1 Introduction
Nitrogen heterocycles play important roles in biology. They have a significant place among natural products and synthetic compounds because of their exclusive properties as traditional key elements of numerous drugs [1]. Pyridine derivatives are an important class of heterocyclic organic compounds that exist in many active pharmaceuticals and functional materials [2]. Among them, 2-amino-3-cyanopyridine derivatives are known to have multiple biological activities, such as anti-inflammatory, analgesic and antipyretic properties [3]. They are known as IKK-b inhibitors [4], adenosine receptor antagonists, potent inhibitor of HIV-1 integrase [5], and are also used for the synthesis of antiexcitotoxic agents [6].
The preparation of 2-amino-3-cyanopyridine derivatives has been reported in the literature via a reaction of four components under reflux condition [5,7], in conventional heating mode [8,9], or in ionic liquids [10], which usually requires harsh reaction conditions, long reaction times, expensive transition metal catalyst or operational complexity.
Magnetic nanoparticles (MNPs) demonstrate high activity and selectivity (similar to a homogeneous catalyst) and can be easily separated and recovered. Magnetically supported catalysts can be recovered with an external magnet owing to the paramagnetic character of the support, and the catalysts can be reused in another cycle. To consider the iron oxide nanoparticles, the iron atoms on the surface act as Lewis acids and coordinate with molecules that donate lone pair electrons [11]. Forming MNPs in polymer media is an acceptable way for applying them due to their ease of processing, solubility, lesser toxicity, and also because of the possibility of controlling the growth of the resulting NPs [12]. The most common organic polymer that is biocompatible and environmental friendly is cellulose. It also has characteristic properties such as hydrophilicity, chirality, degradability, and broad chemical variability initiated by the high donor reactivity of the OH groups, which makes it an excellent candidate as a catalyst support [13,14].
In recent decades, multicomponent reactions (MCRs) have attracted organic chemists because of some remarkable advantages over conventional bimolecular reactions owing to their convergence, atom-economy, operational simplicity, structural diversity and shortness of the synthetic pathway. MCRs have recently gained a new dimension in the field of designing methods to produce elaborate libraries of biologically active compounds and new molecular frameworks for potential drugs with diverse pharmacological activities [15].
In continuation of our previous works on the preparation and application of nanocatalysts in MCRs [16], herein, we report a simple, efficient and one-pot four-component protocol for the synthesis of highly substituted pyridines 5 or 6 via the reaction of various aromatic aldehydes 1, malononitrile 2, cyclic ketones 3 and ammonium acetate 4 in the presence of a catalytic amount of cellulose-based magnetic composite nanocatalyst (Fe3O4/cellulose) at room temperature in ethanol (Scheme 1).

Synthesis of highly substituted pyridines.
To the best of our knowledge, not only is there any report of obtaining the product at room temperature, but also, this is the first report of application of a cellulose-based magnetic nanocomposite as a catalyst for the synthesis of highly substituted pyridines.
2 Results and discussion
The in-situ preparation of cellulose nanocomposite containing Fe3O4 nanoparticles was described in our previous work [16a]. Cellulose films were immersed into FeCl3 solution, Fe3+ could be readily impregnated into the cellulose films through the pores. The incorporated Fe3+ ions could be bound to cellulose macromolecules via electrostatic interaction, because the electron-rich oxygen atoms of the polar hydroxyl of cellulose are expected to interact with electropositive transition metal cations.
The particle size was studied by scanning electron microscopy (SEM). As can be seen in Fig. 1, the morphology of the nanocomposite displayed a homogeneous structure. So, the iron ions were effectively diffused and adsorbed into the hydroxyl groups of cellulose by electrostatic interaction.
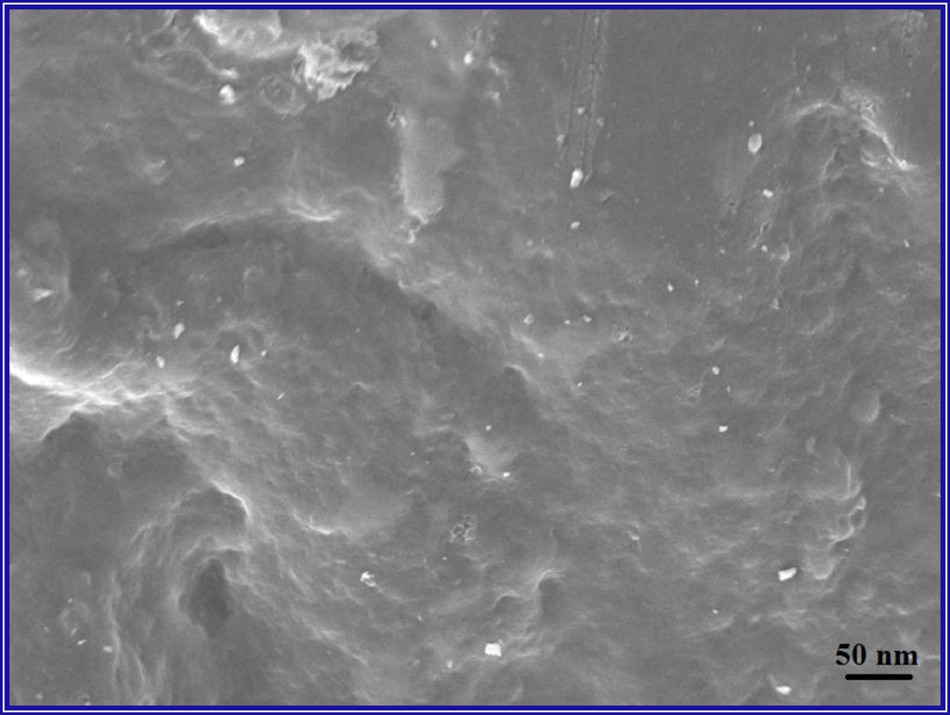
SEM image of our Fe3O4/cellulose nanocomposite.
In Fig. 2, the energy dispersive X-ray spectroscopy (EDS) pattern, obtained from SEM, indicates that there were only C, O and Fe elements in the composite film, suggesting that the iron oxide has been composed in the cellulose films to form a suitable nanostructure catalyst.
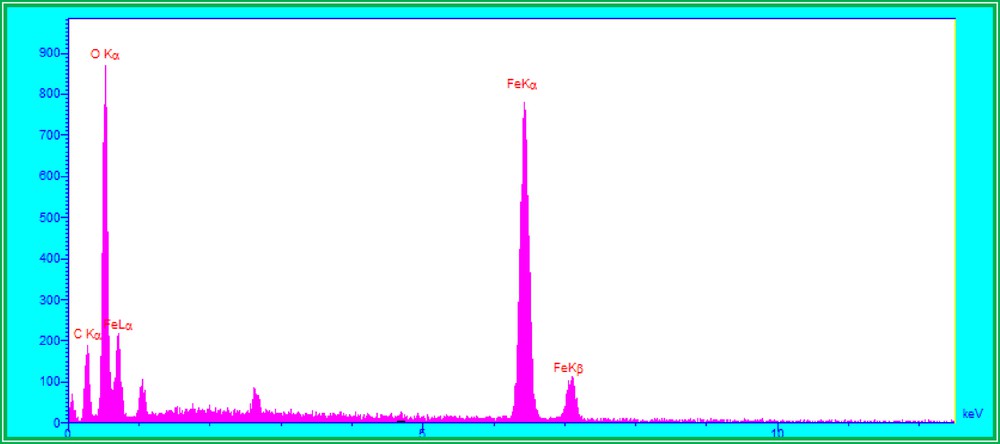
EDS pattern of our Fe3O4/cellulose nanocomposite.
Then, we have prepared highly substituted pyridines via the reaction of aldehydes, cyclic ketones, malononitrile and ammonium acetate in the presence of catalytic amounts of the magnetic nanocomposite in ethanol at room temperature. First, to optimize the reaction conditions, a model experiment was carried out by starting from 4-methylbenzaldehyde (1 mmol), cyclohexanone (1 mmol), malononitrile (1 mmol) and ammonium acetate (1.5 mmol) at room temperature (Table 1). To clarify the need for the Fe3O4/cellulose nanocatalyst, an experiment was conducted in the absence of Fe3O4/cellulose and also in the presence of cellulose and Fe3O4 alone (entries 1–3, respectively). According to our results, the best yield was obtained in the presence of Fe3O4/cellulose (1 mg). As is evident from Table 1, by increasing the amount of nanocomposite catalyst, the yield of the reaction was not improved and also by decreasing it to 0.5 mg, the desired product was obtained in lower yield (70%). In addition, various solvents such as CH3CN, DMF, CHCl3 and EtOH were used to find the optimum reaction media. It was found that the best results were obtained in EtOH as a green solvent.
Optimization of the reaction conditions.
Entry | Catalyst | Amount (mg) | Solvent | Time (h) | Yield (%) |
– | – | EtOH | 24 | 32 | |
1 | Cellulose | 2 | EtOH | 6 | Trace |
2 | Fe3O4 | 2 | EtOH | 6 | 35 |
3 | Fe3O4/cellulose | 2 | EtOH | 3 | 80 |
4 | Fe3O4/cellulose | 8 | EtOH | 3 | 70 |
5 | Fe3O4/cellulose | 5 | EtOH | 3 | 80 |
6 | Fe3O4/cellulose | 1 | EtOH | 3 | 80 |
7 | Fe3O4/cellulose | 0.5 | EtOH | 3 | 70 |
8 | Fe3O4/cellulose | 5 | CH3CN | 6 | No reaction |
9 | Fe3O4/cellulose | 5 | DMF | 6 | No reaction |
10 | Fe3O4/cellulose | 5 | CHCl3 | 6 | No reaction |
In the previous reports, cyclic ketones, such as cyclohexanone and cycloheptanone, are rarely used as starting material to prepare polysubstituted pyridines, probably because of their low activity. As indicated from Table 2, we obtained the same products under better conditions in comparison with literature methods.
Comparison of the nano-Fe3O4/cellulose with other literature reports for 5b and 6b.
Entry | R | Product | Catalyst | Solvent | Temp. | Time (h) | Yield (%) | Ref. |
1 | 4-OMe | 5b | [bmim]OH | [bmim]OH | 80 °C | 4 | 88 | [10] |
2 | 4-OMe | 5b | – | Benzene | Reflux | 4 | 46 | [7] |
3 | 4-OMe | 5b | Fe3O4/cellulose | Ethanol | r.t. | 3 | 98 | Present work |
4 | 4-OMe | 6b | – | Benzene | Reflux | 4 | 68 | [7] |
5 | 4-OMe | 6b | Au/MgO | Ethanol | 70 | 2.5 | 90 | [9] |
6 | 4-OMe | 6b | Fe3O4/cellulose | Ethanol | r.t. | 3 | 90 | Present work |
After optimizing the reaction conditions, some other substituted pyridine derivatives were synthesized by using various aldehydes and ketones. The products 5f and 6e were characterized by IR, 1H NMR and 13C NMR spectral analysis. To our knowledge, there is not any report of physical properties and spectral data of the two above-mentioned products. The results are summarized in Table 3.
Synthesis of highly substituted pyridines via 4-CR in the presence of a cellulose-based magnetic nanocatalyst in EtOH at room temperature.
Entry | RCHO | Product | Time (h) | Yielda (%) | Mp (°C) | |
Obsd | Lit. | |||||
1 | 4 | 93 | 235–237 | 234–235 [7] | ||
2 | 3 | 98 | 232–234 | 232–233 [17] | ||
3 | 3 | 80 | 248–249 | 248–249 [10] | ||
4 | 5 | 75 | 256–258 | 255–256 [10] | ||
5 | 5 | 85 | 256–258 | 258–259 [17] | ||
6 | 4 | 87 | 245–246 | Present work | ||
7 | 1a | 4 | 92 | 229–230 | 227–228 [9] | |
8 | 1b | 3 | 90 | 225–227 | 226–227 [7] | |
9 | 1c | 3 | 80 | 236–237 | 232–233 [7] | |
10 | 1d | 5 | 70 | 233 | 236–237 [9] | |
11 | 1f | 4 | 85 | 245–247 | Present work |
a Isolated yields.
To demonstrate the recyclability of the present composite nanocatalyst, it was recovered and reused several times in the model reaction. After completion of the reaction, the nanocatalyst was simply removed by using an external magnet, washed with EtOH and reused in the subsequent fresh reactions. As shown in Fig. 3, there is no significant loss of catalytic activity after five times.

Recyclability of the nanocatalyst.
3 Conclusions
In summary, a facile, one-pot and four-component protocol for the synthesis of highly substituted pyridines has been developed by using various aromatic and heteroaromatic aldehydes, cyclic ketones, malononitrile and ammonium acetate in the presence of a catalytic amount of Fe3O4/cellulose nanocatalyst at room temperature. This method includes some important advantages such as the use of an efficient, inexpensive, eco-friendly and magnetically recyclable composite nanocatalyst and of EtOH as a green reaction media, room-temperature operating conditions, short reaction times, and an easy work-up procedure. In addition, to best of our knowledge, this is the first report of synthesizing polysubstituted pyridines at room temperature.
4 Experimental
4.1 General
All solvents, chemicals and reagents were purchased from Merck, Fluka and Aldrich chemical companies. Melting points were measured on an Electrothermal 9100 apparatus and are uncorrected. All reactions and the purity of the products were monitored by thin-layer chromatography (TLC) using aluminum plates coated with silica gel F254 plates (Merck) using ethyl acetate and n-hexane as eluents. The spots were detected either under UV light or by placing them in an iodine chamber. IR spectra were recorded on a Shimadzu IR-470 spectrometer. The 1H and 13C NMR spectra were recorded on a Bruker NMR-500 MHz spectrometer. SEM images were obtained on a Seron AIS 2100. EDX spectra were recorded on Numerix DXP-X10P.
4.2 Preparation of Fe3O4/cellulose nanocomposite
Cellulose was dissolved directly in the NaOH aqueous solution which was precooled to −8 °C to prepare 4 wt% cellulose solution. The resulting solution was centrifuged at 2000 rpm for 30 min for degasification. The cellulose solution was cast onto a glass plate to give a thickness of 0.5 mm and was then immersed in a 2000-mL H2SO4 (5 wt%) bath for 5 min for the coagulation and the regeneration of the cellulose films. The resulting films were washed with running water and subsequently with distilled water. The wet films obtained were immersed in a 500-mL mixture of aqueous FeCl3 for 24 h; we then washed out the iron ions adsorbed on the surface of the film with distilled water. Then, the films were immersed in 500 mL of aqueous NaOH (4 mol/L) for 20 min and were then rinsed with distilled water for several times.
4.3 Synthesis of polysubstituted pyridines 5a–f and 6a–e
First, 1 mmol of malononitrile, 1 mmol of ketone and 1.5 mmol of ammonium acetate were added to 3 mL EtOH solution of aromatic aldehyde (1 mmol) in the presence of 1 mg of the nanocatalyst. The obtained solution was stirred for appropriate times at room temperature (Table 3). The completion of the reaction was monitored by TLC and after that the nanocatalyst was removed simply by using an external magnet. The obtained precipitation was filtered and washed with ethanol.
4.4 Spectral data for the products
4.4.1 2-Amino-4-(2-thienyl)-5,6,7,8-tetrahydroquinoline-3-carbonitrile 5f
Yellow solid: mp 245–246; IR (KBr, cm−1): 3423, 3307, 3163, 2935, 2208, 1641; 1H NMR (500 MHz, CDCl3): δ: 1.69–1.82 (4H, m, CH2), 2.51 (2H, br s, CH2), 2.80 (2H, br s, CH2), 5.05 (2H, s, NH2), 7.12–7.25 (3H, m, ArH); 13C NMR (125 MHz, DMSO-d6): δ: 22.9, 23.3, 26.9, 33.7, 89.6, 117.4, 120.3, 128.4, 129.0, 129.6, 136.2, 147.4, 158.9, 162.1.
4.4.2 2-Amino-4-(2-thienyl)-6,7,8,9-tetrahydro-5H-cyclohepta[b]pyridine-3-carbonitrile 6e
White solid: mp 245–247; IR (KBr, cm−1): 3417, 3317, 3188, 2923, 2208, 1643; 1H NMR (500 MHz, CDCl3): δ: 1.53–1.56 (2H, quintet, J = 5.0 Hz, CH2), 1.69–1.72 (2H, quintet, J = 5.0 Hz, CH2), 1.79–1.84 (2H, quintet, J = 5.0 Hz, CH2), 2.58–2.61 (2H, m, CH2), 2.91–2.94 (2H, m, CH2), 5.06 (2H, s, NH2), 7.05 (1H, d, J = 5.0 Hz, ArH), 7.13 (1H, dd, J = 5.0 and 4.0 Hz, ArH), 7.46 (1H, d, J = 5.0 Hz, ArH); 13C NMR (125 MHz, DMSO-d6): δ: 26.7, 28.7, 29.3, 32.1, 39.7, 88.6, 117.5, 126.3, 128.4, 128.9, 129.3, 137.2, 145.9, 159.0, 168.5.
Acknowledgements
The authors gratefully acknowledge the partial support from the Research Council of the Iran University of Science and Technology.
Appendix A Supplementary data
Electronic Supplementary Information includes 1H NMR, 13C NMR copies of the products 5f and 6e and MOL files.