1 Introduction
Due to their simplicity of synthesis and their recyclability, magnetic-nanoparticle-supported catalysts, especially iron oxide nanoparticles such as magnetite (Fe3O4) and maghemite (γ-Fe2O3) have been greatly utilized in organic reactions, including hydrogenation and reduction, oxidation, hydroformylation, C–C coupling, asymmetric, biocatalytic, olefin metathesis, and other catalytic reactions [1–4]. Different catalysts have been immobilized on magnetic nanoparticles (MNPs), including:
- • organic materials such as small molecules and surfactants, polymers, ionic liquids and biological molecules;
- • inorganic materials such as silica, metals and nonmetals, metal oxides and sulfides [5,6].
Because of simple experimental operations, mild reaction conditions, inexpensive, environmentally benign reagents and solvents and large-scale reaction, phase-transfer catalysis (PTC) is a very useful approach [7]. One practical limitation of the phase-transfer method, however, is that many of the catalysts used promote the formation of stable emulsions [8]. Immobilization of the phase-transfer catalyst on an insoluble magnetic support can provide a simple solution to this problem.
β-Azido alcohols are compounds of interest in organic synthesis as either precursors of vicinal amino alcohols or in the chemistry of carbohydrates, nucleosides, lactames, and oxazolines [9]. Generally, azidohydrins are prepared through ring opening reactions of epoxides by using different azides in suitable solvents. Even though the classical protocol uses sodium azide and ammonium chloride, the azidolysis reaction requires a long reaction time (12–48 h) and the azidohydrin is often accompanied by isomerization, epimerization, and rearrangement of products [10].
Although a few reagents and catalysts have been reported recently for the conversion of epoxides to β-azido alcohols, β-cyanohydrins, and β-acetoxy alcohols [11–18], disadvantages such as long reaction times, low yields of products, difficulty in the preparation and/or the storage of reagents or catalysts, tedious workup, and, in most cases, low regioselectivity, clearly identify a need for introducing new methods for such functional group transformations.
In continuation of our effort toward the synthesis of novel catalysts, and their application in organic synthesis [19–24], an Fe3O4@SiO2/bipyridinium nanocomposite (Fe3O4@SiO2/BNC) has been introduced as a novel heterogeneous phase-transfer catalyst for the one-pot preparation of β-azido alcohols, β-cyanohydrins, and β-acetoxy alcohols in aqueous medium (Scheme 1).
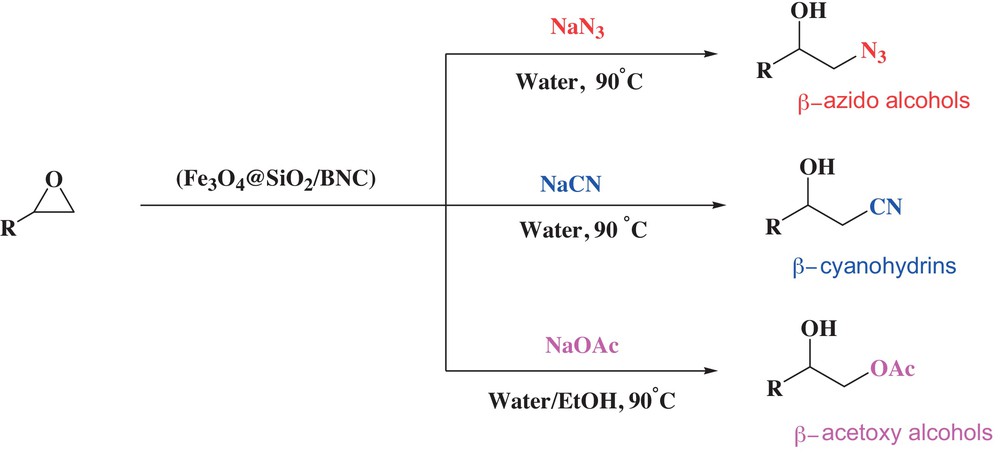
(Color online). One-pot preparation of β-azido alcohols, β-cyanohydrins, and β-acetoxy alcohols using Fe3O4@SiO2/BNC as a catalyst.
2 Experimental
2.1 General
All commercially available chemicals were purchased from Fluka and Merck companies and used without further purification. The products were characterized by their physical constant and comparison with authentic samples. Reaction monitoring was accomplished by TLC on silica gel polygram SILG/UV 254 plates. The IR spectra were recorded on a BOMEM MB-Series 1998 FT–IR spectrophotometer using KBr pellets for the samples and the catalyst in the range between 4000 and 400 cm−1. 1H and 13C NMR spectra were recorded in CDCl3 and DMSO-d6 on a Bruker Advanced DPX 400 MHz spectrometer using TMS as internal standard. The SEM analyses were carried out using a LEO 1455VP Scanning Electron Microscope, operating from 1 to 30 kV. Thermal stability of the supported catalyst was examined using a BÄHR, SPA 503 Thermo-gravimetric Analyzer (TGA) at a heating rate of 10 °C.min−1 over the temperature range from 40 to 950 °C.
2.2 General procedure for the preparation of nano-Fe3O4
Nano-Fe3O4 was prepared via an improved chemical coprecipitation method [23]. A 500-mL round-bottom flask was charged with FeCl2·4H2O (3.1736 g, 16 mmol) and FeCl3·6H2O (7.57 g, 28 mmol) and dissolved in 320 mL of deionized water. The mixed solution was stirred under nitrogen at 80 °C for 1 h, then 40 mL of NH3·H2O (25%) were injected into the reaction mixture rapidly, which was stirred under a nitrogen atmosphere for another 1 h and then cooled to room temperature. The precipitated particles were washed five times with hot water and separated by magnetic decantation. Finally, nano-Fe3O4 was dried under vacuum at 70 °C.
2.3 General procedure for the preparation of N-N-bis(triethoxysilylpropyl)-4,4-bipyridinium dichloride precursor, (TEOS)2 BiPy+22Cl−
In a 25-mL three-neck round-bottom flask, bipyridine (8 mmol, 1.25 g) was added to DMF (5 mL), and stirred to make a clear solution. To this solution, (3-chloropropyl)triethoxy silane (16 mmol, 3.18 g) was added dropwise, and the mixture was stirred at 90 °C for 72 h under an argon atmosphere. Afterwards, the white solid, (TEOS)2 BiPy+22Cl−, was filtered and washed with methanol. This solid was dried for 2 h in an oven at 90 °C [27].
2.4 General procedure for the preparation of Fe3O4@SiO2/BNC
In a 250-mL round-bottom flask, 2 g of Fe3O4 nanoparticles were dispersed in a solution of 40 mL of distilled water and 120 mL of ethanol via an ultrasonic bath for 15 min. Afterwards, 3 mL of ammonia (25%) were added. In the next step, (TEOS)2 BiPy+22Cl− (5 g) was added to the suspension. While stirring, 1.5 mL of TEOS in 40 mL of ethanol was added dropwise to the suspension. After 48 h of stirring at room temperature, the desired catalyst, Fe3O4@SiO2/BNC, was separated via an external magnet and was washed repeatedly with ethanol. Then the catalyst was dried at 60 °C for 2 h.
2.5 General procedure for the preparation of β-azido alcohols in the presence of Fe3O4@SiO2/BNC in water
A 25-mL round-bottom flask was charged with epoxide (1 mmol), sodium azide (3 mmol), Fe3O4@SiO2/bipyridine (0.03 g) and 5 mL of deionized water and was heated at 90 °C in an oil bath for a specific time (10–50 min). After completion of the reaction, which was indicated by TLC [TLC n-hexane/ethyl acetate (5:1)] (see Table 2), the catalyst was separated utilizing an external magnet. The product was extracted with diethyl ether (3 × 10 mL), the organic phase was concentrated and dried using CaCl2. After evaporation of the solvent under reduced pressure with a rotary evaporator, the β-azido alcohols were obtained with 88–95% yield.
Preparation of β-azido alcohols from corresponding epoxides and sodium azide in the presence of Fe3O4@SiO2/BNC in aqueous medium at 90 °C.
Entry | Epoxide | Product | Time (min) | Yield (%) |
1 | 15 | 95 | ||
2 | 50 | 95 | ||
3 | 10 | 91 | ||
4 | 10 | 92 | ||
5 | 10 | 89 | ||
6 | 20 | 90 | ||
7 | 20 | 88 | ||
8a | 30 | 90 |
a Due to low boiling point of 1,2-epoxy butane, the reaction was conducted at 60 °C.
2.6 General procedure for the preparation of β-cyanohydrins in the presence of Fe3O4@SiO2/BNC in water
A 25-mL round-bottom flask was charged with epoxide (1 mmol), sodium cyanide (3 mmol), Fe3O4@SiO2/BNC (0.05 g) and 5 mL of deionized water and was refluxed in an oil bath for a specific time (20–40 min). After completion of the reaction, which was indicated by TLC [TLC n-hexane/ethyl acetate (5:1)] (see Table 3), the catalyst was separated utilizing an external magnet. The product was extracted with diethyl ether (3 × 10 mL), the organic phase was concentrated and dried using CaCl2. After evaporation of the solvent under reduced pressure with a rotary evaporator, the β-cyanohydrins were obtained with 85–95% yield.
Preparation of β-cyano hydrins from corresponding epoxides and sodium cyanide in the presence of Fe3O4@SiO2/BNC in aqueous medium at 90 °C.
Entry | Epoxide | Product | Time (min) | Yield (%) |
1 | 40 | 94 | ||
2 | 20 | 95 | ||
3 | 25 | 92 | ||
4 | 25 | 87 | ||
5 | 25 | 95 | ||
6 | 30 | 91 | ||
7 | 20 | 85 | ||
8a | 40 | 90 |
a Due to low boiling point of 1,2-epoxy butane, the reaction was conducted at 60 °C.
2.7 General procedure for the preparation of β-acetoxy alcohols in the presence of Fe3O4@SiO2/BNC in water
A 25-mL round-bottom flask was charged with epoxide (1 mmol), sodium acetate (8 mmol), Fe3O4@SiO2/BNC (0.07 g) and 5 mL of aqueous ethanol (50:50); the mixture was refluxed in an oil bath for a specific time (25–90 min). After completion of the reaction, which was indicated by TLC [TLC n-hexane/ethyl acetate (5:1)] (as indicated in Table 4), the catalyst was separated utilizing an external magnet. The product was extracted with diethyl ether (3 × 10 mL), the organic phase was concentrated and dried using CaCl2. After evaporation of the solvent under reduced pressure with a rotary evaporator, the β-acetoxy alcohols were obtained with 78–92% yield.
Preparation of β-acetoxy alcohols from corresponding epoxides and sodium acetate in the presence of Fe3O4@SiO2/BNC in ethanol/water (50:50) under reflux conditions.
Entry | Epoxide | Product | Time (min) | Yield (%) |
1 | 90 | 92 | ||
2 | 35 | 90 | ||
3 | 30 | 87 | ||
4 | 30 | 85 | ||
5 | 30 | 82 | ||
6 | 50 | 78 | ||
7 | 25 | 88 | ||
8a | 45 | 79 |
a Due to low boiling point of 1,2-epoxy butane, the reaction was conducted at 60 °C.
3 Results and discussion
In the first step, Fe3O4 magnetic nanoparticles were prepared via the well-known coprecipitation method. Afterwards, N-N-bis(triethoxysilylpropyl)-4,4-bipyridinium dichloride, (TEOS)2 BiPy+22Cl, as a precursor was prepared by the reaction of bipyridine and (3-chloropropyl) triethoxy silane in DMF at 90 °C for 72 h (Scheme 2). In the next step, (TEOS)2 BiPy+22Cl− and TEOS were added to the suspension of magnetite nanoparticles in the presence of ammonia to prepare the silica shell containing bipyridinium units through a sol–gel method (Scheme 3).

Preparation of (TEOS)2 BiPy+22Cl−.
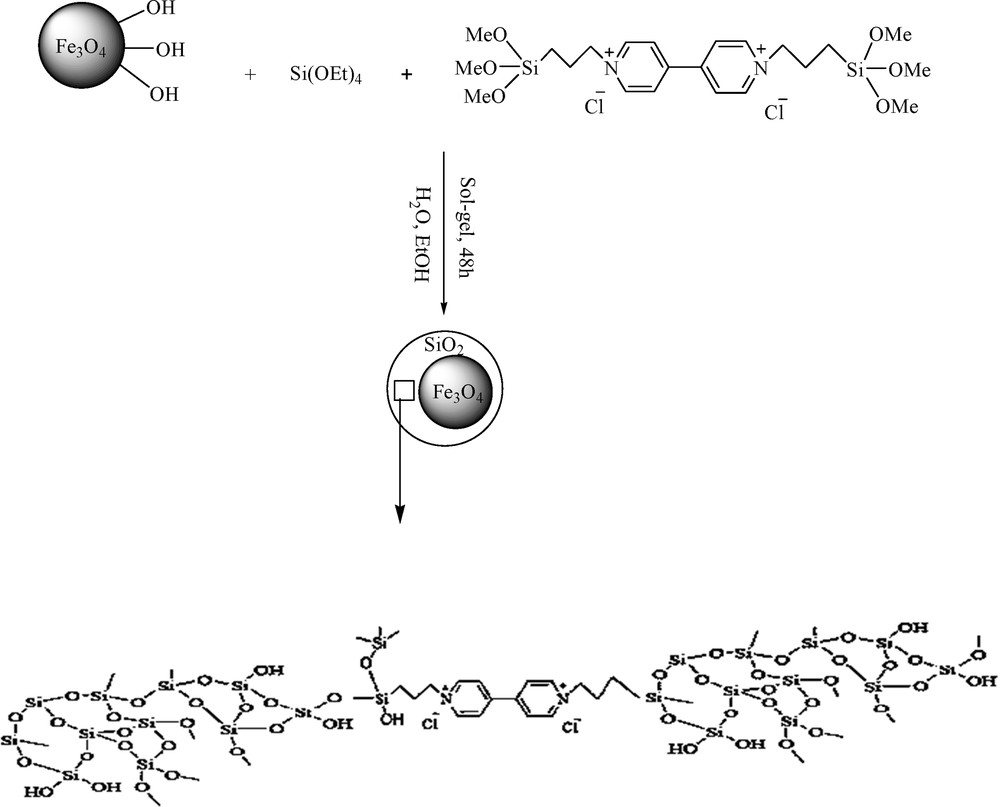
Preparation of Fe3O4@SiO2/BNC.
To study the structure of Fe3O4@SiO2/BNC, its characterization was accomplished with FT–IR, SEM, TGA, VSM and XRD techniques.
The FT–IR spectra of Fe3O4 nanoparticles, (TEOS)2BiPy+22Cl− and Fe3O4@SiO2/BNC were recorded. In the spectra of Fe3O4 nanoparticles and of Fe3O4@SiO2/BNC, the characteristic peak corresponding to the stretching of the Fe–O bond is evident. Peaks in the range from 990 to 1200 cm−1 in the spectra of (TEOS)2 BiPy+22Cl− and Fe3O4@SiO2/BNC is due to symmetrical and unsymmetrical stretching vibrations of the Si–O bond, which verifies the presence of silica shell around the magnetic nanoparticles. The hydroxyl groups on the surface of the silica shell and also the absorbed water show stretching vibration peaks of the O–H bond in the 3200–3600 cm−1 region. The stretching peaks due to the aromatic moiety are evident in the 1400–1600 cm−1 region. The bending vibration of water is seen at 1640 cm−1 (Fig. 1).
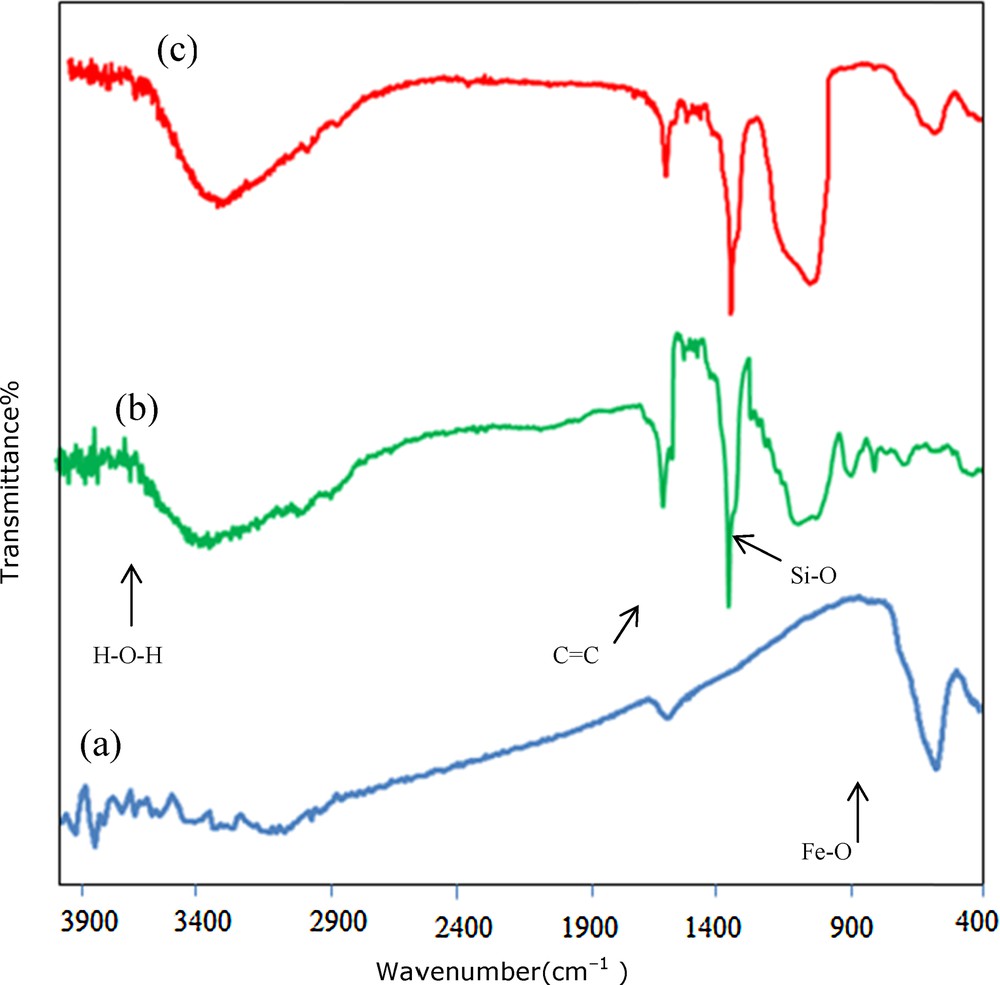
(Color online.) FTIR spectra of nano-Fe3O4 (a); (TEOS)2 BiPy+22Cl− (b); and Fe3O4@SiO2/BNC (c).
After successful verification of the structure by recording the FT–IR spectrum, it was decided to record the SEM image of the catalyst. According to the SEM image, although the particles have coagulated, the size of the nanocomposite particles is under 100 nm (Fig. 2).
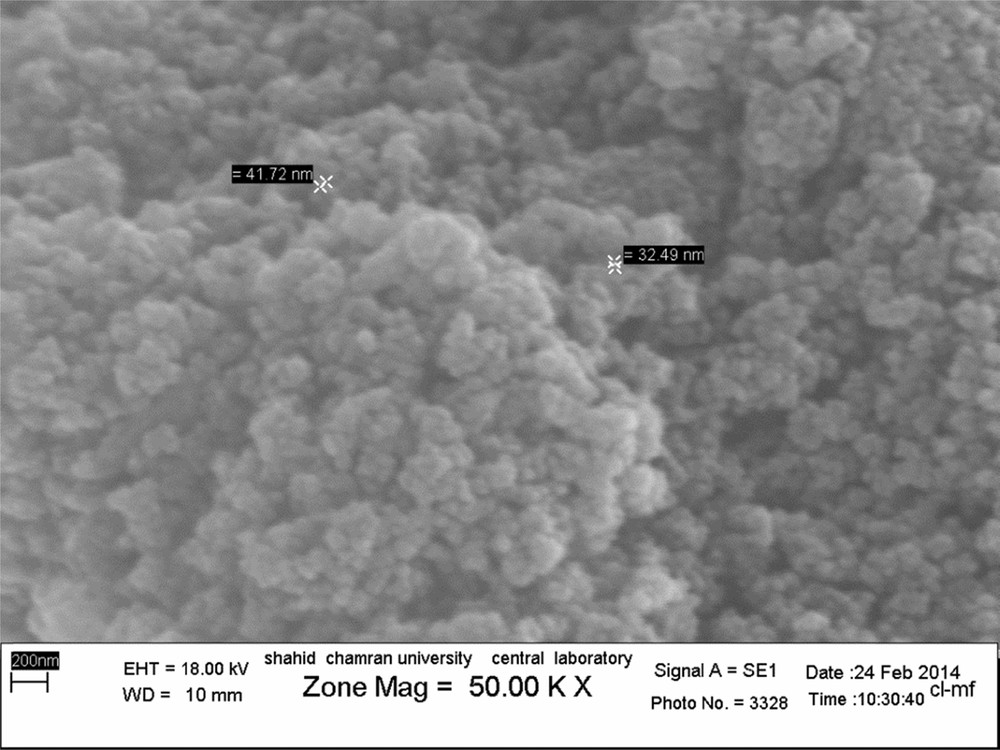
(Color online.) SEM image of Fe3O4@SiO2/BNC.
To evaluate the thermal stability of Fe3O4@SiO2/BNC, thermogravimetric analysis (TGA) was conducted. According to the TGA diagram, the grafted copolymer is stable up to 250 °C (Fig. 3).
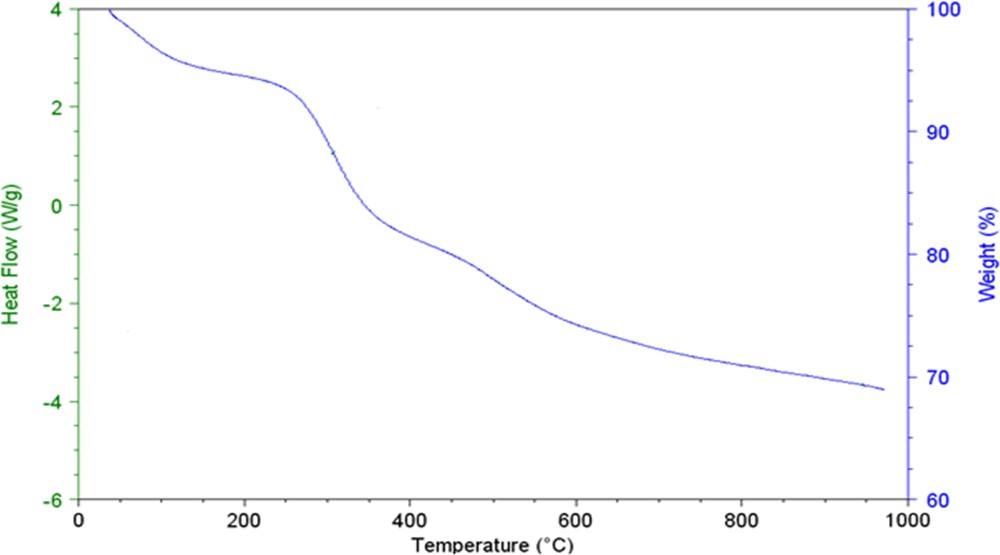
(Color online.) TGA diagram of Fe3O4@SiO2/BNC.
A mass loss of a ca. 6% is seen at 200 °C, which can be attributed to the elimination of water. After 250 °C, it seems that the organic part starts to decompose and a mass loss of about 20% is seen in the range from 250 to 600 °C. Further weight loss in the range between 650 and 800 °C can be attributed to the phase transition from Fe3O4 to FeO [28].
The X-ray diffraction pattern of Fe3O4@SiO2/BNC was recorded (Fig. 4). It can be seen that the positions and the intensity of the diffraction peaks are consistent with the standard pattern for JCPDS Card No. (19–629) for magnetite, with six peaks at 2θ = 30.5, 35.8, 43.4, 53.8, 57.5, and 63.1°.
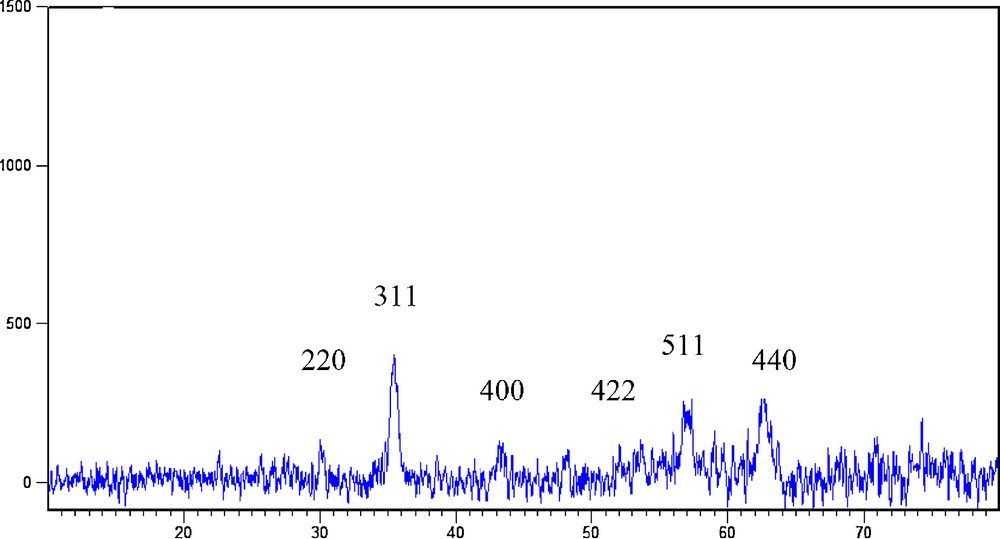
(Color online.) X-ray diffraction pattern of Fe3O4@SiO2/BNC.
In order to investigate the magnetic properties of Fe3O4@SiO2/BNC, a vibrating sample magnetometer (VSM) was used. The saturation magnetization of Fe3O4@SiO2/BNC was 33.8 emu/g (Fig. 5).
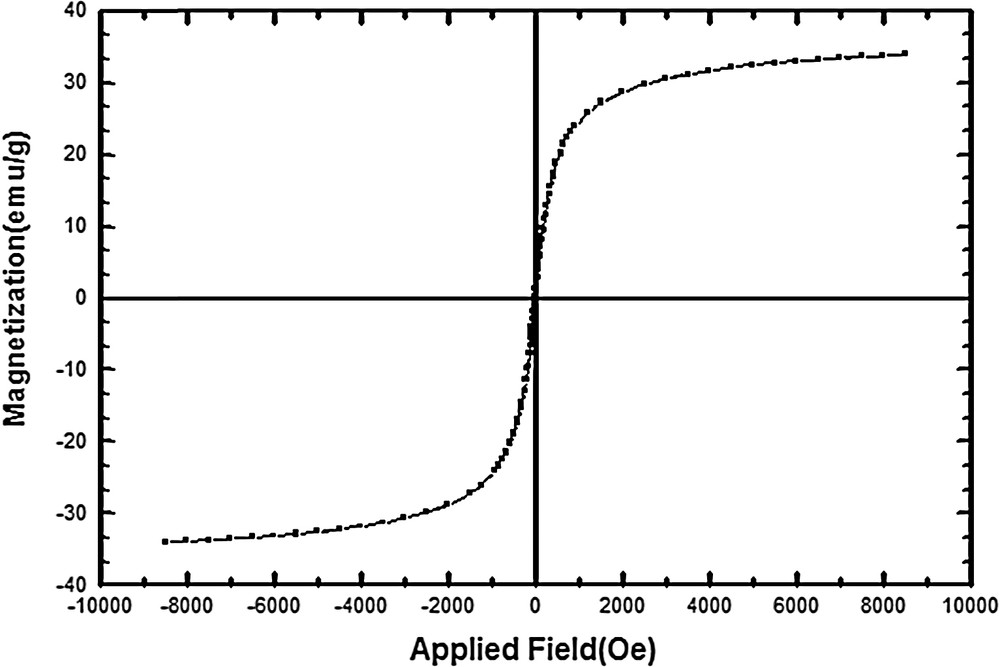
VSM curve of Fe3O4@SiO2/BNC.
After successful characterization of Fe3O4@SiO2/BNC using FT–IR, SEM, TGA, VSM and XRD techniques, it was decided to evaluate its catalytic activity as a phase-transfer catalyst in the ring opening of epoxides.
In the beginning, a model reaction of phenyl glycidyl ether (1 mmol) and sodium azide (2–3 mmol) in the presence of Fe3O4@SiO2/BNC in 5 mL of deionized water was conducted under different conditions. According to TLC, the optimized amount of reactants and of the catalyst was found: 3 mmol of sodium azide and 0.03 g of the catalyst complete the reaction at 90 °C in a minimum time (Table 1).
Optimization of reaction conditions for the preparation of β-azido alcohol from phenyl glycidyl ether, in the presence of Fe3O4@SiO2/BNC in aqueous medium.
Entry | NaN3 (mmol) | Nanocomposite (g) | Temperature (°C) | Time (min) |
1 | 2 | 0.07 | 90 | 60 |
2 | 2 | 0.1 | 90 | 50 |
3 | 3 | 0.03 | 90 | 50 |
4 | 3 | 0.05 | 90 | 50 |
5 | 3 | 0.07 | 90 | 50 |
6 | 3 | – | 90 | 360 |
7 | 3 | 0.07 | 60 | 240 |
8 | 3 | 0.07 | r.t | 300 |
In the FT–IR spectrum, the stretching vibration of azide group is obvious at 2106 cm−1 (Fig. 6).
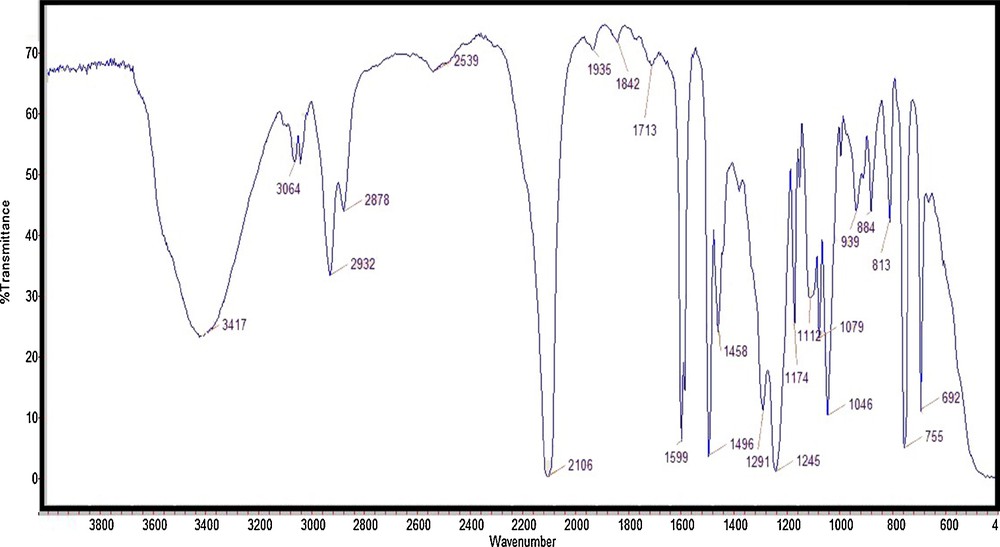
(Color online.) FT–IR spectrum of 2-hydroxy-3-phenoxy propyl azide.
In the next step, with optimum conditions in hand (1 mmol epoxide: 3 mmol azide: 0.03 g Fe3O4@SiO2/BNC: 5 mL of deionized water at 90 °C), the generality and synthetic scope of this coupling protocol were demonstrated by synthesizing a series of β-azido alcohols (Table 2) in 10 to 50 min with 88–95% yield.
A glimpse to Table 2 makes clearly evident the generality of this methodology. Different functional groups, including ether, ester and double bond, are easily tolerated in the reaction conditions. The results clearly indicate the high regioselectivity of epoxide ring-opening by the azide anion. Steric and electronic effects direct regioselectivity; for example, in styrene oxide, the phenyl group acts as an electron-donating group via resonance, which in turn stabilizes the carbocation. As a result, the azide attack is directed by electronic effects and the reaction is thought to be pseudo SN1. In the other cases, due to steric hindrance of bulky groups, the unsubstituted carbon is more accessible to the azide attack and the reaction proceeds with a SN2 mechanism.
After the success of Fe3O4@SiO2/BNC in the preparation of β-azido alcohols, we decided to explore the catalytic activity of this catalyst in the preparation of β-cyanohydrins. Subsequently, the ring opening of epoxides (1 mmol) was conducted with sodium cyanide (3 mmol) in the presence of Fe3O4@SiO2/BNC (0.05 g) as the magnetic PTC at 90 °C in aqueous medium. In the FT–IR spectrum, the stretching vibration of the cyanide group is obvious at 2253 cm−1 (Fig. 7).
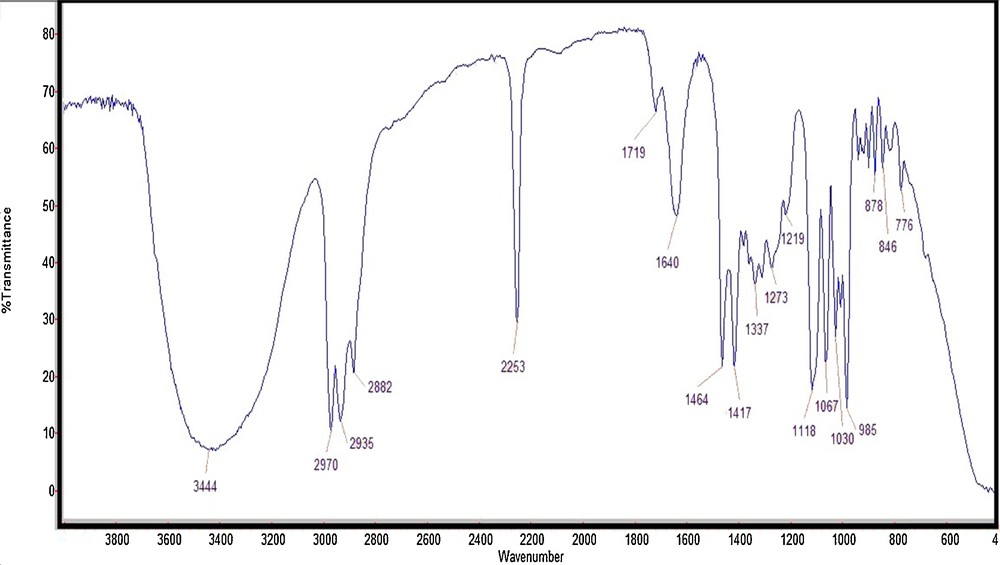
FT–IR spectrum of 2-hydroxy-pentanenitrile.
All epoxides reacted almost equally well to afford the corresponding β-cyano hydrins in high to excellent yields (Table 3).
With the same method, we decided to explore the catalytic activity of this catalyst in the preparation of β-acetoxy alcohols. Thus, the ring opening of epoxides (1 mmol) was conducted with sodium acetate (8 mmol) in the presence of Fe3O4@SiO2/BNC (0.07 g) as the magnetic PTC in a 50:50 mixture of ethanol/water under reflux conditions. In the FT–IR spectrum, the stretching vibration of carbonyl group is obvious at 1719 cm−1 (Fig. 8).
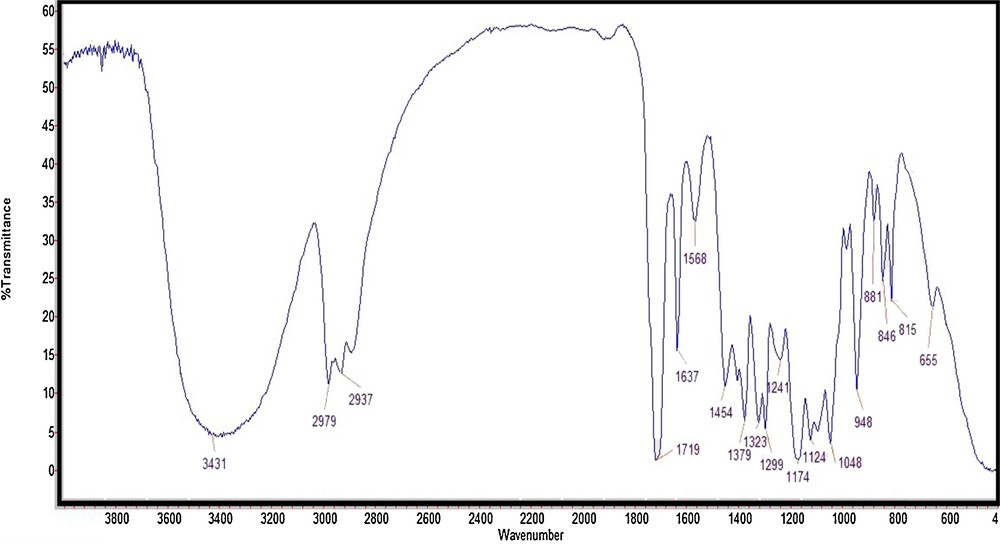
FT–IR spectrum of 3-acetoxy-2-hydroxy-propylmethacrylate.
All epoxides reacted almost equally well to afford the corresponding β-acetoxy alcohols in high to excellent yields (Table 4).
Due to the importance of green chemistry and catalyst recycling, the recyclability of Fe3O4@SiO2/BNC was assessed in the reaction of phenyl glycidyl ether with the azide anion. The result is shown in Fig. 9. The activity of the nanocomposite is slightly decreased after 4 times of reuse.

(Color online.) Reusability of the nanocomposite in the reaction of phenyl glycidyl ether with the azide anion.
To demonstrate the superiority of Fe3O4@SiO2/BNC over the reported catalysts, the azidolysis reaction of styrene oxide was considered as a representative example (Table 5). In all of these cases (except for entry 1, 2), a low yield of the desired product was obtained. It is also noteworthy to mention that all of the reported procedures required long reaction times (except for entries 4, 7). These results clearly demonstrate that Fe3O4@SiO2/BNC is an equally or more efficient catalyst for this reaction.
Comparison of azidolysis of styrene oxide with other reported methods in the literature.
Entry | Catalyst | Solvent | Temperature (°C) | Time (h) | Yield (%) | Ref |
1 | LiClO4 | CH3CN | 80 | 5 | 92 | [25] |
2 | NH4Cl | MeOH–H2O | 70 | 5 | 90 | [25] |
3 | (TBA)4PFe11MO39·3H2O | CH3CN–H2O | 80 | 4.5 | 85 | [26] |
4 | Dowex–PEG | H2O | 100 | 0.5 | 85 | [19] |
5 | PEG300 | PEG300 | 60 | 1.5 | 85 | [20] |
6 | SiO2–PEG | H2O | 100 | 1 | 80 | [23] |
7 | MPTCa | H2O | 90 | 0.5 | 85 | [24] |
8 | Network polymer | H2O | 80 | 1.5 | 85 | [22] |
9 | Fe3O4@SiO2/BNC | H2O | 90 | 0.25 | 95 | This work |
a Multi-site phase-transfer catalyst.
4 Conclusion
In conclusion, a novel Fe3O4@SiO2/BNC, has been synthesized and its application as a magnetic and recyclable phase-transfer catalyst in the preparation of β-azidoalcohols, β-cyanohydrins, and β-acetoxy alcohols was investigated. The catalyst was characterized with FT–IR, SEM, XRD, VSM and TGA. According to the experimental results, the regioselective ring opening reaction of epoxides, in the presence of azide, cyano and acetate anions in aqueous medium, leads to the corresponding products in a short reaction time and with high yields. This methodology offers several advantages, including an easy work-up procedure, excellent regioselectivity, high yields, short reaction times, recyclability of the catalyst, easy separation of the catalyst through an external magnet; it is also an eco-friendly procedure.
Acknowledgments
We are grateful to the Research Council of Shahid Chamran University for financial support.