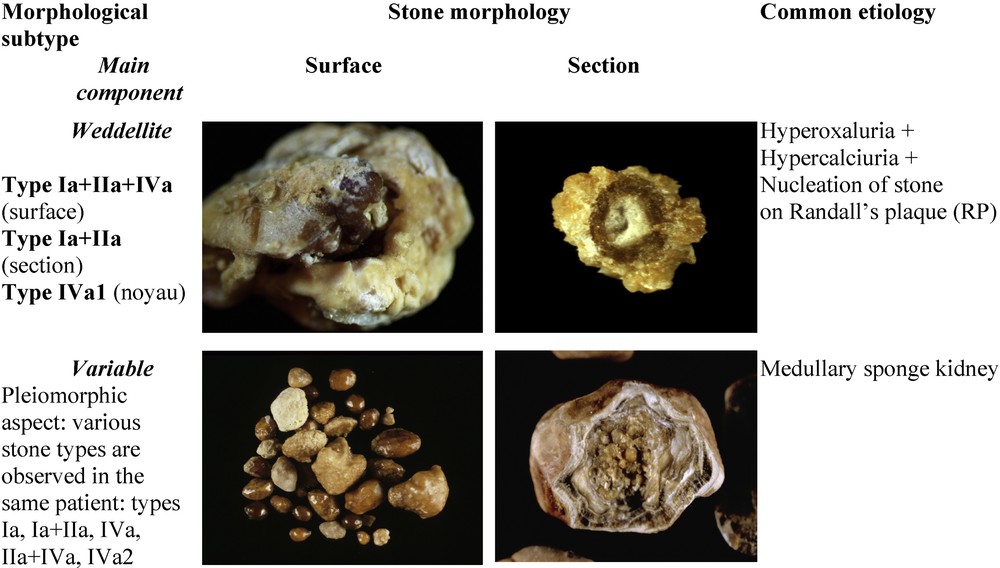
Main relations between ternary mixed types, polymorphs of calcium-containing stones and etiology.
Mixed CaOx/CaP calculi most often present as idiopathic, in a context of both hypercalciuria and relatively high urine pH [30,45]. However, they may also reflect specific etiologies, particularly medullary sponge kidneys (MSK) and, less frequently, primary hyperparathyroidism [26,31,46].
In our series, nearly 60% of calculi from patients with primary hyperparathyroidism exhibited a mixed II+IVa morphology, with carbapatite having a low (<10%) carbonation rate. Some calculi also contained COM, but never exhibited a type I morphology, whereas other stones had COD (12%), carbapatite (12%) or brushite (14%) as the main component. Interestingly, type IVd stones mainly composed of brushite and mixed stones of II+IV type together account for more than 72% of stones associated with primary hyperparathyroidism [46]. A predominant content of CaP in stones produced by patients with primary hyperparathyroidism has also been reported by other authors [45,47,48] but without the mention of the diverse composition and morphology of the calculi.
In patients with MSK, stones were most often composed of CaOx (COM or COD) but also of CaP in the form of carbapatite of IVa1 morphology often admixed with COM or COD, or of IVa2 morphology [29]. A same patient may produce calculi of diverse CaOx or CaP composition, this pleiomorphism being suggestive of MSK (Fig. 6).
In addition, phosphate stones may be produced in patients with rare hereditary monogenic diseases characterized by a urinary phosphate leak often associated with hypercalciuria. These diseases affect the proximal tubule such as Dent's disease (or X-linked hypercalciuric nephrolithiasis) and related syndromes [49–51], hereditary hypophosphatemic rickets with hypercalciuria induced by inactivating mutations of the proximal tubule sodium/phosphate co-transporters [52–54], or mutations of genes coding for paracellin 16 or 19, responsible for familial hypercalciuria with hypomagnesemia and nephrocalcinosis (FHHNC) [55,56]. In hereditary hypophosphatemic rickets resulting from mutations associated with increased circulating levels of FGF23, which induce a marked phosphate leak, the palliative treatment of profound hypophosphatemia with calcitriol adds hypercalciuria to hyperphosphaturia and may induce the formation of CaP stones [57,58]. In these diseases, nephrolithiasis is associated with nephrocalcinosis made of tubulo-interstitial deposits of CaP crystals in the renal parenchyma, which is the revealing sign more frequently than nephrolithiasis, inasmuch as these diseases manifest early in life with marked, symptomatic disorders of calcium-phosphate homeostasis.
4.7 Etiopathogenic orientations based on the morphology of CaP stones
Identification of the various subtypes of CaP stones suggests etiopathogenic hypotheses that orient biochemical and genetic investigations.
CaP stones having carbapatite (without any struvite) as the main component (type IVa1) are observed in common idiopathic CaP nephrolithiasis, mainly observed in women, but also suggest the search for past or current UTI involving non-urea-splitting bacteria especially if some amount of whitlockite or ACCP is also present (Fig. 7) [40].
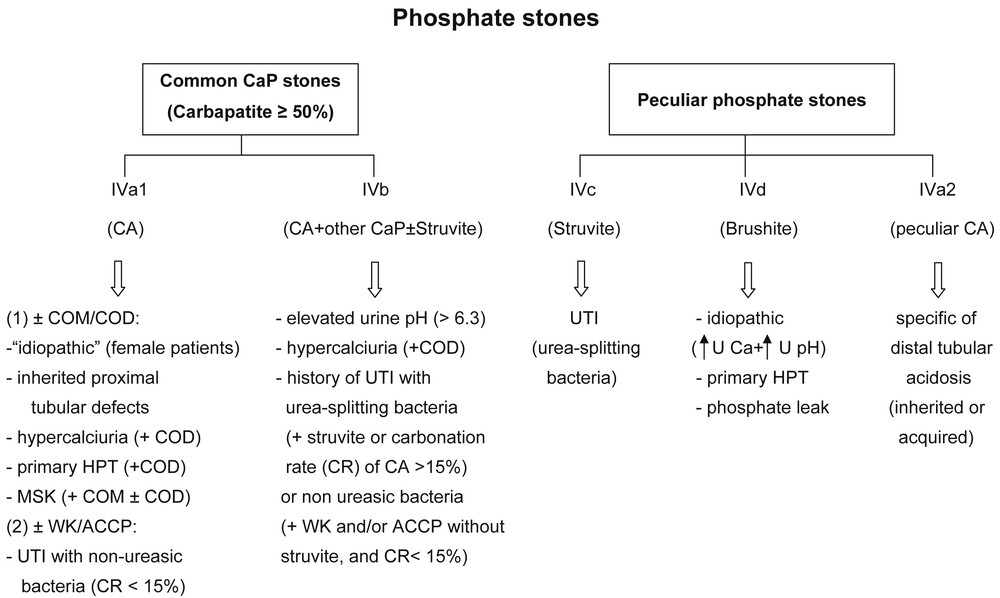
Main relations between phosphate stones and etiology according to their morphology and composition. Abbreviations: CaP = calcium phosphate; CA = carbapatite; COM = calcium oxalate monohydrate; COD = calcium oxalate dihydrate; CR = carbonation rate; MSK = medullary sponge kidney; UTI = urinary tract infection; WK = whitlockite; ACCP = amorphous carbonated calcium phosphate; U Ca = urinary calcium; U pH = urine pH.
In patients with nephrocalcinosis, type IVa1 carbapatite stones suggest a proximal tubulopathy such as Dent's disease or some form of hereditary hypophosphatemic rickets. Stones predominantly composed of type IVa1 carbapatite or of a mixture of CaOX and CaP (types IVa1+IIa or IIb) (Fig. 4) are observed in half of the patients with primary hyperparathyroidism, who also form type IVd (brushite) stones in 15–20% of cases in our most recent evaluation.
Type IVa2 carbapatite stones specifically orient toward distal renal tubular acidosis of genetic origin (various forms of hereditary dRTA) or associated with Sjögren's syndrome, which may be revealed by nephrocalcinosis besides the usual extrarenal symptoms such as eye or mucosa dryness [29]. MSK with localized defects of distal tubular acidification is another cause of type IVa2 stones. Usually, in MSK, other types of calculi are observed in the same patients in addition to IVa2 stones.
4.8 The clinical relevance of minor stone component identification for etiological diagnosis
Identification of the minor components associated with carbapatite contributes to etiological diagnosis. Presence of brushite, COD or octacalcium phosphate pentahydrate is suggestive of hypercalciuria while ACCP or whitlockite orient towards urinary tract infection. Mixtures of CA and COM are common in MSK.
It is noteworthy that stones may stay for a long time within the kidney and that successive pathological conditions may participate in stone growth. Therefore, it is clinically relevant to take into account the composition and morphology of the different areas of the calculus. For example, a stone containing more than 80% of COM with a cross-section of type Ia morphology suggests moderate hyperoxaluria of dietary origin as the driving force of the lithogenic process, whereas a stone surface mainly composed of a mixture of COD and CA (mixed type IIa+IVa1), is suggestive of a recent, new lithogenic condition, such as primary hyperparathyroidism inducing hypercalciuria.
5 Uric acid and urate stones (type III)
5.1 Common uric acid stones
Common uric acid (UA) stones present two distinct morphologies (Fig. 8).
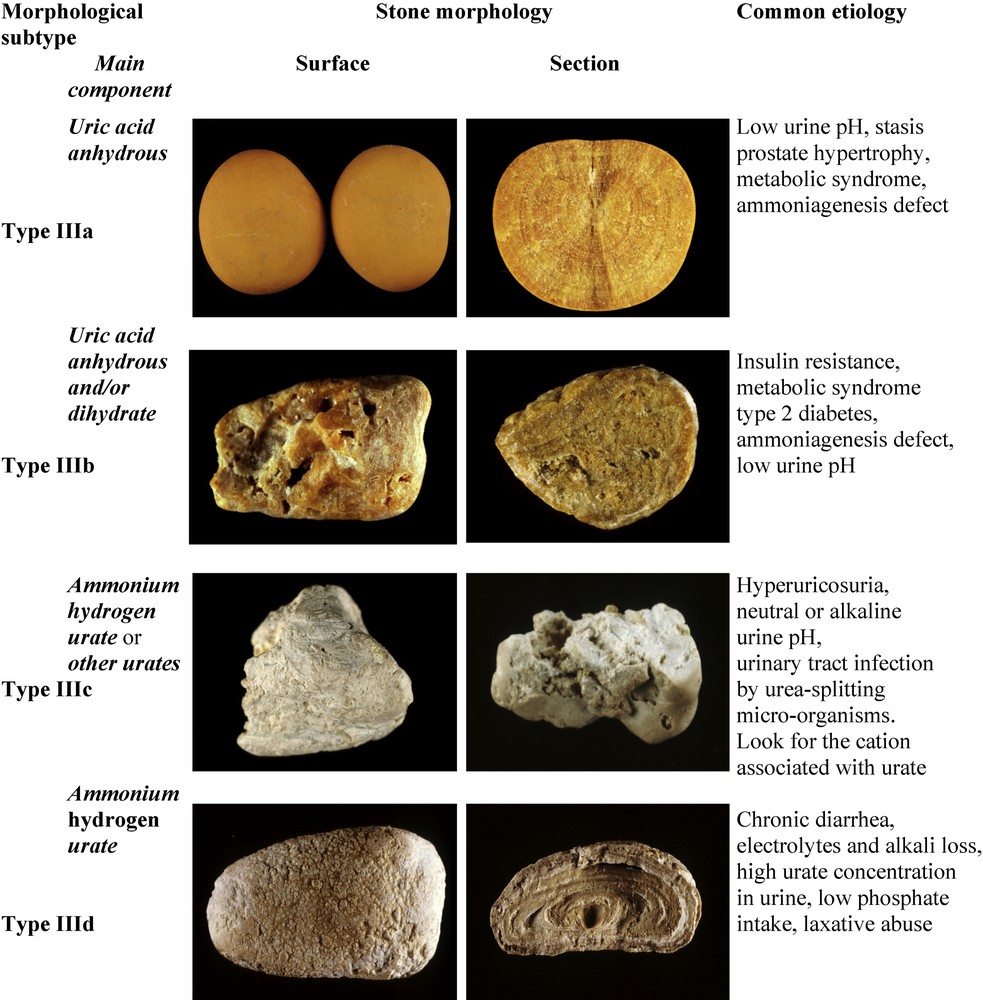
Main relations between type III stones and etiology.
Type IIIa stones, mainly composed of anhydrous UA exhibit a smooth, typically orange surface with a section showing concentric layers with a radiating organization around a well-defined nucleus. They account for 1.6% of the whole series and are mainly seen as bladder urolithiasis in older men with benign prostate hypertrophy. Of note, the typical orange color of uric acid calculi results from a particular affinity between uric acid crystals and a red pigment naturally present in urine and named uricine [59]. However, due to a low content of uricine in urine or because of a poor adsorption of the pigment at the surface of uric acid crystals, perhaps depending on urine pH, the stone surface of uric acid calculi may appear moderately colored and sometimes entirely whitish while inner layers always exhibit some degree of pigmentation, ranging from pale yellow to ochre.
Type IIIb calculi, predominantly made of UA dihydrate, exhibit a rough and porous surface with a heterogeneous, beige to orange color and an orange-red section with a poorly organized, porous structure. Of note, because UA dihydrate is unstable with time and spontaneously converts to anhydrous UA, IIIb calculi initially formed as UA dihydrate may be composed mainly of anhydrous UA at the time of analysis, although retaining a IIIb morphology.
Type IIIb calculi are the most frequently encountered as they represent 77% of pure UA stones and they account for 7–8% of all stones. They are strongly pH dependent and form in acidic urine (pH < 5.5) even in normouricosuric patients [60], but more so if hyperuricosuria is present. This type of UA stones is most often observed in patients with gout, metabolic syndrome, or type 2 diabetes who have both hyperuricosuria and acidic urine [61–63]. In these patients, the low urinary pH reflects reduced ammonia excretion resulting from insulin resistance, the common metabolic disorder underlying these conditions [64]. UA stones also are produced in cases of chronic hydro-electrolytic diarrhea in patients with inflammatory bowel diseases, especially after an ileostomy which results in a heavy loss of water and bicarbonates and thus in a low-volume urine with very low pH.
The association between type 2 diabetes and UA nephrolithiasis is two way. In our experience, UA stones were three times more prevalent in type 2 diabetic stone formers than in non-diabetics, whereas reciprocally prevalence of type 2 diabetes was 2–3 times higher among UA than calcium stone-formers [63,65]. Therefore, the onset of UA nephrolithiasis in an adult patient should prompt a search for latent type 2 diabetes, especially in the case of obesity.
5.2 Mixed uric acid–calcium oxalate stones
Mixed stones made of uric acid (type IIIb) and CaOx (COM, type Ia) are not infrequent. They are observed in patients, mostly obese and overeaters, who cumulate risk factors of both uric acid and COM formation. This form of mixed CaOx and UA nephrolithiasis is especially severe and recurrent [66]. Fig. 9 illustrates various forms of these associations.
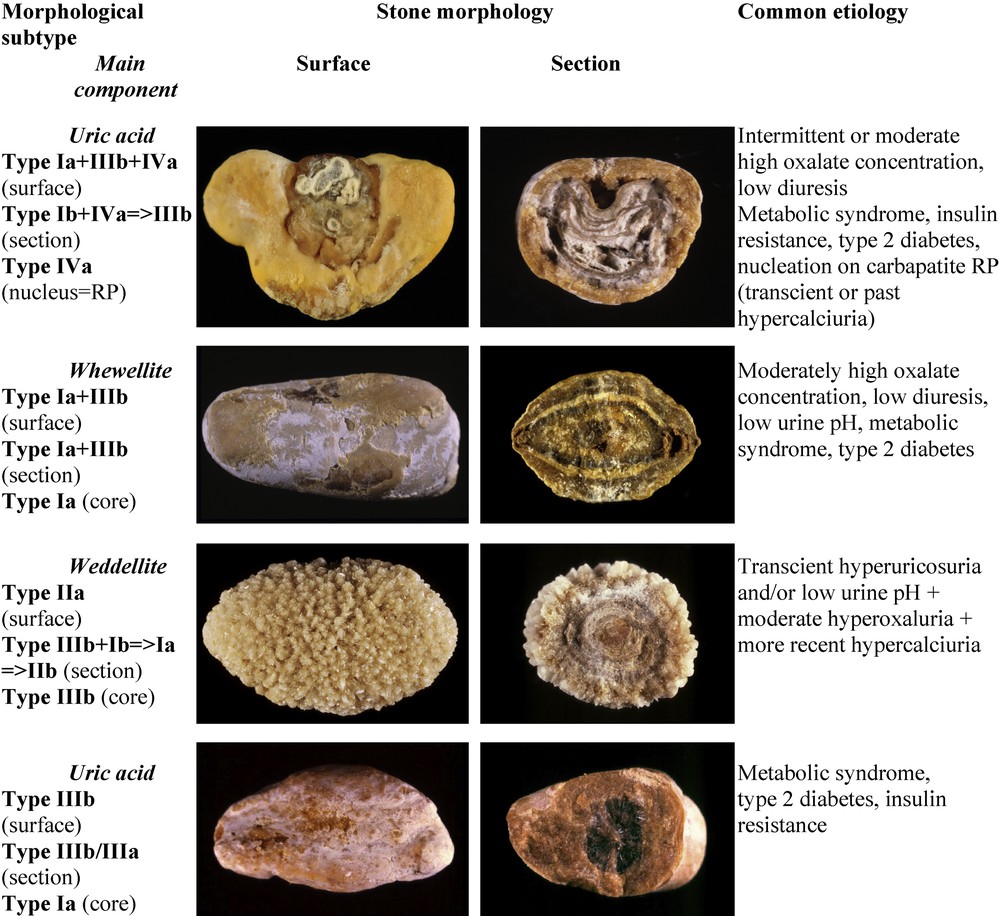
Main relations between mixed types of calcium oxalate–uric acid stones and etiology.
5.3 Ammonium urate stones
Ammonium urate stones are very infrequent, as they account for less than 0.4% of all calculi in our series. They are still frequent in other parts of the world such as Africa or South-East Asia [67] although their prevalence appears to be decreasing in most of affected countries [68,69]. They exhibit two different aspects.
Type IIIc stones have a rough and locally porous beige or grayish surface, and an unorganized porous section of the same color (Fig. 8). They are composed of various urate salts, most often ammonium hydrogen urate, which is the least soluble form of urates. They crystallize in urines of very high pH (around 8) in the context of UTI with urea-splitting bacteria which result in the local production of ammonium ions from urea, and are mainly observed in developing countries. In Western countries, they also may result from excessive therapeutic urine alkalization.
Type IIId stones have a heterogeneously rough and porous surface with a dark-brown color, and a section showing alternatively thick, brown and thin, clear layers with small porous zones (Fig. 8). They are predominantly or totally made of ammonium hydrogen urate. Commonly, they result from chronic diarrhea of infectious origins in subjects eating an imbalanced diet characterized by high fibers (cereal) and low phosphorus (animal proteins) consumption.
In Western countries, type IIId stones are observed in the peculiar context of chronic laxative abuse, which induces chronic occult diarrhea and high urinary ammonia excretion, compensating for the diminished titratable acidity in anorectic patients (mostly young women) with very low protein, and thus a low phosphate intake. Such a composition of stones is virtually specific of laxative abuse [70]. In developing countries, type IIId calculi are mainly found in children (mostly boys) with endemic bladder urolithiasis [71]. Ammonium urate is still found as the major component of the nucleus of stones in these countries, although urinary stones mainly composed of ammonium urate have become infrequent [72].
Ammonium urate may be admixed with uric acid and/or calcium oxalate, resulting in a heterogeneous morphology (Fig. 10). In most cases, it is the mark of changes in risk factors or diseases involved in stone formation. It is then very important for diagnosis to consider successive layers of the stone from the core to the surface. An example is provided by the stone shown at the top of Fig. 10. The photograph on the left side exhibits a typical Ia+IIIb structure which corresponds to a mixture of COM and uric acid. Such a finding is highly suggestive of a metabolic syndrome with low urine pH and commonly normal uric acid excretion and concentration. However, the section of the stone (top right) displays a large poorly organized grayish core made of ammonium hydrogen urate (type IIIc). This compound cannot be formed in very acidic urine as does uric acid. In addition, ammonium urate crystallization requires a high urate concentration. In our experience, ammonium urate in the form of the IIIc morphology is commonly observed in alkaline urine with a high ammonium content as found in urinary tract infection by urea-splitting bacteria. Thus, these conditions prompt to look for another biochemical environment than that associated with the metabolic syndrome. It is worth noting that the intermediate layer of the stone is made of almost pure COM in the form of type Ia. This part of the stone is a marker of changes in urinary pH with time.
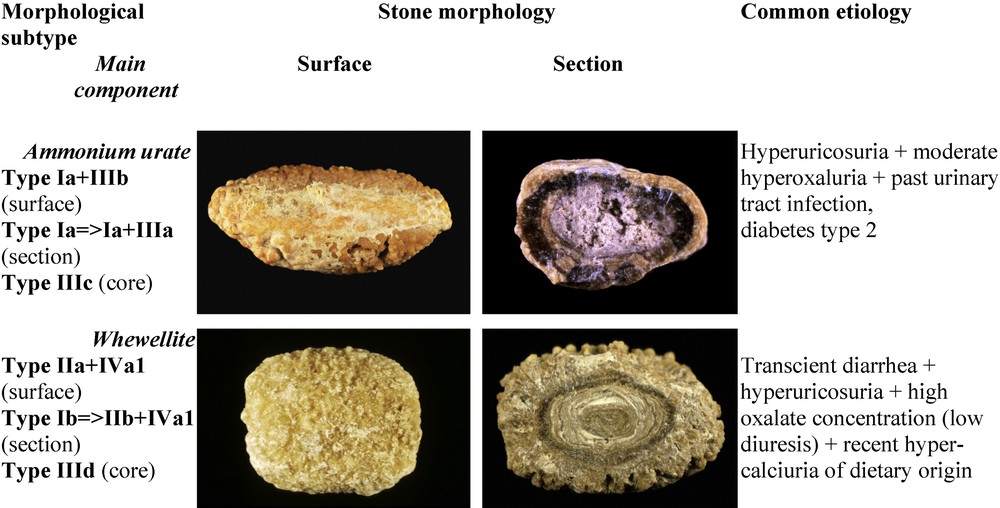
Main relations between mixed types of calcium oxalate-uric acid-ammonium urate stones and etiology.
At the bottom of Fig. 10 (left), a mixed stone of type IIa+IVa1 morphology corresponds to an association of weddellite and carbapatite. It appears as a marker of hypercalciuria. However, the section of the stone is heterogeneous (Fig. 10, right). The core is made of ammonium hydrogen urate with a IIId morphology. In our experience, such a morphological type is related to hyperuricosuria associated with chronic diarrhea and hydro-electrolytic losses. The change from ammonium urate to COM in the intermediate layer suggests a transient episode of diarrhea initiating the stone. Thereafter, ammonium urate was surrounded by COM as a result of high oxalate concentration mainly related to very low diuresis. Finally, the stone growth was continued by the deposition of a mixture of COD and carbapatite, suggestive of hypercalciuria. The cause was a marked change in life style conditions and dietary habits of the patient who developed dietetic hypercalciuria by excessive dairy product consumption.
6 Other stones
Infrequent stones made of cystine, proteins, rare purines or drugs exhibit specific morphological types as observed for common stones. However, in these types of nephrolithiasis, the composition of stones is more informative than morphology, although, in some cases, stone morphology may help to orient toward specific lithogenic conditions.
6.1 Cystine stones
Cystine calculi account for less than 1% of all stones and they are usually made of pure cystine. Nevertheless, they have two distinctive morphologies named type Va (the most common one) and type Vb (Fig. 11). Type Va stones are about ten times more frequent than type Vb stones. Because basal medical treatment for the prevention of cystine stones is urine alkalization, in 5–10% of patients with recurrent stones, the presence of small proportions of calcium phosphate, mainly in the form of carbapatite or ACCP, may be observed within the new cystine stones produced under medical treatment.
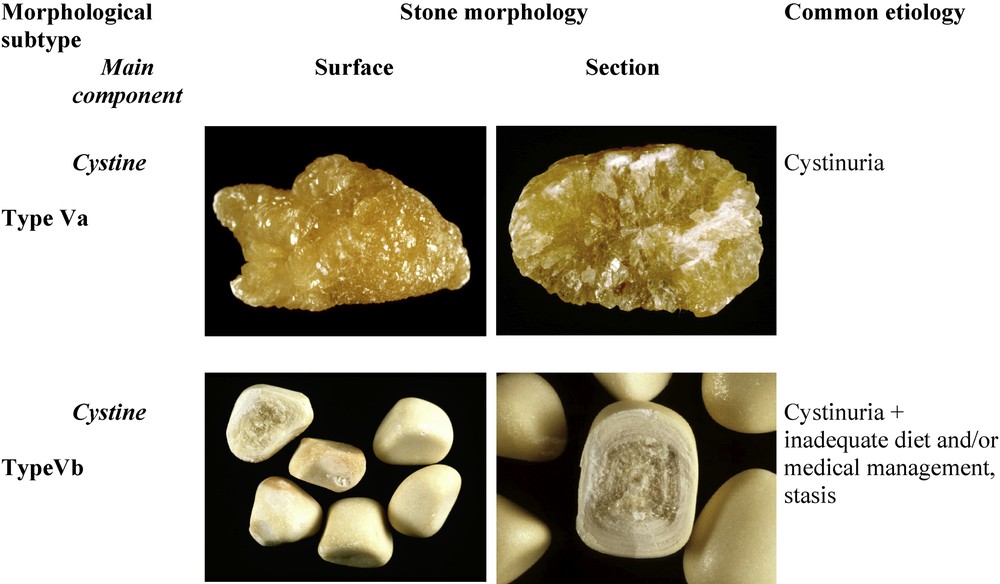
Main relations between type V stones and etiology.
6.2 Protein stones
Calculi mainly made of proteins are infrequent. They may be encountered in three main clinical contexts: (i) chronic pyelonephritis related to urinary tract infection; (ii) proteinuria observed in glomerular kidney diseases, hematuria or crystal-induced bleeding; and (iii) end-stage renal failure.
Chronic pyelonephritis may result in the formation of soft matrices spreading into all kidney cavities, pyelons and calices. The typical morphology of such soft calculi is type VIa (Fig. 12). Of note, the stones are light-brown in color in contrast with other types of protein-rich calculi.
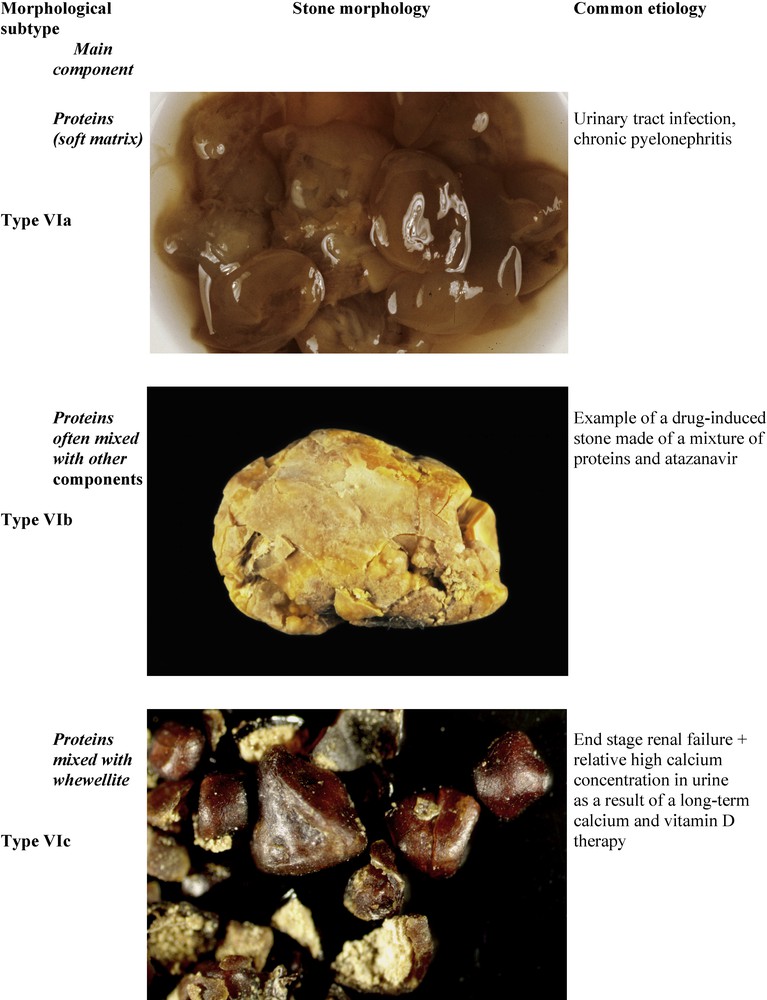
Main relations between type VI stones, high protein content and etiology.
Stones produced by patients with heavy proteinuria and/or hematuria exhibit a very different aspect (type VIb), as do soft matrices secondary mineralized by calcium and/or magnesium phosphates in the case of infection stones. Type VIb stones are made of loose concentric protein sheets often covered by crystals from metabolic, infectious or drug origin. The color and structure of the stone are influenced by the compounds deposited on the protein sheets (Fig. 12).
Pure protein stones or, more often, mixed protein and calcium oxalate stones, may be found in patients at end-stage renal disease (ESRD) and are treated for several months or years by maintenance dialysis. The stones are commonly of small size (1–3 mm) and present with a particular morphology (type VIc). They are made of unorganized light agglomerates of whewellite and proteins surrounded by a dark brown shell often composed of nearly pure proteins (Fig. 12). These stones result from moderate hyperoxalemia, defective reabsorption of small proteins in the proximal tubule and supplementation with calcium and vitamin D. It is a rare side effect of the long-term management of ESRD patients [73].
6.3 Drug-containing stones
A limited number of drugs may induce urinary stone formation [74]. Only molecules mainly excreted by the kidney, poorly soluble in urine and administered at high doses may crystallize in urine. Most of them belong to antiseptic, antibiotic or antiviral families. In recent years, the drugs most commonly responsible for stone formation are atazanavir, indinavir, sulphonamides (mainly N-acetylsulfadiazine) and calcium salt of ceftriaxone. Drugs may form pure stones by themselves or they may coat metabolic calculi. In other cases, the drug may be admixed with metabolic components such as calcium oxalate, calcium phosphate or uric acid. Fig. 13 illustrates some examples of drug-containing stones.
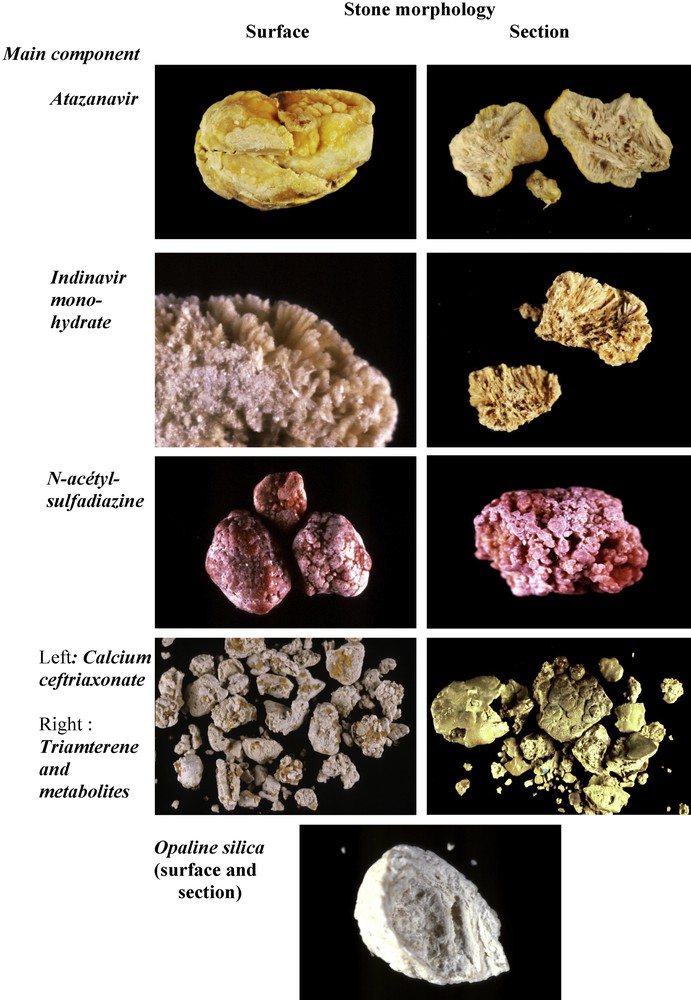
Morphological aspect of drug-containing stones.
6.4 Drug-induced stones
A special attention should be paid to drug-induced stones. Such calculi are made of common chemical phases such as calcium oxalate, calcium phosphate or urate. They result from the metabolic effects of some drugs in patients treated for a long time by these drugs for chronic diseases. Only few molecules seem to be involved in stone formation [74]. In some cases, the stone morphology may help to suspect an unusual cause of lithogenesis. As discussed in Section 3.4, an historical example was provided by the association of piridoxilate and pentaerytritol tetranitrate that was responsible for calcium oxalate stone formation in patients receiving Myocoril® for several months or years for cardiovascular diseases. Unusual stone morphology due to the presence of caoxite was one of the criteria used for the detection of such etiology [21,22]. However, in most cases, clinical inquiry is a major step for identifying long-term treatments that could be involved in stone formation in a given patient. Supplements of both vitamin D and calcium were reported to increase the risk of calcium stone formation [75–77] and dramatic epidemiological changes with time in the lithogenesis of calcium oxalate from carbapatite Randall's plaque raise questions regarding the role of vitamin D and calcium supplements [78,79]. Other drugs may induce stone formation. It was reported several decades ago that carbonic anhydrase inhibitors may induce calcium phosphate stone formation [80]. More than 10% of patients treated for a long term with acetazolamide or topiramate for glaucoma or epilepsy develop calcium phosphate stones [81,82]. Among other pharmacological classes able to induce urinary stones, it was reported that chronic laxative abuse may be responsible for ammonium urate stone formation [70,83]. Finally, from an epidemiological point of view, it was shown that urinary stones were more frequent in men who ingest one gram or more of ascorbic acid [84].
7 Clinical relevance of the morpho-constitutional analysis of urinary stones
The morphological examination of urinary stones in addition to chemical composition and identification of the crystalline phases of the chemical components defined by FTIR provide additional information of clinical relevance for etiologic diagnosis and choice of the best therapeutic strategy (Fig. 14). Obviously, stone analysis does not substitute for metabolic evaluation in stone formers. All patients should profit from a metabolic investigation based on the measurement of solutes in blood and 24 h-urine collection to look for inherited or acquired diseases and for risk factors for stone formation. Of note, in case no stone is available for a morpho-constitutional analysis, a crystalluria study may be very useful in addition to a metabolic evaluation.
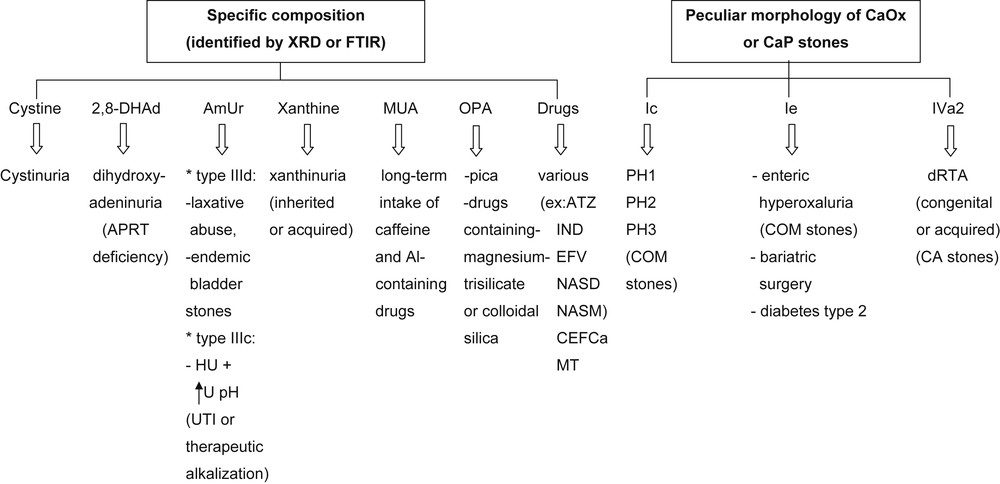
Contribution of morpho-constitutional analysis to the etiological diagnosis of rare stone diseases. Abbreviations: 2,8-DHAd = 2,8-dihydroxyadenine; AmUr = ammonium hydrogen urate; MUA = methyl-1 uric acid; OPA = opaline silica: PH = primary hyperoxaluria; dRTA = distal renal tubular acidosis; APRT = adenine phosphoribosyltransferase; COM = calcium oxalate monohydrate; Al = aluminum; CA = carbapatite; ATZ = atazanavir; IND = indinavir; EFV = efavirenz; NASD = N-acetylsulfadiazine; NASM = N-acetylsulfamethoxazole hydrochloride; CEFCa = ceftriaxone, calcium salt; MT = triamterene metabolites; HU = hyperuricosuria; U pH = urine pH; UTI = urinary tract infection.
7.1 The detection of hereditary stone diseases
One major interest is the rapid detection of severe hereditary diseases that, if treated late or improperly, may lead to a progressive impairment of renal function and end-stage renal failure. Such a dramatic evolution is usually due to late or erroneous diagnosis and is all the more unfortunate since it might be prevented or substantially delayed by an appropriate treatment and since these diseases are most often revealed by a stone episode. This aspect is to be especially emphasized with regards to two hereditary diseases of particularly high detrimental consequences:
- - primary hyperoxaluria type 1 is the most severe of all stone diseases because the permanent endogenous oxalate overproduction leads to massive hyperoxaluria, recurrent COM nephrolithiasis and nephrocalcinosis with a parenchymal deposit of COM crystals, decline in GFR, hyperoxalemia with a systemic oxalosis, and ultimately end-stage renal disease requiring a combined liver–kidney transplantation [85,86]. Discovery of type Ic calculi made of pure COM with an unexpected clear color instead of the usual dark color of common-type COM stones should immediately alert as to the probability of PH1 (or also PH2 and PH3), prompting an early laboratory evaluation to achieve a definitive diagnosis [4,86] and early institution of conservative medical treatment, which starts by testing the effect of pharmacological doses of pyridoxine, which diverts glyoxylate metabolism and may substantially reduce endogenous oxalate production [87,88].
- - dihydroxyadeninuria, due to mutations of the enzyme adenine phosphoribosyltransferase (APRT), results in both the formation of stones made of 2,8-DHA and infiltration of the renal parenchyma by 2,8-DHA crystals, leading to extensive interstitial fibrosis and ultimately to end-stage renal disease, requiring chronic dialysis and kidney transplantation [89–92]. The identification of stones made of 2,8-DHA by means of FTIR or XRD immediately orients the diagnosis that will be confirmed by the determination of the enzymatic activity of APRT [93]. Considering the small number of cases reported in various countries, it appears that APRT deficiency is an underestimated pathology [94], which is detrimental to the patients because an early instituted, life-long pharmacologic treatment with allopurinol or febuxostat totally prevents the overproduction of DHA and its clinical consequences. Of note, alkalization is totally ineffective to treat DHA stones, at variance with uric acid calculi, that may be misdiagnosed on the basis of radiolucency in the absence of a proper stone analysis. As shown in Fig. 15, DHA stones have peculiar morphologic characteristics, and thus may be differentiated from UA calculi on stereomicroscopic examination in patients presenting with radiolucent calculi [19]. The surface of the stones is often heterogeneous, embossed and locally rough. The color is beige to rust-brown. A particular aspect of DHA calculi is the local presence of small spherical deposits of pale rust-brown in color (black arrows in Fig. 15) visible at the stone surface and also in the cross-section. It is of prime importance for the affected patients that an early diagnosis might be performed on the basis of stone analysis or, if no stone is available, of microscopic examination of urine that contains numerous typical crystals of dihydroxyadenine [95].
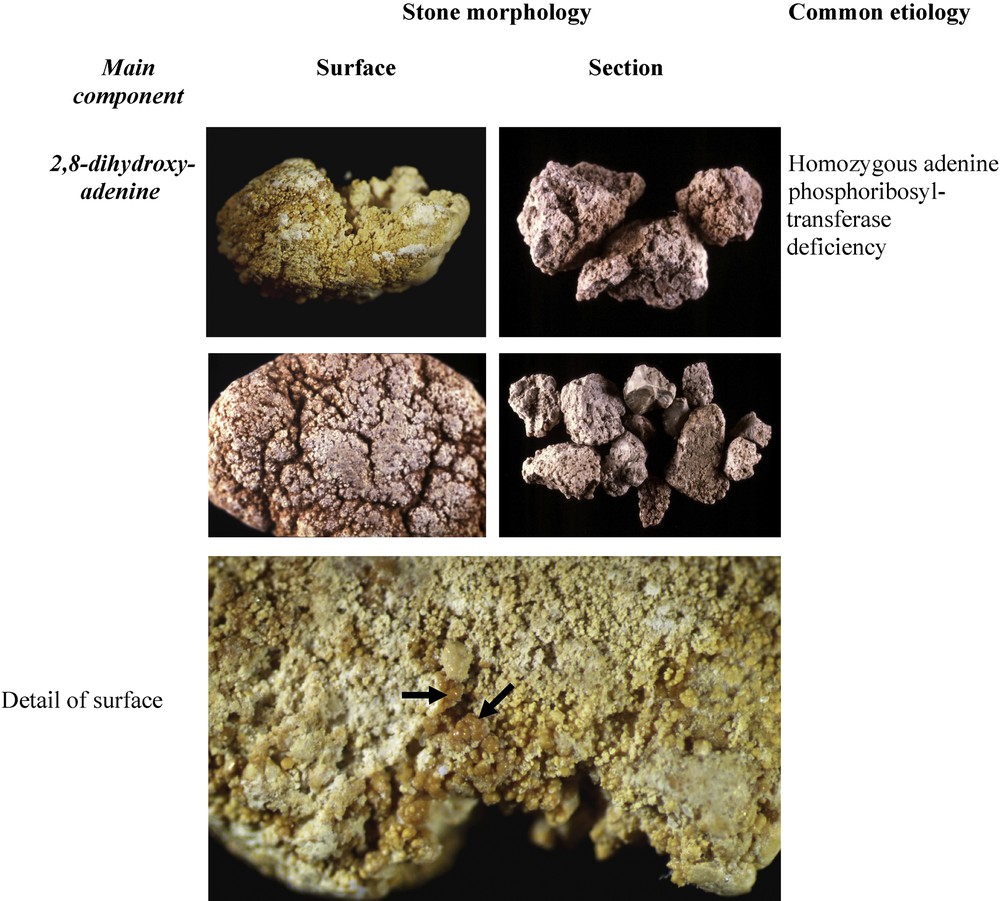
Morphological aspects of 2,8-dihydroxyadenine stones.
7.2 Orientation toward hypercalciuria or moderate hyperoxaluria in common CaOx stone-formers
Among common CaOx calculi, the predominance of COD type IIa suggests hypercalciuria as the main lithogenic factor, and type IIb is suggestive of hypercalciuria associated with moderate hyperoxaluria. The predominance of COM (type Ia) is most often associated with an increased urine oxalate concentration resulting from poor liquid intake and normal or moderately increased oxalate excretion, often of dietary origin. In addition, type IIa or IIb COD stones exhibiting at their surface dodecahedral crystals instead of the usual octahedral (bipyramidal) crystals are indicative of heavy hypercalciuria with urine Ca concentration often in excess of 10 mmol/l. Type IIa or IIb COD calculi showing at their surface very large octahedral crystals (commonly a length of crystal side ≥ 2.5 mm) were found in our experience in patients with hypercalciuria associated with additional lithogenic factors such as hyperoxaluria and/or hypocitraturia (Fig. 16).
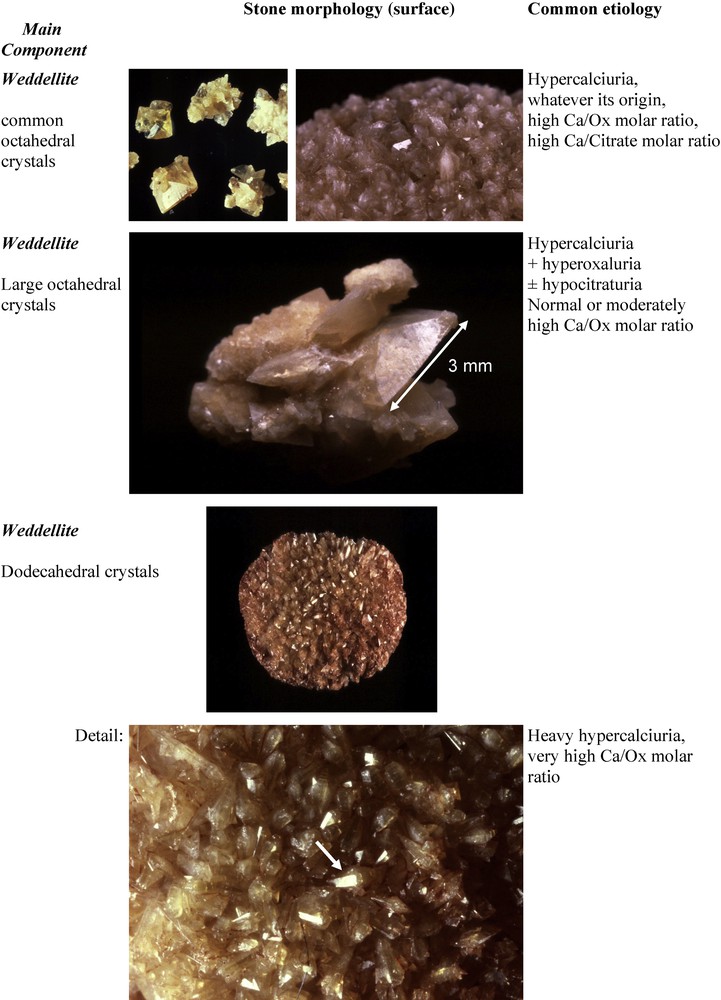
Type IIa stones: relation between octahedral or dodecahedral crystals of weddellite and etiology.
Spontaneously passed umbilicated type Ia calculi with Randall's plaque made of carbapatite surrounded by COM [7,79,96], either pure or admixed with COD (Figs. 4 and 6), suggest a pathogenic role for hypercalciuria in the origin of the stone [97,98]. Further changes in metabolic risk factors may induce the coverage of the stone by other crystalline phases such as uric acid in patients who develop insulin resistance, metabolic syndrome and type 2 diabetes (Fig. 9).
In patients with inflammatory bowel diseases such as Crohn's disease or pancreatic insufficiency, CaOx stones often form as a consequence of absorptive hyperoxaluria. However, in the early stages of such diseases, CaOx calculi are commonly composed of COM or, less frequently of COM and COD mixtures resembling common CaOx stones with a type Ia or type Ia+IIb morphology. At further stages of the disease, especially in the case of ileal resection, changes in stone morphology from type Ia to type Ie are progressively observed in recurrent stone patients [99].
7.3 Orientations given by the diverse types of CaP stones
In common CaP stones made predominantly of carbapatite type IVa1 or IVb, the presence of even small amounts of struvite, ACCP or whitlockite and a high carbonation rate (≥15%) of carbapatite are indicative of past or current UTI [40]. Conversely, absence of these compounds and a low rate of carbonation (<10%) are indicative of CaP stones of metabolic origin such as in primary hyperparathyroidism.
CaP stones of the IVa2 morphology are specific of distal renal tubular acidosis [29], either congenital (complete or incomplete) or acquired such as in patients with Sjögren syndrome [3] and in some patients with MSK [29].
7.4 Orientations given by the diverse types of uric acid or urate stones
Among uric acid stones, type IIIb calculi are frequently associated with conditions of insulin resistance such as obesity, metabolic syndrome and type 2 diabetes [100]. When occurring in overweight patients, they should prompt an evaluation of glucose homeostasis.
In contrast with uric acid stones, urate calculi result from hyperuricosuria and not from urine acidity. The nature of the cation associated with the urate anion is important to consider. Sodium hydrogen urate is associated with a high sodium concentration in the urine, whereas ammonium hydrogen urate is associated with a high urinary ammonium concentration, involving different origins: metabolism, acid-base status, or UTI with urease-producing microorganisms. Metabolism is the natural origin of ammonium production as the kidney excretes a major part of H+ ions produced by the metabolism in the form of ammonium ions. However, due to the poor solubility of ammonium urate in alkaline urine, patients presenting with hyperuricosuria may form ammonium hydrogen urate when treated with alkali to improve the solubility of uric acid. Ammonium urate stones resulting from alkalizing treatment exhibit a type IIIc morphology. The same type is encountered in the case of local ammonium production by urea-splitting bacteria. In patients suffering from chronic or intermittent diarrhea related to enteric infections (endemic urolithiasis) or to laxative abuse (in Western countries), the loss of alkali and electrolytes by the gut results in the overproduction of ammonium to reduce acidosis, defect of electrolytes in urines, low diuresis and high urate concentration, a combination of factors that favor the crystallization of ammonium urate in the form of type IIId stones. This morphology appears as a reliable marker of such an etiology [101].
7.5 The prediction of response of stones to ESWL
The identification of the crystalline phases of CaOx or CaP stones is of major interest for predicting the probability of fragmentation by ESWL, inasmuch as imaging techniques such as dual-energy X-ray absorption do not afford totally reliable information [102,103]. Indeed, common COM stones (type Ia) have a slightly higher attenuation coefficient (indicating a higher density on computerized tomography) than COD stones [103]. COD stones are easily fragmented whereas COM stones are often resistant to fragmentation, depending on their internal crystal organization [104]. Among CaP stones, type IVd calculi made of brushite have the highest density on computerized tomography and are especially resistant to ESWL. Cystine calculi, when modified by alkalization (type Vb, or ‘smooth’), are less easily fragmented than native ‘rough’ cystine stones (type Va) [105]. Most medical treatments may modify some characteristics of cystine crystals within stones [106], but usually fail to dissolve them.
In conclusion, the morpho-constitutional analysis of urinary stones which combines careful morphologic examination of the surface and section of stones with an analysis of their composition by means of FTIR or XRD considerably refines the etiologic diagnosis of kidney stone disease. If identification by these physical methods of specific components such as cystine, 2,8-DHA, xanthine, struvite, uric acid, ammonium hydrogen urate or drugs is by itself sufficient to identify the corresponding types of nephrolithiasis, the simple mention of calcium oxalate or calcium phosphate, as often only provided in laboratory reports, does not provide the full information that might be gained from a comprehensive analysis. Indeed, despite a same chemical composition, CaOx or CaP stones exhibit distinct morphologic characteristics according to their etio-pathogenic factors. In particular, the identification of a peculiar, morphologic aspect of COM calculus (Ic) orients towards the hypothesis of primary hyperoxaluria, whereas a peculiar morphology of carbapatite stones (IVa2) is specific of distal renal tubular acidosis, either congenital or associated with acquired diseases. Among common CaOx stones, distinction between COM and COD as the main component orients toward, respectively, hyperoxaluria (mainly of nutritional origin) or hypercalciuria (due to high protein or salt intake and genetic predisposition) as the preponderant lithogenic factor. An FTIR analysis of the composition of the core (or umbilication) of calculi indicates the initiating lithogenic process whereas the surrounding layers reflect factors acting in stone growth, that may differ from those involved in the core composition. Thus, the proposed morpho-constitutional method, which is simple, rapid and cost-effective, appears to be the most informative method of stone analysis.